Principles of Cancer Immunotherapy
Mario Sznol
I. INTRODUCTION
Agents that produce anticancer activity through one or more components of the immune system play an important and, in some cases, a predominant role in the treatment of several malignancies. The broad relevance of antitumor immunity to cancer therapeutics is likely underappreciated. For example, components of the immune system may contribute to the overall activity of certain cytotoxic and molecular targeted agents, and also to the activity of therapeutic antibodies against cell surface receptors with oncogenic function.1,2,3,4,5,6 The graft-versus-tumor effect in allogeneic bone marrow transplant can be considered a form of cancer immunotherapy. This chapter reviews the principles of cancer treatment with agents whose principal activity is mediated by the cells of the immune system.
II. ANTICANCER IMMUNE RESPONSES
The majority of effective immunotherapies produce tumor regression through induction, expansion, or activation of T-lymphocytes (T-cells) that recognize tumor antigens. Through their T-cell receptors (TCR), T-cells recognize peptide fragments of proteins that are processed intracellularly, bound to major histocompatibility complex (MHC) molecules, and then brought to the cell surface. Studies in patients have shown that T-cell responses against peptides from mutated proteins (neoantigens), tissue differentiation proteins such as MART-1 in melanoma, or developmental proteins re-expressed during malignant transformation (e.g., NY-ESO-1) can mediate tumor regression.7,8,9
Naïve T-cells are first activated by interactions with professional antigen-presenting cells (APCs, also called dendritic cells) in lymph nodes. Once activated, T-cells differentiate into effector cells and long-lived memory cells. In addition to the signal received by the TCR, T-cell activation, differentiation, and function are controlled by multiple soluble cytokines (hormone-like substances) or cell surface ligands on other cells (including APC and tumor cells) interacting with receptors on the T-cell surface.10 Because the immune system must have the capacity to eliminate foreign invaders while limiting autoimmunity and tissue damage, multiple mechanisms exist not only to provide activation signals but also to delete, inhibit, or terminate immune responses. Multiple types of T-cells with multiple functions can
be involved in the generation of an immune response, including regulatory T-cells (Tregs) that are capable of suppressing the function of effector cells. For T-cells to produce an antitumor response, they must migrate back to the tumor site where they produce cytokines or kill tumor cells directly following TCR binding to the tumor peptide-MHC complexes.11
be involved in the generation of an immune response, including regulatory T-cells (Tregs) that are capable of suppressing the function of effector cells. For T-cells to produce an antitumor response, they must migrate back to the tumor site where they produce cytokines or kill tumor cells directly following TCR binding to the tumor peptide-MHC complexes.11
Anticancer T-cell responses can be enhanced by several types of interventions. The interventions can include providing the putative tumor antigen in the form of an optimized “vaccine”; providing signals that enhance APC function; providing cytokines that support T-cell expansion and function; administering antibodies that directly activate costimulatory receptors or block inhibitory receptors on T-cells; administering agents that inhibit other T-cell-suppressive mechanisms within the tumor microenvironment; infusing T-cells with natural or engineered recognition of tumor cells; or combining one or more of the possible immunomodulating strategies. The type of intervention necessary to produce effective antitumor immune responses is highly dependent on the nature of the tumor-host relationship in the individual patient, which can be influenced by many factors, including host genetic variations in immune response, the number and type of tumor mutations, prior exposures to foreign antigens, and possibly even by the microbiome. In a substantial number of patients, the immune system is able to mount a T-cell response against tumor antigens during the course of time in which the malignancy is evolving in the host. In fact, inflammation may promote tumor growth at the early stages of tumor development, and likely controls the developing cancer for a period of time until the tumor develops escape mechanisms.12 Consistent with the latter observations, multiple factors that are capable of suppressing effector tumor antigen-specific T-cells within the tumor microenvironment have been identified. One of the most important is induced by tumor-infiltrating T-cells mounting the anticancer response. Activated T-cells produce cytokines, particularly interferon-γ (IFN-γ), which induce PD-L1 expression by tumor cells, stromal cells, or other immune infiltrating cells. PD-L1 then binds to the inhibitory receptor PD-1 on the activated T-cells, suppressing their function.13
Chronic exposure of tumor-specific T-cells to tumor antigens either within the tumor or in draining lymph nodes produces further “exhaustion” of the T-cells, with diminished capacity to produce cytokines or to proliferate.14 The exhausted T-cells are characterized by expression of multiple other coinhibitory receptors. Other factors that could inhibit effector T-cells include Tregs, myeloid-derived suppressor cells, a subset of macrophages, or possibly other stromal cells such as vascular endothelium. Inhibition may require direct cell-cell contact or production of inhibitory substances (e.g., enzymes that deplete essential amino acids and/or produce inhibitory metabolites, or cytokines that inhibit or deviate T-cell function or contribute to formation
of Tregs). While not yet proven, a subset of tumors containing minimal to no T-cell infiltrate may actively “exclude” T-cells from the microenvironment by mechanisms which remain to be defined.
of Tregs). While not yet proven, a subset of tumors containing minimal to no T-cell infiltrate may actively “exclude” T-cells from the microenvironment by mechanisms which remain to be defined.
