Chapter Outline
PERITRANSPLANT SUPPORTIVE CARE
ORGAN TOXICITY ASSOCIATED WITH HEMATOPOIETIC STEM CELL TRANSPLANTATION
CLINICAL APPLICATIONS OF HEMATOPOIETIC STEM CELL TRANSPLANTATION
Hematopoietic stem cell transplantation (HSCT) is an accepted treatment for a wide variety of diseases afflicting children, including hematologic malignancies, bone marrow failure syndromes, immunodeficiency disorders, congenital hematologic defects, and select solid tumors. HSCT generally involves a conditioning phase with administration of chemotherapy with or without radiation therapy followed by the infusion of autologous (self) or allogeneic (other) hematopoietic stem cells (HSCs). The conditioning damages the patient’s immune system, allowing engraftment of the new stem cells, and, in malignant diseases, can eradicate residual tumor cells, but at the cost of normal organ injury. Patients are profoundly immunocompromised as a result and are at risk for opportunistic infections. These infectious risks, together with other transplant-related complications such as graft rejection and graft-versus-host disease (GVHD) ( Box 8-1 ), and primary disease recurrence have been the main barriers to success in HSCT. However, advances in understanding transplant biology coupled with advances in the supportive care and clinical management of HSCT recipients have made this approach an increasingly attractive modality for a broadening range of indications.
Short Term
Graft rejection
Infection
Bleeding
Acute graft-versus-host disease
Veno-occlusive disease of the liver
Idiopathic pneumonitis
Side effects of radiation therapy, chemotherapy, and immunosuppressive therapy (e.g., radiation nephritis, azotemia)
Long Term
Late graft failure
Chronic graft-versus-host disease and its sequelae (e.g., bronchiolitis obliterans)
Pulmonary disorders
Infection
Altered intellectual and growth development in children
Reduced stamina
Endocrine dysfunction
Hypothyroidism
Growth retardation (children)
Pubertal delay; gonadal failure
Sexual dysfunction (both sexes)
Complications secondary to radiation therapy (e.g., cataracts, radiation nephritis)
Complications secondary to immunosuppressive therapy (e.g., aseptic necrosis of bone, hemolytic uremic syndrome)
Dental problems
Psychosocial problems
Increased risk for second malignancies
History and Overview
Infusion of bone marrow in an effort to restore hematopoiesis was first reported in 1939 when a patient with aplastic anemia was treated by infusion of a small aliquot of fraternal bone marrow. Subsequently, Jacobson and colleagues demonstrated that shielding the spleen allowed lethally irradiated mice to recover normal hematopoiesis. Later, it became clear that the hematopoietic reconstitution of irradiated animals originated from elements in the bone marrow or spleen and that these HSCs could survive cryopreservation and thawing. Investigators subsequently demonstrated that animals could recover only when histocompatible HSCs were infused but suffered from lethal GVHD if given cells from a histoincompatible donor. Better understanding of the essential mediators of the immune response coupled with the observation that administration of methotrexate was effective both in the prophylaxis and treatment of GVHD provided the theoretical and practical tools necessary for HSCT to be attempted in humans.
The first semi-successful attempt at a human bone marrow transplant (BMT) was carried out in 1957 by E. Donnall Thomas, whose body of work in this area was later recognized by a Nobel Prize. These first experiments demonstrated that chemotherapy followed by intravenous marrow infusion could result in a transient graft, although all patients subsequently died of progressive disease. In 1959, lethal doses of total-body irradiation (TBI) and bone marrow from an identical twin were used to in an attempt at transplant in two patients with advanced acute lymphoblastic leukemia. Hematopoiesis was established within weeks, although again both patients experienced relapse and died. The first successful allogeneic HSCT were performed in 1968 and 1969 with the survival of three patients who underwent transplants for congenital immunodeficiencies.
HSCT is currently used in a variety of malignant and nonmalignant disorders in which replacement of HSC-derived populations of cells provides or restores normal hematopoiesis or other marrow-derived elements. In malignant diseases, infusion of previously collected autologous HSC significantly shortens the period of myelosuppression and allows for dose escalation as an approach to decreasing relapse rates in malignant diseases. Although bone marrow was initially considered the only source of pluripotent HSC, more recently it has become clear that other sources exist. For example, self-renewing HSC can be collected from umbilical cord blood (UCB). HSCs also circulate in the peripheral blood, albeit in very low numbers; they can be increased after chemotherapy or administration of hematopoietic growth factors and collected as mobilized peripheral blood stem cells (PBSCs). Exploration of the potential for cell populations derived from tissues such as liver, nervous system, and muscle to provide HSCs has been initiated.
Reinfusion of a patient’s own HSCs after administration of high-dose chemotherapy, radiation therapy, or both is referred to as autologous HSCT. Both tumor contamination and preexisting damage to HSC from prior therapy limit its application. Moreover, the lack of allogeneic immunologic response against any residual or recurrent tumor further impacts success. Despite these limitations, autologous HSCT remains the standard approach to patients with refractory or recurrent lymphoma and other select solid tumors of transplant that demonstrate a response to dose escalation of chemotherapy. In the case of congenital conditions or acquired bone marrow failure syndromes, the abnormalities of the HSCs prevent autologous HSCT from being a meaningful option for most patients. Allogeneic HSCT involves the infusion of HSCs from a related donor (RD) or an unrelated donor (UD). Complications of allogeneic HSCT, such as graft rejection and GVHD, are generally more common or severe with increasing histoincompatibility between donor and host; and a histocompatible sibling, if available, remains the preferred donor. However, it is estimated that only 15% to 40% of patients will have a matched RD. Available alternatives include HSCT from an RD that is mismatched or from donors in the international UD registries, including previously collected and cryopreserved unrelated UCB.
