Abstract
Prostate specific antigen (PSA) has transformed the diagnosis and treatment of prostate cancer by enabling early detection at global scale. Due to expression in both benign and malignant cells, PSA-based prostate cancer screening using single cut-points yields imperfect diagnostic performance and has led to the detection and over-treatment of low-grade prostate cancer. Additional challenges in the interpretation of PSA include substantial inter and intrapersonal variation, differences with age and prostate volume, and selection of standardized PSA value cutoffs for clinical application. In response, refinements to PSA including risk and age-based thresholds, age and genetic adjustments, PSA density, percentage free PSA, and PSA velocity have been proposed and extensively studied. In this review, we focus on the clinical role of PSA as a screening biomarker with a particular emphasis on its test characteristics, clinically actionable thresholds, and strategies to refine its clinical interpretation.
1
Introduction
The widespread use of prostate-specific antigen (PSA) has shaped the diagnosis, treatment, and outcome of prostate cancer. From a global perspective, PSA is among the most impactful cancer biomarkers that have emerged. As a low-cost, widely available, and easy to interpret clinical tool, PSA has allowed prostate cancer screening to be conducted across the world. By conservative estimates, PSA has averted or altered the natural history of prostate cancer in millions of individuals. Prior to the discovery and wide availability of PSA, prostate cancer was frequently diagnosed at locally advanced or metastatic stages. For example, the 5-year survival among those diagnosed with prostate cancer in the mid-1970s and 1980s was 69.3% and 76.3% respectively [ ]. Due to both earlier stage detection and the availability of effective treatment, modern 5-year survival estimates are 97.1% overall and nearly 100% for those with localized disease in 2013 to 2019 [ , ]. Since the introduction of PSA testing in the 1990s, there has been a substantial (45%–70%) reduction in prostate cancer mortality, much of which has been attributed to early detection enabled by PSA testing [ ]. Furthermore, diagnostic strategies for the considerable number of patients found to have abnormal PSA screening tests have evolved, with shifts from sextant biopsy towards image-guided and risk-adapted approaches. Detection of prostate cancer at an earlier stage has allowed for a flourishing of local treatments including refinements of radical prostatectomy, radiation, as well as ablative therapies. As a result, PSA has allowed for a transition from radical or palliative treatments for prostate cancer to an array of interventions tailored to disease-specific risk and functional preservation.
Operationalizing PSA screening results as “normal” vs. “abnormal” has remained an enduring challenge and continues to be the subject of active debate today. With early recognition that PSA is expressed by both benign and malignant prostate cells, some degree of controversy has surrounded the interpretation of PSA values since its earliest application as a prostate cancer biomarker given the challenges in distinguishing between these entities. Indeed, these concerns have been prescient as integration of PSA has also catalyzed a wave of over-treatment of incident prostate cancers, including those lacking the biologic capacity or sufficient time horizon for metastatic spread [ ].
The controversy surrounding PSA screening is reflected in the variation among global clinical guidelines concerning PSA utilization. The current National Comprehensive Cancer Network (NCCN) and American Urological Association (AUA) guidelines recommend PSA testing beginning at age 45 in average-risk patients and at age 40 in high-risk patients (African American individuals, concerning germline mutations, or family history) after counseling on the risks and benefits of screening [ , ]. This is in contrast to current European Assocation of Urology (EAU) guidelines which recommend screening in men with elevated risk of prostate cancer only (age ≥50, age ≥45 with family history and/or African descent, or age ≥40 with BRCA2 mutations), reflecting the competing risks and benefits of population-based PSA screening [ ].
In this review, we focus on the clinical role of PSA as a diagnostic biomarker with a particular emphasis on its screening test characteristics, clinically actionable thresholds, and strategies to refine the interpretation of PSA values during screening.
1.1
Discovery and history of PSA
PSA has been identified as a biomarker for prostate cancer since its purification and initial description by Wang et al. in 1979 [ ]. In early studies, PSA was found to be elevated in patients with known prostate cancer reflecting the test’s high sensitivity and thus suggesting a potential role in screening and monitoring response to therapy [ ]. However, PSA was soon recognized to also be elevated in the absence of known prostate cancer, foreshadowing challenges associated with diagnostic specificity [ ]. By 1991, the value of integrating additional stratification tools such as physical examination was noted to refine the specificity of PSA as a prostate cancer biomarker [ ].