The targets for agents that modulate T-cell responses can be found on multiple types of immune cells that may have opposing functions, and the outcome of any specific intervention may be context dependent and thus vary on the basis of unknown factors. For example, interleukin-2 (IL-2) administered to enhance the function of effector T-cells can also expand a population of Tregs.15 Moreover, activating interventions (e.g., vaccines, cytokines, or agonist T-cell costimulatory antibodies) can induce counterregulatory mechanisms that limit effector T-cell expansion and function, and the tumor-specific T-cells must still overcome induced and constitutive immunosuppressive mechanisms in the tumor microenvironment. The latter factors likely explain the relative lack of efficacy for multiple cytokines and cancer vaccines developed since the early 1980s. In contrast, antibody antagonists of PD-1 or one of its ligands PD-L1 are capable of producing substantial and durable tumor regression in a subset of patients across many different malignancies.16,17,18,19,20 The remarkable activity of PD-1/PD-L1 antagonists confirms that many patients have ongoing T-cell responses against their cancers at the time of presentation with advanced disease, and highlights the importance of blocking negative regulation of T-cell responses to enable their antitumor function, particularly within the tumor microenvironment.
Currently, there are relatively few examples of effective anticancer immunotherapies that are T-cell independent or rely primarily on other components of the immune system. Natural killer (NK) cells, whose cytotoxic activity is controlled by activating and inhibitory receptors, may contribute to the graft-versus-leukemia effect when the recipient is lacking ligands for the donor killer-inhibitory receptors.21 Induced or passively transferred antibodies can produce antitumor activity by mediating antibody-dependent cellular cytotoxicity (ADCC) or complement-dependent cytolysis (CDC). ADCC is triggered by binding of the Fc portion of the antibody with the Fc receptor on NK cells or myeloid-derived cells. The ability to induce ADCC depends on the IgG isotype and also on the type of Fc receptor, which may be either activating or inhibitory.22 Preclinical studies and some clinical correlative data suggest that ADCC may play a role in the antitumor activity of antibodies such as trastuzumab and rituximab. Currently only one antibody, targeted to the ganglioside GD-2 (ch14.18), is presumed to produce its anticancer effect primarily by ADCC. It was effective when used in combination with IL-2 and granulocyte-macrophage colony-stimulating factor (GM-CSF) in neuroblastoma patients following autologous marrow transplant, suggesting that the antitumor activity mediated by ADCC would produce the greatest benefit in a minimal residual disease setting.23
III. TOXICITY AND MANAGEMENT OF TOXICITY
Excluding hypersensitivity reactions or antibody infusion-related events, the toxicities of immunotherapies can be classified as follows: cytokine-induced, for example, after administration of IFN or IL-2 or after T-cell expansion and activation in adoptive cellular therapy; autoimmune-type reactions, usually observed after administration of immune checkpoint inhibitors; and on-target effects, by recognition of passively transferred antibodies or cells of their intended targets on normal tissues. In addition to the indicated supportive care for symptoms, management of toxicity depends on the cause and expected time course for reversibility, and will often involve steroids.
Because of the broad applicability of immune checkpoint inhibitors, most physicians treating cancer patients will be required to manage the autoimmune-like reactions associated with their use. Currently, only anti-CTLA-4 and anti-PD-1 have been approved for cancer treatment indications around the world. Unlike cytokines, immune checkpoint inhibitors rarely produce acute adverse events following the infusion. When adverse events occur, almost any organ system can be affected, but most common are skin, gastrointestinal tract, liver, and endocrine glands.16,24,25,26 In a subset of patients, adverse events can occur in multiple organ systems, either concurrently or serially. Adverse events induced by anti-CTLA-4 are more frequent and can be more severe than by PD-1/PD-L1 antagonists. Within the dose range of 1 to 10 mg/kg, the toxicity of anti-CTLA-4 is dose-related; in contrast, for anti-PD-1 doses in the 0.3- to 10-mg/kg range, no clear dose-response relationship for toxicity is apparent.16,27 Preliminary data suggest that patients developing high-grade immune-related adverse events (irAEs) from anti-CTLA-4 can be treated safely with PD-1/PD-L1 antagonists once toxicities resolve to baseline.28 Because of the long half-life of the antibodies, combined effects could be observed if administered within a short interval of each other. Concurrent administration of 3 mg/kg of anti-CTLA-4 with 1 mg/kg of nivolumab produced a higher rate of grade III to IV irAEs compared to either agent alone, which in incidence and severity resembled the toxicity profile of ipilimumab administered alone at 10 mg/kg.29,30,31
Careful observation of patients, frequent communication between patients and medical staff, and prompt administration of steroids when indicated are necessary to prevent severe outcomes from irAEs. A few toxicities associated with immune checkpoint inhibitors may be life-threatening if not treated promptly (e.g., bowel perforation that results from ongoing colitis, or progressive pneumonitis). After the development of an irAE, an initial workup is required to exclude other potential causes, and supportive care measures should be initiated. Physicians must subsequently determine if and when to start steroids, the dose of steroids, route of administration, inpatient or outpatient management, and length of steroid therapy. In steroid-refractory toxicity, a second immunosuppressive agent may be
required, such as anti-tumor necrosis factor (TNF) or mycophenolate mofetil. Algorithms have been developed and published for management of each of the most common irAEs.
