Soft tissue sarcoma (STS) staging is a constantly evolving process. Grading is still of utmost importance and has been adapted into a three-tier system. The STS most difficult to categorize are those with uncertain malignant potential, such as solitary fibrous tumors, gastrointestinal stromal tumors, and glomus tumors, some of which have developed completely separate staging systems and may not even be considered sarcomas. Beyond the current TNM staging system, a multitude of prognostic factors for STS will continue to be discovered and ultimately incorporated into future revisions of the staging system.
Incidence and epidemiology
Soft tissue sarcomas (STSs) represent fewer than 1% of all adult solid malignancies. In 2010, an estimated 10,520 new cases of STS and 3920 deaths occurred from the disease. Overall 5-year survival for extremity STS is 50% to 90%; however, this varies greatly based on the grade and anatomic site. In general, the estimated 5-year survival is 25% to 50% for retroperitoneal sarcoma, which is poor compared with extremity STS. The prognosis for patients with high-grade retroperitoneal sarcoma is less favorable than for those with tumors at other sites because of the technical complexity of completely resecting these tumors and limitations to delivering high-dose radiation therapy to the abdomen. The foundation for staging and grading systems for STS arose in the late 1970s and early 1980s when a task force convened to discuss these issues after one of the earliest publications on staging.
STSs are putatively derived from the mesenchymal cells during embryologic development, including fibrous connective tissue, fat, smooth or striated muscle, vascular tissue, peripheral neural tissue, and visceral tissue. Approximately 50% to two-thirds involve the extremities and 40% the trunk and retroperitoneum, with approximately 10% arising from the head and neck. The most common subtypes are malignant fibrous histiocytoma (pleomorphic undifferentiated sarcoma), liposarcoma, leiomyosarcoma, synovial sarcoma, and malignant peripheral nerve sheath tumor ( Table 1 ). In children, rhabdomyosarcoma is the most common STS, although it ranks as seventh overall when combining adults and children. Other entities not included are Kaposi sarcoma, malignant extrarenal rhabdoid tumor, malignant tenosynovial giant cell tumor, malignant ossifying fibromyxoid tumor, malignant perivascular epithelioid cell tumors, desmoplastic round cell tumor, malignant glomus tumor, malignant triton tumor, and malignant gastrointestinal stromal tumor. Terminology for two of the listed tumors in Table 1 has been altered to malignant hemangiopericytoma-solitary fibrous tumor and Ewing’s sarcoma/primitive neuroectodermal tumor . With ongoing improvements in molecular pathology, probably more than 50 histologic subtypes of STS exist.
Histologic Subtypes | n | % |
---|---|---|
Malignant fibrous histiocytoma | 349 | 28 |
Liposarcoma | 188 | 15 |
Leiomyosarcoma | 148 | 12 |
Synovial sarcoma | 125 | 10 |
Malignant peripheral nerve sheath tumor | 72 | 6 |
Rhabdomyosarcoma | 60 | 5 |
Fibrosarcoma | 38 | 3 |
Ewing’s sarcoma | 25 | 2 |
Angiosarcoma | 25 | 2 |
Osteosarcoma | 14 | 1 |
Epithelioid sarcoma | 14 | 1 |
Chondrosarcoma | 13 | 1 |
Clear cell sarcoma | 12 | 1 |
Alveolar soft part sarcoma | 7 | 1 |
Malignant hemangiopericytoma | 5 | 0.4 |
Despite the variety of histologic subtypes, STSs have many clinical and pathologic features in common. Because STSs are biologically similar and traditionally treated the same, this allows for common staging systems, which may not be appropriate. The American Joint Committee on Cancer (AJCC) staging system attempts to incorporate this commonality through only addressing anatomic depth, grade, and size of the tumor.
STSs metastasize primarily via a hematogenous route, particularly to the lungs. Lymph node metastasis is rare (<5%), except for a few histologic subtypes, such as epithelioid sarcoma, synovial sarcoma, rhabdomyosarcoma, clear-cell sarcoma, and angiosarcoma. Local control is the primary treatment goal, with acceptable limb salvage rates for extremity STS. Selective metastasectomy also has a role. Five-year overall survival for patients who undergo metastasectomy is 30% if all metastatic disease is resected.