1.2
PSA biology
PSA is produced by prostate epithelial cells, including both benign cells and those bearing histologic evidence of cancer. Found in high concentrations in the semen, PSA facilitates the dissolution of the semen coagulum, facilitating sperm transit. PSA is initially secreted as a proenzyme (proPSA) into the prostatic lumen where it is converted into active PSA [ ]. Active PSA can then enter the circulation via diffusion where it may be bound by protease inhibitors [ ]. Alternatively, active PSA can undergo proteolysis and enter circulation as inactive free (unbound) PSA [ ]. Although PSA expression is generally lower in prostate adenocarcinoma as compared with normal prostate tissue, disruption of native cellular architecture allows a greater proportion of PSA to enter circulation, resulting in increased serum PSA concentrations [ , ]. Proteolytic inactivation and cleavage of pro-PSA into PSA is relatively inefficient in prostate cancer, resulting in a smaller fraction of free PSA in circulation compared with benign prostatic tissue [ , ]. Therefore, in addition to measurements of total PSA, quantification of the proportion of free PSA and complexed PSA can be further informative for identifying prostate cancer [ ].
2
PSA measurement
2.1
Normal values and natural variation
There has long been an interest in defining PSA thresholds that constitute normal or abnormal values to simplify clinical decision-making. However, given a wide distribution of expression in both benign and malignant prostatic tissue, single cut points provide tradeoffs in diagnostic sensitivity and specificity. Significant inter and intrapersonal variation in PSA measurement provides an additional challenge in identifying a singular threshold for screening across all populations. In a retrospective analysis of healthy individuals without known prostate cancer, the mean coefficient of variation in consecutive PSA measurements for a given patient was 55.3%, with increases and decreases in PSA seen with roughly equal frequency [ ]. Such variation may also be present in those with prostate cancer as well, further complicating the straightforward interpreteation of a single PSA value.
In response to variation in PSA measurement, efforts have been made to examine the utility of repeat PSA in patients with an elevated levels measurement prior to prostate biopsy. In an analysis of 1,686 men from the Stockholm-3 (STHLM3) study with initial PSA measurements between 3 and 10 ng/mL and 2 PSA tests within 8 weeks and before prostate biopsy, it was found that omitting prostate biopsy in those in whom repeat PSA decreased to levels below 3 ng/ml would avoid 17% of biopsies while missing 5.4% of clinically significant (Gleason score ≥7) prostate cancers [ ]. These findings were supported by a study of a cohort of Italian men undergoing prostate biopsy which demonstrated that a decrease in PSA levels of ≥20% on repeat measurement was associated with a significant reduction in the likelihood of identifying high-grade disease at biopsy [ ]. However a separate analysis of over 7,000 men with initially elevated PSA found that short-term decreases in PSA may also be seen in patients with high-grade disease and thus should not influence the decision to pursue prostate biopsy [ ]. While the optimal interval for PSA re-testing remains unclear, confirmation of abnormal PSA levels is prudent for the evaluation of most patients with a single elevated PSA test and no overt signs of local or distant prostate cancer [ ].
Twin studies estimate that 40% to 45% of the interpersonal variation in PSA levels can be explained by inherited factors [ ]. Genome-wide association studies (GWAS) have identified genetic loci associated with interpersonal PSA variation. In 2010, Gudmundsson et al. identified 6 genetic loci independently associated with PSA which help to explain a component of interpersonal PSA variation [ , ]. In 2017, Hoffmann et al. described a list of 40 SNPs which explain ∼9.5% of variability in non-Hispanic whites [ ]. These studies have motivated initiatives to adjust and personalize PSA measurements based on genetic testing to improve the specificity and sensitivity of PSA. However, a significant amount of variation in PSA measurement remains unexplained.