required, such as anti-tumor necrosis factor (TNF) or mycophenolate mofetil. Algorithms have been developed and published for management of each of the most common irAEs.
For severe toxicities, including grade III to IV colitis or diarrhea, high-dose steroids equivalent to 2 mg/kg of IV solumedrol are administered for approximately a week, and then tapered slowly over approximately 30 days. Although the management algorithms are useful, clinical judgment is still required, for example, in assessing the rate of improvement and deciding if escalation of measures is required. If symptoms worsen or the rate of improvement is too slow on the starting dose of steroids, options include administering even higher doses of steroids, in some cases up to a gram of solumedrol IV daily, or initiating treatment with a second immunosuppressive agent. Recurrence of symptoms during the steroid taper can be managed by re-escalation of the steroid dose until resolution of the irAE, followed by another period of steroid dose taper, or administration of a second immunosuppressive agent. Because of the potential liver toxicity of anti-TNF agents, mycophenolate is used as the second-line immunosuppressive for liver irAEs.
For prolonged immunosuppression exceeding 4 to 6 weeks, administration of prophylactic antibiotics to prevent opportunistic infection should be considered. With few exceptions such as the endocrinopathies, irAEs fully reverse over time. Administration of steroids or other immunosuppressive agents to prevent onset of toxicity would be expected to counteract the antitumor effect of immune checkpoint inhibitors. However, current data suggest that use of steroids or other immunosuppressive agents to manage an adverse event does not substantially affect tumor response or duration of response.
In contrast to the immune checkpoint inhibitors, toxicities induced by cytokines or cytokines combined with cell therapies are generally managed with intensive supportive care, and steroids are administered only if the complications are immediately life-threatening.32 The toxicities are almost always rapidly reversible over hours to days. Administration of T-cells modified with a chimeric antigen receptor targeting CD19 on B-cells produced severe toxicity during the period of T-cell activation and expansion. Studies correlated the severe toxicity to induced cytokines and particularly to high circulating levels of IL-6. Toxicity was reversed rapidly by administration of an anti-cytokine against IL-6 without affecting antitumor response.33
IV. RESPONSE KINETICS
Studies of cytokines such as IL-2 provided strong proof of concept that immunotherapies could produce regression of advanced and large-volume metastatic disease.34,35,36 Moreover, in a subset of patients, complete regressions persisted for years without relapse, indicating that responses were durable and possibly curative. For cytokines such as IL-2, which is given weeks 1 and 3 in cycles of 8 to 12 weeks, tumor
regression was usually observed at the 8- to 12-week staging scans. Most patients with evidence of tumor regression received a second “cycle” of treatment, and rarely a third. Regression could continue beyond the end of treatment, but near-maximal tumor regression was often observed on staging scans obtained 5 to 9 weeks after the second cycle. Most patients with stable disease at the end of the first cycle did not go on to achieve complete responses with additional cycles of treatment. The majority of partial responses were short-lived, and occasional mixed responses were observed, but there are no comprehensive data on outcome for treatment continued beyond mixed response. The benefit, if any, of cytokine-induced mixed or partial responses or stable disease cannot be determined. However, a small subset of patients experienced long-term disease-free survival after surgical removal of a residual, recurrent, or discordant progressing lesion following substantial regression of multiple other sites of disease.
regression was usually observed at the 8- to 12-week staging scans. Most patients with evidence of tumor regression received a second “cycle” of treatment, and rarely a third. Regression could continue beyond the end of treatment, but near-maximal tumor regression was often observed on staging scans obtained 5 to 9 weeks after the second cycle. Most patients with stable disease at the end of the first cycle did not go on to achieve complete responses with additional cycles of treatment. The majority of partial responses were short-lived, and occasional mixed responses were observed, but there are no comprehensive data on outcome for treatment continued beyond mixed response. The benefit, if any, of cytokine-induced mixed or partial responses or stable disease cannot be determined. However, a small subset of patients experienced long-term disease-free survival after surgical removal of a residual, recurrent, or discordant progressing lesion following substantial regression of multiple other sites of disease.