Although the development of most STS is spontaneous, some STSs occur after a previous insult or exposure to carcinogenic agents. Radiation exposure accounts for only approximately 5% of all STSs, but a history of external beam radiation (e.g., for breast, cervix, ovarian, or lymphatic malignancies) is a well-known risk factor with an 8- to 50-fold increased incidence. The risk of STS is directly related to the dose of radiation, and there appears to be a latency period of approximately 10 years. The most common histologic subtypes of radiation-associated sarcoma are extraskeletal osteogenic (21%), malignant fibrous histiocytoma (16%), and angiosarcoma/lymphangiosarcoma (15%). Most of these tumors (87%) are also high grade. Other risk factors include exposure to certain chemicals, including herbicides such as phenoxyacetic acids, and wood preservatives such as chlorophenols. Chronic lymphedema after axillary node dissection has been associated with lymphangiosarcoma, otherwise known as Stewart-Treves syndrome. Lymphangiosarcoma has also been associated with filarial infections and congenital lymphedema, seen predominantly on the lower extremities. Certain viruses are linked to the development of sarcomas, including human immunodeficiency virus 1, human herpes virus 8 in Kaposi’s sarcoma, and Epstein-Barr Virus in leiomyosarcoma.
Genetics
Genetic mutations in mesenchymal stem cells give rise to malignant clones. STS are sometimes associated with specific inherited genetic alterations, but most have complex karyotypes. Numerous oncogenes have been implicated in the development of STS, including MDM2 (liposarcoma), N-myc (Ewing sarcoma), c-erbB2 (Ewing sarcoma), and members of the ras family (malignant fibrous histiocytoma, embryonal rhabdomyosarcoma). In addition to being important for diagnostic purposes, amplification of several of these genes in specific STS subtypes has been associated with a worse outcome. Mutations in specific oncogenes result in chromosomal translocations that are associated with some histologic subtypes of STS. For instance, Ewing’s sarcoma is associated with EWS-FLI1 fusion, clear cell sarcoma with EWS-ATF1 fusion, myxoid liposarcoma with TLS-CHOP fusion, alveolar rhabdomyosarcoma with PAX3-FHKR fusion, desmoplastic small round-cell tumor with EWS-WT1 fusion, and synovial sarcoma with SSX-SYT fusion ( Table 2 ). All of these tumors are considered high-grade STSs and are most often associated with extensive cytogenetic abnormalities. Multiple inherited syndromes have STS components, including neurofibromatosis type I, retinoblastoma, Li-Fraumeni syndrome, Gardner syndrome, Werner syndrome, Gorlin syndrome, Carney triad, and tuberous sclerosis.
Sarcoma Type | Chromosomal Translocation | Fusion Gene | Year Reported |
---|---|---|---|
Ewing sarcoma | t(11;22)(q24;q12) t(21;22)(q22;q12) | EWS-FLI1 EWS-ERG | 1992 1993 |
Clear cell sarcoma | t(12;22)(q13;q12) | EWS-ATF1 | 1993 |
Desmoplastic small round cell tumor | t(11;22)(p13;112) | EWS-WT1 | 1994 |
Extraskeletal myxoid chondrosarcoma | t(9;22)(q22;q12) | EWS-CHN | 1995 |
Myxoid/round cell liposarcoma | t(12;16)(q13;p11) | TLS-CHOP | 1993 |
Angiomatoid fibrous histiocytoma | t(12;16)(q13;p11) | TLS-ATF1 | 2000 |
Alveolar rhabdomyosarcoma | t(2;13)(q35;q14) t(1;13)(p36;q14) | PAX3-FKHR PAX7-FKHR | 1993 1994 |
Extraskeletal myxoid chondrosarcoma | t(9;17)(q22;q11) | TAF2N-CHN | 1999 |
Synovial sarcoma | t(X;18)(p11;q11) | SYT-SSX1,2 | 1994 |
Dermatofibrosarcoma protuberans | t(17;22)(q22;q13) | COL1A1-PDGFB | 1997 |
Congenital fibrosarcoma | t(12;15)(p13;q25) | ETV6-NTRK3 | 1998 |
Inflammatory myofibroblastic tumor | t(2p23) | Various ALK fusions | 2000 |
Alveolar soft part sarcoma | t(X;17)(p11;q25) | ASPL-TFE3 | 2001 |
Endometrial stromal sarcoma | t(7;17)(p15;q21) | JAZF1-JJAZ1 | 2001 |
In addition to oncogenes, tumor suppressor genes can be inactivated by either hereditary or sporadic mechanisms, and allow for uninhibited growth of tumor cells. Two tumor suppressor genes that are particularly relevant to STS are retinoblastoma ( Rb-1 ) and p53 . Mutations or deletions in the Rb gene can result in the development of retinoblastomas and sarcomas of soft tissues and bone. Mutations in the p53 tumor suppressor gene are the most common cytogenetic alteration in all human solid tumors, and have been associated with 30% to 60% of all STS. Li-Fraumeni syndrome is also associated with this mutation.