2.2
Performance test characteristics and cutoffs
Numerous PSA thresholds have been proposed and clinically implemented. A systematic review of pooled data from 9 studies including the Prostate Cancer Prevention Trial (PCPT) and European Randomized Study of Screening for Prostate Cancer (ERSPC) estimated the screening test characteristics of PSA at common cutoffs [ , ]. At a level of 4.0 ng/ml, PSA was estimated to have a specificity of 91%, a sensitivity of 21% for the detection of any prostate cancer, sensitivity of 51% for the detection of high-grade cancer (Gleason score ≥8), and a positive predictive value (PPV) of 30%. Lowering the cutoff to 3.0 ng/ml improved sensitivity for detection of any prostate cancer and high-grade cancer to 32% and 68% respectively, but lowered specificity to 85% and PPV to 28%.
As a result of modest sensitivity and specificity, PSA values in the range of 4.0 to 10.0 ng/ml may represent a diagnostic grey area in which the prevalence of significant prostate cancer is low but not negligible; the cancer detection rate in this range is between 16% and 39% and the rate of high-grade (Gleason score ≥7) disease on biopsy is between 4.1% and 25% [ ]. Moreover, approximately one third of patients with PSA levels between 4.0 and 10.0 ng/ml with prostate cancer are found to have pathologic evidence of extra-capsular invasion at prostatectomy, further underscoring the potential for aggressive disease [ ]. Subsequent studies have highlighted the prevalence of prostate cancer at lower PSA levels, including the observation that nearly 20% of men with diagnosed prostate cancer have PSA levels below 4.0 ng/ml [ , ].
With growing awareness of the indolent nature of low-grade (i.e. Gleason grade group 1) prostate cancer, there is increasing emphasis on focusing risk estimates around clinically significant prostate cancer. Common definitions include the presence of Gleason grade group 2 or higher disease, as well as a minimum linear extent of cancer within biopsy specimens [ ]. Numerous studies have highlighted the prevalence of clinically significant cancers at PSA levels below 4.0 ng/ml. The Prostate Cancer Prevention Trial (PCPT) randomly assigned 18,882 men with a normal DRE and PSA levels ≤3 ng/ml to either finasteride or placebo for 7 years, after which all participants received a prostate biopsy. Analysis of the 5,519 individuals in the placebo arm identified the prevalence of prostate cancer across a range of PSA values. 88.6% of participants in the placebo arm had PSA values less than or equal to 4.0 ng/ml. Of those, 18.7% had prostate cancer and 3.2% had high-grade prostate cancer (Gleason score ≥7) on end-of-study biopsy. Of those with PSA values between 2.1 to 4.0 ng/ml (23.3% of participants), 27.9% had prostate cancer including 7.1% with high-grade disease [ , , ].
2.2.1
Alternative PSA cutoffs
In the European Randomized study of screening for prostate cancer (ERSPC), a multicenter population-based trial conducted in 8 European countries, a PSA cutoff of 3.0 ng/ml was used to guide recommendations for prostate biopsy. When compared to a cutoff of 4.0 ng/ml, lowering the PSA cutoff to 3.0 ng/ml improved the positive predictive value from 18.2% to 24.3% and reduced the number of necessary biopsies to detect 1 case of prostate cancer from 5.2 to 3.4. Cancers detected at a PSA threshold of 3.0 ng/mL had a similar distribution of Gleason scores but a larger proportion of prostate-confined disease. However, even when applying a lower PSA cutoff, investigators have noted that 16% of the cancers detected in the ERSPC were found at a PSA range of 1.0 to 2.9 ng/ml and would have been missed using a cutoff of 3.0 ng/ml [ ].
Alternative thresholds have been suggested to reflect compromises between diagnostic sensitivity and specificity. For example, Krumholtz et al. proposed a PSA cutoff of 2.6 ng/ml based on an analysis of 94 patients who underwent radical prostatectomy. Of the patients, 42 had a PSA between 2.6 and 4.0 ng/ml while the remaining patients had PSA values between 4.0 and 10.0 ng/mL. Based on prostatectomy pathology, patients in the 2.6 to 4.0 ng/ml range had smaller tumor volumes; however, there was no difference in the proportion of “clinically insignificant” cancers (those defined as organ confined, less than 0.2 cm 3 tumor volume, and Gleason score ≤6) between the 2 PSA groups. The study concluded that a PSA cutoff of 2.6 ng/ml may increase the detection of small, organ-confined cancers without over-detecting clinically insignificant ones [ ].