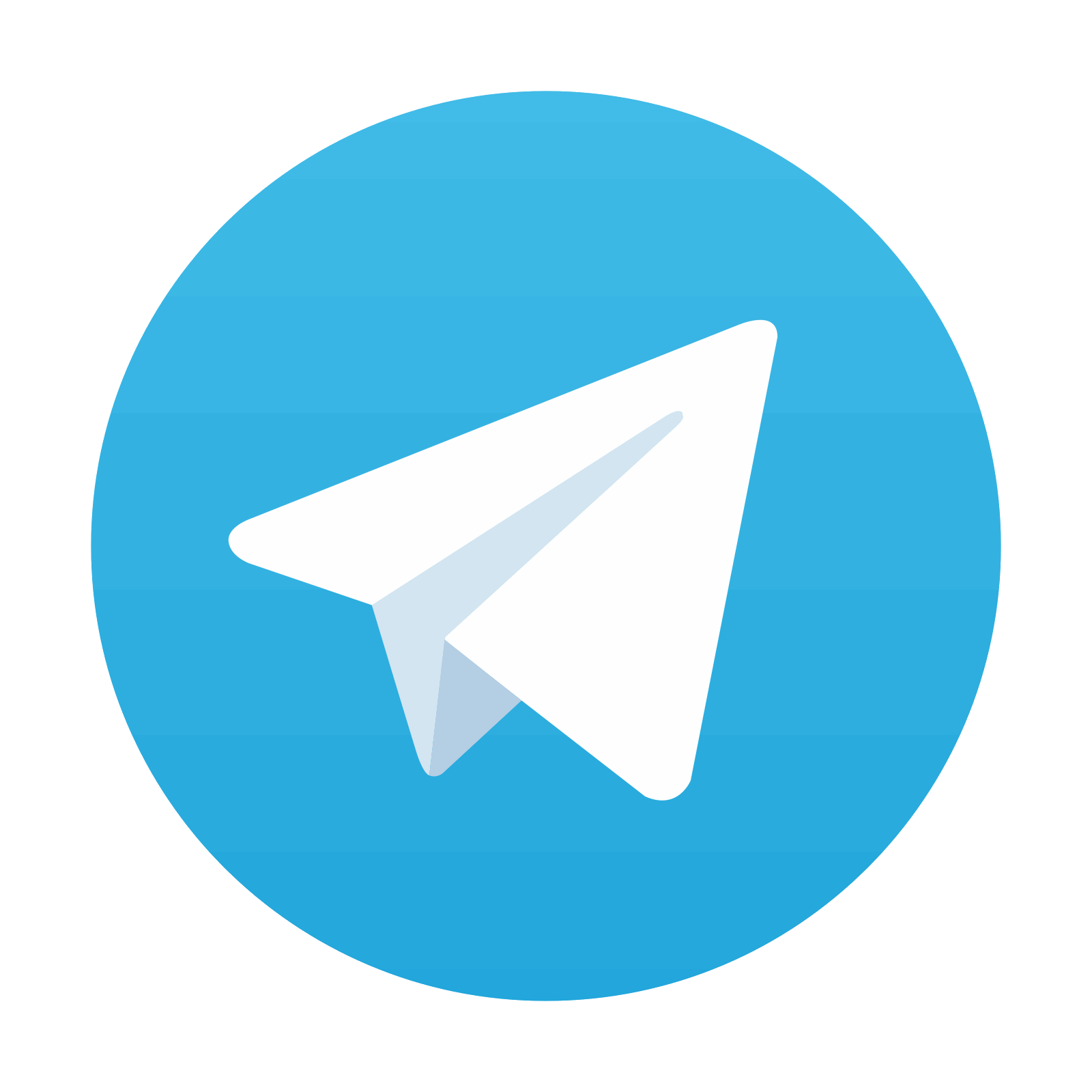
Stay updated, free articles. Join our Telegram channel

Full access? Get Clinical Tree
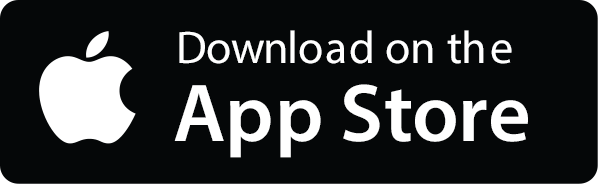
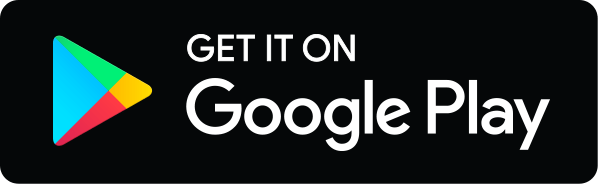