Genetics
Genetic mutations in mesenchymal stem cells give rise to malignant clones. STS are sometimes associated with specific inherited genetic alterations, but most have complex karyotypes. Numerous oncogenes have been implicated in the development of STS, including MDM2 (liposarcoma), N-myc (Ewing sarcoma), c-erbB2 (Ewing sarcoma), and members of the ras family (malignant fibrous histiocytoma, embryonal rhabdomyosarcoma). In addition to being important for diagnostic purposes, amplification of several of these genes in specific STS subtypes has been associated with a worse outcome. Mutations in specific oncogenes result in chromosomal translocations that are associated with some histologic subtypes of STS. For instance, Ewing’s sarcoma is associated with EWS-FLI1 fusion, clear cell sarcoma with EWS-ATF1 fusion, myxoid liposarcoma with TLS-CHOP fusion, alveolar rhabdomyosarcoma with PAX3-FHKR fusion, desmoplastic small round-cell tumor with EWS-WT1 fusion, and synovial sarcoma with SSX-SYT fusion ( Table 2 ). All of these tumors are considered high-grade STSs and are most often associated with extensive cytogenetic abnormalities. Multiple inherited syndromes have STS components, including neurofibromatosis type I, retinoblastoma, Li-Fraumeni syndrome, Gardner syndrome, Werner syndrome, Gorlin syndrome, Carney triad, and tuberous sclerosis.
Sarcoma Type | Chromosomal Translocation | Fusion Gene | Year Reported |
---|---|---|---|
Ewing sarcoma | t(11;22)(q24;q12) t(21;22)(q22;q12) | EWS-FLI1 EWS-ERG | 1992 1993 |
Clear cell sarcoma | t(12;22)(q13;q12) | EWS-ATF1 | 1993 |
Desmoplastic small round cell tumor | t(11;22)(p13;112) | EWS-WT1 | 1994 |
Extraskeletal myxoid chondrosarcoma | t(9;22)(q22;q12) | EWS-CHN | 1995 |
Myxoid/round cell liposarcoma | t(12;16)(q13;p11) | TLS-CHOP | 1993 |
Angiomatoid fibrous histiocytoma | t(12;16)(q13;p11) | TLS-ATF1 | 2000 |
Alveolar rhabdomyosarcoma | t(2;13)(q35;q14) t(1;13)(p36;q14) | PAX3-FKHR PAX7-FKHR | 1993 1994 |
Extraskeletal myxoid chondrosarcoma | t(9;17)(q22;q11) | TAF2N-CHN | 1999 |
Synovial sarcoma | t(X;18)(p11;q11) | SYT-SSX1,2 | 1994 |
Dermatofibrosarcoma protuberans | t(17;22)(q22;q13) | COL1A1-PDGFB | 1997 |
Congenital fibrosarcoma | t(12;15)(p13;q25) | ETV6-NTRK3 | 1998 |
Inflammatory myofibroblastic tumor | t(2p23) | Various ALK fusions | 2000 |
Alveolar soft part sarcoma | t(X;17)(p11;q25) | ASPL-TFE3 | 2001 |
Endometrial stromal sarcoma | t(7;17)(p15;q21) | JAZF1-JJAZ1 | 2001 |
In addition to oncogenes, tumor suppressor genes can be inactivated by either hereditary or sporadic mechanisms, and allow for uninhibited growth of tumor cells. Two tumor suppressor genes that are particularly relevant to STS are retinoblastoma ( Rb-1 ) and p53 . Mutations or deletions in the Rb gene can result in the development of retinoblastomas and sarcomas of soft tissues and bone. Mutations in the p53 tumor suppressor gene are the most common cytogenetic alteration in all human solid tumors, and have been associated with 30% to 60% of all STS. Li-Fraumeni syndrome is also associated with this mutation.
Clinical presentation and staging
The most common clinical presentation of STS is a painless and gradually enlarging mass. Sometimes trauma to the site may call attention to the presence of the sarcoma, but trauma is not necessarily a causative factor. In general, tumors of the thigh and retroperitoneum are larger on initial presentation than distal extremity tumors, because these spaces can conceal a mass much longer before it becomes noticeable. Often a zone of compression or pseudocapsule exists if no anatomic constraints are present, which can lead to pressure, paresthesias, distal edema, or bowel obstruction. Pseudocapsules will almost always have viable tumor in them, particularly in high-grade tumors. Different histologies can have varying growth rates and associated effects on the surrounding normal tissues. For example, liposarcoma is characteristically a “pushing” tumor, whereas synovial sarcoma is infiltrative.