In a screening algorithm described by Crawford et al., a PSA threshold of 1.5 ng/ml was proposed as a cutoff above which follow-up reflex investigation such as biomarker studies or prostate biopsy would be pursued. In this algorithm, if screening PSA was below 1.5 ng/ml (approximately 70% of men who receive screening PSA), primary care providers would consider a 5-year re-screening interval. For patients with screening PSA above 1.5 ng/ml, referral to a specialist or follow-up biomarker tests may be warranted [ ].
Despite the numerous proposed PSA thresholds, there is comparatively little published literature concerning the observed PSA levels that prompt referrals for evaluation and biopsy in the real-world setting, including how these recommendations have been operationalized.
2.2.2
Digital rectal examination (DRE)
Digital rectal examination (DRE) in conjunction with PSA measurement remains one of the most widely utilized prostate cancer screening strategies. Results from a multicenter clinical trial including 6,630 men demonstrated that the use of PSA and DRE together increased detection of organ-confined prostate cancer by 78% compared to DRE alone [ ]. In addition to providing local staging information, DRE as a screening tool has shown utility in patients with aggressive prostate cancer at lower PSA levels in whom further evaluation would not be triggered [ ]. A recent systematic review and meta-analysis of 71,219 patients from randomized trials and prospective diagnostic trials highlighted limitations of both DRE and PSA as screening tests. For example, the pooled PPV for combined DRE and PSA was similar to DRE alone, but was associated with improved cancer detection [ ].
3
How to improve?
Given the challenges of applying a singular PSA cutoff for population-based prostate cancer screening, several refinements have been made to improve the diagnostic performance of PSA. Those with the strongest evidence include adjustments for patient age, prostate volume, and the state of PSA measured.
3.1
Age-PSA relationship
There is a strong relationship between increasing age and serum PSA concentration, in part attributable to age-related increases in prostate volume. [ ]. Longitudinal studies have identified associations among PSA, age, and prostatic volume, including 1 study estimating that for a healthy 60-year-old, serum PSA increases at a rate of 3.2% per year [ , ]. A subsequent retrospective analysis of 4,597 men with clinically localized prostate cancer compared a PSA cut point of 4.0 ng/mL to age-PSA reference ranges of 0 to 2.5 ng/ml (40–49 years), 0 to 3.5 ng/ml (50–59 years), 0 to 4.5 ng/ml (60–69 years) and 0 to 6.5 ng/ml (70–79 years). Age-specific ranges increased detection of more potentially curable tumors in young men and decreased the detection of nonaggressive disease in older men [ ]. In an Austrian screening study of over 21,000 patients, age-specific reference ranges resulted in an 8% increase in the diagnosis of localized cancer in men younger than 59 and resulted in 21% fewer biopsies in men older than 60 compared to using a PSA cutoff of 4.0 ng/ml [ ]. Recognition of age-related distributions may allow for greater personalization when interpreting PSA values.
Wider ranges of clinical thresholds for further evaluation are endorsed by major clinical practice guidelines. For example, the NCCN early detection guidelines recommend referral for diagnostic evaluation for any patient ≤75 years with PSA values >3 ng/mL, however recommend further evaluation at a level of >4 for patients greater than 75 years [ ].
3.1.1
Baseline PSA
PSA levels early in life are prognostic for risks of prostate cancer detection, metastasis, and cancer-related mortality. Prostate carcinogenesis is likely initiated far earlier in life than is detected through screening. Reflecting early differences in PSA distribution, several studies have demonstrated the prognostic role of baseline PSA levels in early and middle age. In a longitudinal study including 21,277 Swedish men, Lilja et al. found that single PSA values measured at or before age 50 were associated with advanced prostate cancer diagnosed up to 30 years later [ ]. Baseline PSA has also been shown to strongly predict future prostate cancer mortality in demographically diverse cohorts, suggesting that the potential benefits of risk-stratified screening based on midlife PSA could apply broadly at the population level [ ].
Given differences in longitudinal risk baseline PSA has been proposed as a means to tailor the frequency of screening. For example, Kovac et al. found that the 13-year incidence of clinically significant prostate cancer (defined as clinical stage of cT2b or greater, biopsy Gleason grade of 7 or greater, or prostate cancer-specific mortality) was 0.4% in those with a baseline PSA less than 0.49, 1.5% at a baseline PSA between 0.5 and 1.0 ng/ml, and 5.4% at a baseline PSA between 1.0 and 1.99 ng/ml, suggesting that screening intensity could be reduced in individuals with a baseline PSA below 2.0 ng/ml and possibly discontinued in individuals with a baseline PSA below 1.0 ng/ml [ ].
3.2
PSA density
Serum PSA is correlated with prostate gland volume [ ], and in 1992 Benson et al. described PSA density (PSAD) as a correction factor to improve discrimination between prostate cancer and benign conditions [ ]. PSAD reflects the ratio of serum PSA concentration to prostate volume, generally estimated by transrectal ultrasound (TRUS) or magnetic resonance imaging (MRI). Early studies suggested that a PSAD cutoff of 0.15 ng/ml/cc could improve discrimination of prostate cancer and BPH, with higher PSAD values suggesting cancer [ , ]. However, use of PSAD alone to select patients for biopsy appears to be an imperfect approach to identifying patients with prostate cancer [ ].
With the growing utilization of prebiopsy imaging, prostate MRI and PSAD appear to be complementary tools for risk refinement. In a systematic review and meta-analysis, Wang et al studied the diagnostic performance of PSAD combined with prebiopsy MRI in the detection of clinically significant prostate cancer. Definitions of clinical significance varied across studies with a default definition of Gleason score ≥7 or Gleason Group ≥2, and the definition of a positive MRI similarly varied across studies with a default definition of any MRI-visible lesion that was targeted (PI-RADS or Likert 3–5 lesions). Among patients with a positive MRI, the sensitivity and specificity for diagnosing clinically significant prostate cancer were, respectively, 87% and 35% for a PSAD cutoff of 0.1 ng/ml/cc, 74% and 61% for a PSAD cutoff of 0.15 ng/ml/cc, and 51% and 81% for a PSAD cutoff of 0.2 ng/ml/cc. PSAD was particularly useful in ruling out clinically significant prostate cancer in patients with a negative MRI or those with PI-RADS or Likert 3 lesions. In this subset, the probability of identifying clinically significant prostate cancer in those with PSAD <0.10 ng/ml/cc is very low (4% for those with negative MRI and 6% for those with PI-RADS or Likert 3 lesions), potentially reducing the need for biopsy in these patients [ ].
Although TRUS has been historically used to measure prostate gland volume, MRI has been increasingly used for volume estimates integrated into PSAD calculations. A recent study comparing TRUS and multiparametric MRI (mpMRI) in 640 patients found that TRUS underestimates prostate volume compared to mpMRI and that PSAD calculated from mpMRI had higher odds than TRUS PSAD to detect any prostate cancer as well as clinically significant (Gleason score ≥7) prostate cancer [ ]. New approaches leveraging machine-learning-based image analysis have also been utilized for the automated calculation of PSAD from mpMRI imaging. Automated PSAD has demonstrated significant improvements in estimates of prostate volume and PSAD compared to an experienced radiologist, potentially reducing errors in PSAD calculation [ ].
3.3
Free PSA
Prostate cancer is associated with a reduction of free PSA detected in the serum (i.e. the fraction unbound to protein). As a result, the percentage of free:total PSA has been clinically incorporated as both a dichotomized cutoff or as part of an individual patient’s cancer risk assessment. For example, in a study of 773 patients with PSA levels between 4.0 and 10.0 ng/ml who received ultrasound-guided prostate biopsy, a cutoff of <25% free PSA would detect 95% of cancers while avoiding 20% of unnecessary biopsies. Analysis of the subset of patients who underwent prostatectomy demonstrated a significant association between free PSA and the probability of favorable pathology (defined as Gleason score <7, organ-confined [stages T1 and T2], tumor volume ≤10% of gland) as percentage of free PSA increased (34% with favorable pathologic findings for patients with values ≤15% free PSA compared to 70% for patients with >25% free PSA). Thus, the cancers identified in those with greater than 25% free PSA harbored lower risk features [ ]. Another study found that in patients with PSA levels between 2.0 and 4.0 ng/ml, a cutoff of 27% free PSA would have detected 90% of cancers and avoided 18% of benign biopsies while yielding a positive predictive value of 24% among those who underwent biopsy [ ].