Preoperative imaging is essential for surgical planning (for the biopsy and the resection). When inadequate, this can contribute to margin-positive primary resections and the need for reexcision for residual disease in as many as 39% to 87% of patients subsequently seen at tertiary care centers. A combination of CT and MRI are used in preoperative planning. Contrary to other tumors in which chest radiograph may be adequate for assessing pulmonary metastases, chest CT is typically indicated for ruling out pulmonary disease in STS. CT of the abdomen and pelvis is used to characterize retroperitoneal STS. No studies have compared CT and MRI in retroperitoneal STS. However, double-contrast CT of the abdomen and pelvis is most commonly used to identify liver metastases, define vital structures that might require en bloc resection, and identify surrounding organs at risk for radiation exposure.
MRI plays a central role in STS management and planning, particularly for structures affected by motion artifact, such as the extremities. MRI is capable of producing images in a variety of planes and often has more familiar surgical, anatomic, or conventional radiographic orientations. Several authors have compared CT with MRI in the evaluation of extremity STS and have shown MRI to be superior in delineation between tumor and muscle, vessels, fat, and bone, but not between tumor and nerve. Overall, MRI seems to be better for extremity STS. Multiple studies have also shown MRI to have superior accuracy for predicting STS resectability compared with CT. MRI is also superior to CT in identifying muscle compartment invasion by the sarcoma, leading to improved clinical staging. Despite most studies favoring MRI over CT, CT was found to be equivalent to MRI by the Radiation Diagnostic Oncology Group (RDOG). The RDOG prospectively studied 133 patients with primary STS across several institutions and found that CT was equivalent to MRI for determining involvement of muscle, bone, joint, and neurovasculature. Therefore, CT is an excellent alternative in patients who cannot get an MRI, and should have similar ability to predict tumor invasion or help plan the operative strategy for limb-sparing resection in extremity STS.
The role of PET scan for STS is still being examined and defined. It has been shown to correlate with STS grade and can be helpful for identifying distant metastases in patients with high-risk tumors. A recent study from Germany using 18(F)-fluorodeoxyglucose (FDG)-PET showed that tumor glycolytic phenotype (standardized uptake value [SUV]) correlated significantly with histologic grade, as determined by both the Fédération Nationale des Centres de Lutte Contre le Cancer (FNCLCC) and two-tier (high vs low) grading systems. PET/CT has also been shown to be accurate and sensitive for detecting lymph node and bony metastases. Finally, FDG-PET has been used to determine response to neoadjuvant chemotherapy for stratifying histopathologic response in patients with high-grade sarcomas.
Diagnostic biopsy
Orientation of the STS biopsy is critical for preoperative planning in that the trajectory and scar should be parallel to the long axis of the extremity and lie within the area of subsequent en bloc tumor resection. Fine-needle aspiration (FNA) biopsy is classically described using a 21-gauge needle and a 10-mL syringe with several passes through the tissue and stained with Giemsa, Papanicolaou, and hematoxylin-eosin stains. At select major sarcoma centers, FNA biopsy is frequently used for diagnostic purposes. Tertiary care institutions report 89% sensitivity in detecting malignancy, with 95% accuracy in grading when using FNA. However, the accuracy of FNA biopsy is directly related to the skill and experience of the pathologist interpreting the sample.
Diagnostic percutaneous core needle biopsy is safe and reliable, and can be performed with local anesthesia for palpable masses or in conjunction with CT, ultrasound, or MRI for deep-seated tumors. Core needle biopsy can determine the STS histologic subtype and grade. Its main advantage over FNA biopsy is that it provides the pathologist with the histologic architecture of the tumor, which is often critical for diagnosing STS. Diagnostic accuracy of core needle biopsy is 95% to 99% compared with incisional biopsy. Other groups propose using a combination of FNA and core needle biopsies to improve diagnostic accuracy (77% positive identification of subtype and 90% positive identification of grade) and efficiency, nearly eliminating the need for incisional biopsy.