Further evidence supports the long-term prognostic utility of percent-free PSA measurements. A recent analysis of a large U.S. screening trial consisting of 6,727 men within the intervention arm of PLCO (Prostate, Lung, Colorectal, and Ovarian Cancer Screening Trial) with baseline PSA ≥2 ng/ml found that the addition of percentage free PSA was independently associated with risks of clinically significant prostate cancer (hazard ratio, HR for % free PSA as a continuous variable per 1% decrease 1.05, 95% confidence interval 1.04–1.06) and fatal prostate cancer (HR for % free PSA as a continuous variable per 1% decrease 1.02, 95% CI 1.02–1.04). Moreover, the addition of percent-free PSA to total PSA improved the discriminative ability of multivariable Cox proportional hazards regression models for the prediction of both clinically significant prostate cancer (Gleason score ≥7) and fatal prostate cancer [ ]. However, these results must be taken in study context; percent-free PSA was measured in less than 20% of men in the intervention arm of PLCO and the trial is known to have suffered significant crossover bias, underscoring the problem of study heterogeneity with regards to not just percent-free PSA, but all PSA metrics. Nonetheless, clinical guidelines endorse the use of refined risk estimates to inform shared decision-making among patients with elevated PSA levels. The 2023 AUA guideline [ ] highlights the use of multivariable risk calculators such as the Prostate Cancer Prevention Trial (PCPT) risk calculator which includes % free PSA as well as several other risk factors including race, age, PSA, family history, DRE, prior biopsy and urinary PCA3 levels [ ]. However, neither the AUA guideline nor the NCCN guideline explicitly comments on the role of free PSA as a stand-alone test to prompt further testing and an optimal cutoff value remains unclear.
3.4
PSA kinetics
Another strategy to improve the performance of PSA in cancer detection is to account for change in PSA over time. An increase in PSA velocity has been associated with prostate cancer [ ] and may add specificity to total PSA measurements. In 1992, Carter et al. reported that a PSA velocity cutoff of 0.75 ng/ml per year distinguished patients with prostate cancer who were diagnosed at prostatectomy or sextant biopsy from those with BPH (pathologically confirmed diagnosis at prostatectomy) and healthy control subjects with a specificity of 90% and 100% respectively [ ]. Very high PSA velocity (>3 ng/ml per year) may be more consistent with inflammatory conditions on biopsy than cancer [ ]. PSA velocity may also provide long-term prognostic information. D’Amico et al found that patients with high PSA velocities have a higher risk of postradical prostatectomy death and postradiation therapy death due to prostate cancer [ , ]. An analysis of 89 patients in the Baltimore Longitudinal Study of Aging with PSA values between 2.0 and 4.0 ng/ml for at least 18 months found that PSA velocity may be useful in prostate cancer risk assessment in men with PSA levels below 4.0 ng/mL [ ].
However, more contemporary large-scale studies in Europe and the U.S. have shown that PSA velocity does not meaningfully improve estimates of significant prostate cancer on biopsy. In an analysis of 2,742 screening-arm participants in the ERSPC with initial PSA <3.0 ng/ml who were subsequently biopsied due to elevated PSA, addition of PSA velocity to logistic regression models adjusting for age and PSA led to small improvement in predictive accuracy for the detection of any cancer (Area Under Curve (AUC) of 0.569 vs. 0.531). However, there was no meaningful improvement in predictive accuracy for high-grade disease defined as Gleason score ≥7 (AUC of 0.683 vs. 0.689) [ ]. In a similar analysis of 5,519 participants undergoing biopsy in the PCPT, logistic regression models adjusting for age, PSA, DRE, family history, and prior biopsy showed only a very small increase in model performance for detection of any cancer with the addition of PSA velocity (AUC of 0.702 vs. 0.709). These improvements were even smaller for the endpoints of high-grade cancer (Gleason score ≥7) and clinically significant cancer (Epstein criteria [ ]). Of note, PSA cutoffs with a comparable specificity (87%) to PSA velocity cutoffs had a higher sensitivity for detection of any cancer (23% vs. 19%), particularly for high-grade (41% vs. 25%) and clinically significant (32% vs. 22%) cancers, drawing into question the rationale for a formal calculation of PSA velocity [ ]. Intrapatient variation in PSA also limits the clinical utility of PSA velocity, with at least 3 consecutive measurements required to establish a PSA velocity [ ]. Finally, there may be redundancy in PSA velocity given that individuals with high PSA velocities will rapidly have their PSA rise above traditional cutoff values and thus would likely be detected using traditional PSA screening. Ultimately, the 2023 AUA guideline on early detection of prostate cancer notes that PSA velocity should not be used as the solitary factor for a secondary biomarker, imaging, or biopsy [ ].
3.5
Genetic adjustment
Genetically attributable variation in PSA production contributes to interpersonal variation in PSA levels which may impact performance as a screening tool. As a result, adjustment for genetic factors has been increasingly explored to improve risk estimates. In the STHLM3 study, an individualized prediction model (S3M) was compared to PSA ≥3 ng/ml as a screening test for prostate cancer. The model included a combination of plasma protein biomarkers (including PSA), genetic polymorphisms, and clinical variables (age, family history, previous prostate biopsy, prostate exam). With maintained sensitivity for detecting Gleason score ≥7 disease, use of S3M was estimated to avoid 32% of prostate biopsies compared to screening using PSA ≥3 ng/ml as an indication for a prostate biopsy [ ]. Such a model could also improve prostate cancer screening specificity when utilized as a second test—a reflex test—for individuals with elevated PSA [ ]. Other methods of genetic PSA adjustment have also been explored. In a recent GWAS study including 95,768 individuals, Kachuri et al developed a PSA polygenic score (PGSPSA) that explained 9.61% of constitutive PSA variation. Clinical implementation of the PGS-adjusted PSA was estimated to avoid up to 31% of negative biopsies but also result in 12% fewer biopsies in patients with prostate cancer, mostly with Gleason grade group <2 tumors [ ].
4
Conclusions
Widespread PSA screening has shaped the diagnosis, treatment and outcome of prostate cancer. Although PSA as a biomarker has enabled the early detection of prostate cancer, there is active continued debate about its optimal integration including optimal thresholds for further evaluation. In this review, we examined the performance of PSA as a diagnostic prostate cancer biomarker including inherent limitations relating to inter/intrapersonal variation, overlap with benign prostatic conditions, and the presence of clinically significant cancers across a wide range of PSA values.
Many strategies have been utilized to improve the performance of PSA accounting for age, prostate volume, genetic factors, and the biochemistry of PSA. Despite these advancements in testing, most have not been widely adopted, with many practitioners still relying on total PSA and a cutoff of 4.0 ng/ml as the sole indication for biopsy. Regardless of cutoff, total PSA alone will likely never have optimal specificity and sensitivity for population-level screening. However, as PSA is further refined and supplemental technologies improve, PSA will likely continue to play a key role in prostate cancer screening.
CRediT authorship contribution statement
Vinaik M. Sundaresan: Conceptualization, Writing – original draft, Writing – review & editing. Shayan Smani: Writing – original draft. Pawel Rajwa: Conceptualization, Writing – original draft. Joseph Renzulli: Writing – original draft. Preston C. Sprenkle: Conceptualization, Writing – original draft. Isaac Y. Kim: Conceptualization, Supervision, Writing – original draft. Michael S. Leapman: Conceptualization, Formal analysis, Methodology, Project administration, Resources, Supervision, Writing – original draft, Writing – review & editing.
Declaration of competing interest
The authors declare that they have no known competing financial interests or personal relationships that could have appeared to influence the work reported in this paper.