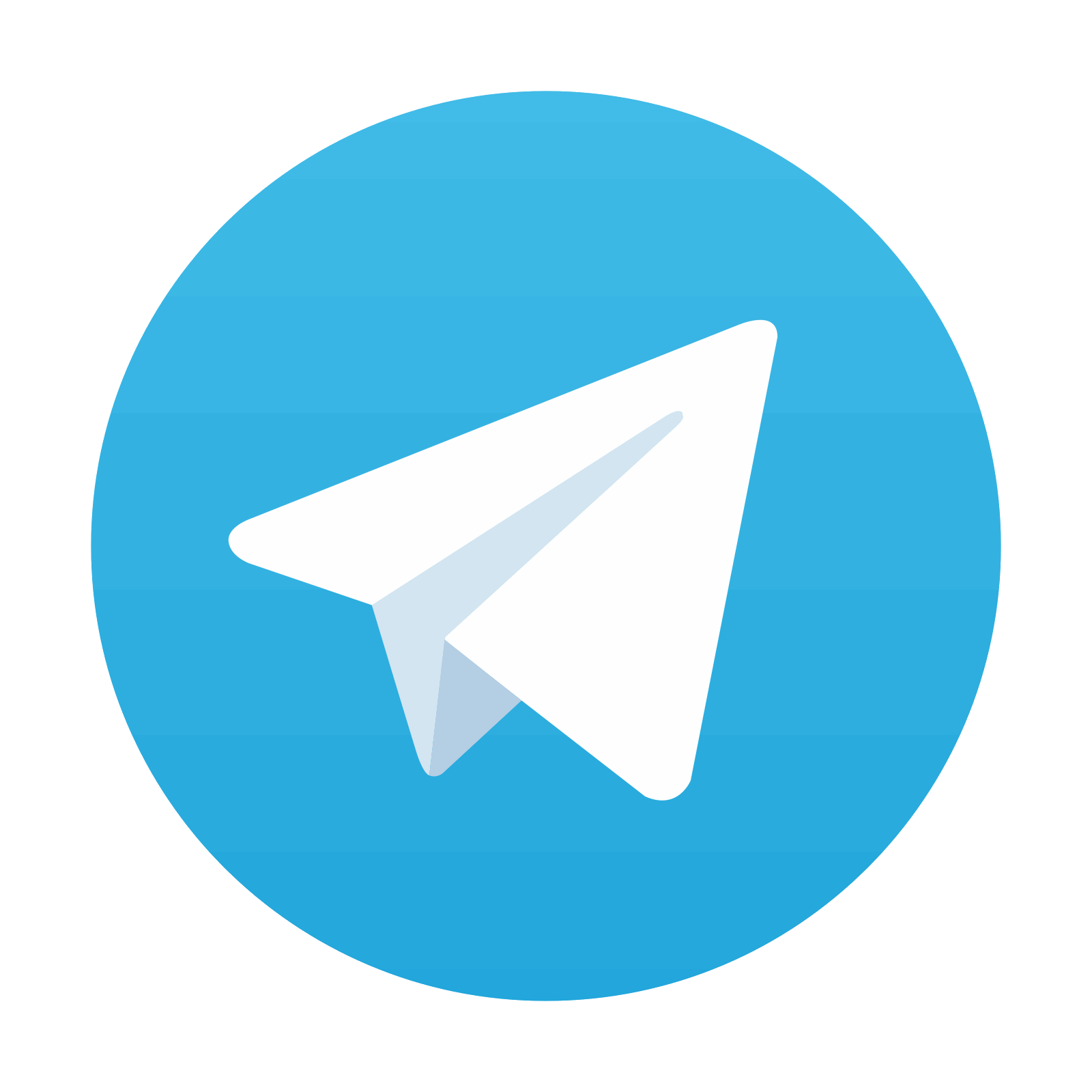
Stay updated, free articles. Join our Telegram channel

Full access? Get Clinical Tree
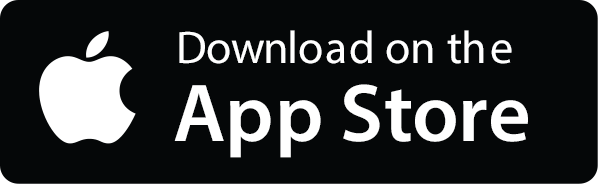
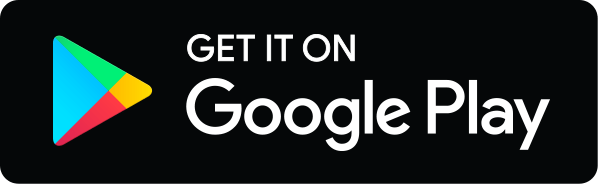