References
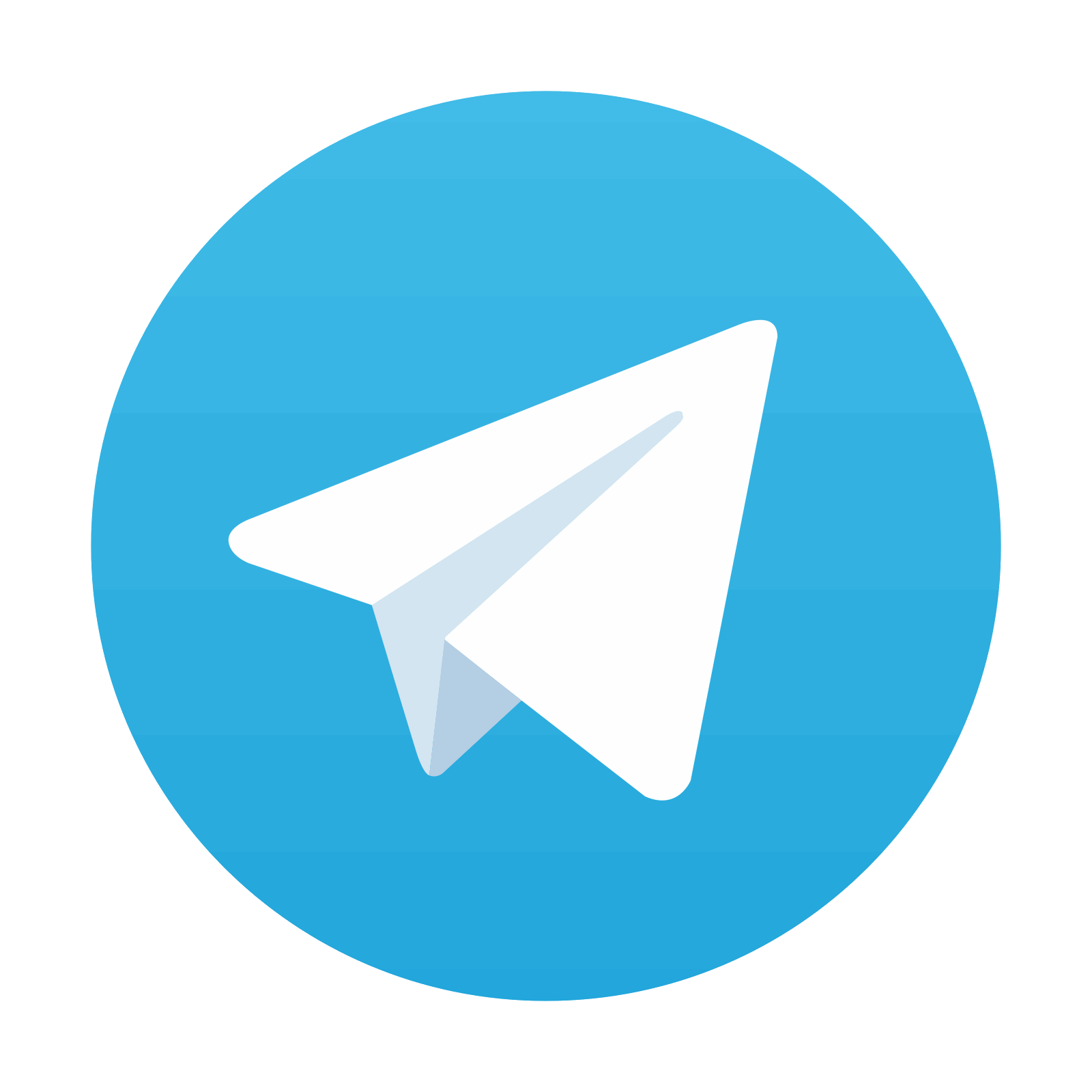
Stay updated, free articles. Join our Telegram channel

Full access? Get Clinical Tree
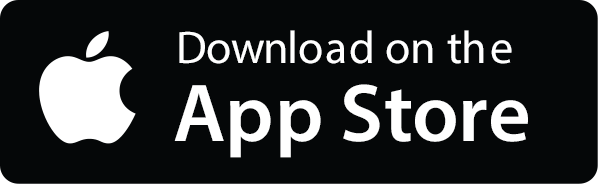
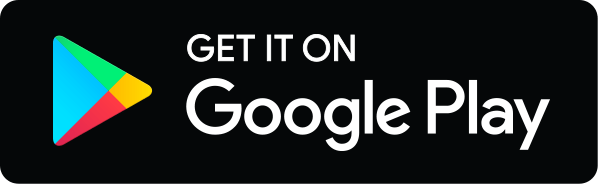