Specific conditioning regimens used to prepare patients are reviewed in the next section. In brief, the preparative regimen must reduce or eliminate tumor burden and/or recipient hematopoietic cells and provide sufficient immunosuppression to permit engraftment. Reduced-intensity regimens were developed in the late 1990s to decrease acute transplant toxicity and thus make HSCT possible for a broader demographic. These regimens use intense immunosuppression and harness graft-versus-tumor effect instead of cytotoxicity to address residual malignant cells. Early and late graft failure and relapse remain problematic in this setting. After conditioning, HSCs are infused intravenously into the host; the day of HSC infusion is termed day 0. Neutrophil engraftment occurs 10 to 24 days after infusion, with red blood cell and platelet recovery somewhat more delayed. A number of peritransplant and posttransplant complications influence not only survival but also quality of life after transplant. There is a risk for early graft rejection and for late graft failure. Early complications of HSCT are due to profound pancytopenia, regimen-related toxicity, immunologic reaction of the graft against host tissues (acute GVHD) if the transplant is allogeneic, and protracted immune incompetence. Late complications of HSCT are due to chronic end organ damage from drug and immune insults, ongoing or de novo manifestations of immune dysregulation such as poor immune function or chronic GVHD, and the consequences of disease recurrence. These factors and complications are considered in detail later in this chapter.
Given these obstacles, the evolution of HSCT into a practical and curative therapy has been dependent on a number of important advances. These include an improved appreciation of the human histocompatibility system and development of more exact methods to establish the degree of histocompatibility between the donor and recipient. The ability to deliver the preparative regimen with greater accuracy, as illustrated both by improvements in TBI dosimetry and by the ability to monitor busulfan pharmacokinetics, have contributed to a decrease in regimen-related toxicity. Early and aggressive use of antibiotics, antifungal agents, and antiviral agents and more sophisticated screening for viral reactivation have improved survival during the time of neutropenia and the period of profound and persistent immunosuppression after engraftment. Advances in transfusion support, including viral screening and irradiation of blood products and availability of leukopheresed platelet products, have had enormous impact. Nutritional and other supportive therapies, including the ability to establish long-term vascular access with indwelling lines significantly changed the experience and comfort of HSCT recipients. Although HSCT was originally offered to a small number of patients who had no alternative therapeutic option, improved outcomes due to these and other advances have markedly increased the indications for which populations for whom HSCT can provide benefit. Analysis of registry data suggests that the lifetime probability of undergoing HSCT in the population may reach almost 1% for currently accepted indications if universal donor availability and an increase in the number of eligible patients who actually underwent transplant were to occur. Indeed, given ongoing scientific advances the indications for HSCT and cellular therapies such as gene therapy that utilize a backbone of HSCT are predicted to expand. The increasing population of HSCT patients and HSCT long-term survivors will be best served by improved infrastructure and research into lessening the burden of long term effects.
Conditioning Regimens
The term conditioning regimen refers to the preparative drugs and/or radiation that are administered to an HSCT recipient before the graft is infused. The purpose of conditioning is threefold: first, in the setting of HSCT for malignant conditions, to eradicate any residual malignant cells; second, to be sufficiently immunosuppressive to prevent the immune system of the recipient from rejecting the incoming allogeneic cells; and third, to induce enough marrow aplasia that the donor HSCs have a competitive advantage in reconstituting hematopoiesis. The regimens that are capable of achieving all three goals are usually associated with significant toxicity. Therefore a major goal in HSCT is the development of conditioning regimens that provide an optimal balance between effective myeloablation and manageable toxicity.
The first human BMT by Thomas and colleagues in 1957, and a subsequent experience using UD marrow to treat victims of a radiation accident in Belgrade in 1959, established the basic principle of TBI-based conditioning. Work initiated by Santos, Owens, and Sensenbrenner in the 1960s first demonstrated the use of cyclophosphamide as a conditioning agent. TBI combined with cyclophosphamide has remained the standard conditioning regimen for the past 30 years. More recently, the alkylating agent busulfan has become more widely used as an alternative to TBI, in part because of limited access to costly radiation facilities.
The wide range of indications for HSCT has led to considerable variation in the design of conditioning regimens. For example, high relapse rates after HSCT stimulated attempts to increase antileukemic efficacy of conditioning regimens by employing other high-dose chemotherapy agents in place of or in addition to cyclophosphamide. In contrast, the problem of graft failure/rejection in patients receiving grafts that are T cell depleted or are from genetically disparate donors has led to regimens that increase recipient immunosuppression. Patients with aplastic anemia or with severe combined immunodeficiency (SCID) have preexisting marrow aplasia or immunosuppression, and thus conditioning regimens in these diseases do not require the same degree of ablation as regimens for patients with intact immune function. Similarly, patients with benign hematologic diseases have no need of the antileukemic effect of conditioning, and thus specialized regimens have been developed specifically for these diseases. In addition, as autologous HSC support after high-dose chemotherapy became more common, many new conditioning approaches were developed, emphasizing maximal antitumor effects. Although individual studies demonstrated acceptable or even favorable outcomes related to some of these changes, no consensus as to optimal recipient preparation for either autologous or allogeneic SCT has emerged. More recently, alternative concepts regarding the relative roles of conditioning regimens and the donor immune system in achieving recipient hematopoietic ablation have been applied in a nonmyeloablative approach. Some key issues are highlighted in the next sections.
Total-Body Irradiation
Several parameters associated with TBI can be adjusted to deliver different amounts of radiation and different degrees of end organ effect. Total dose, dose rate, fraction size, interfraction interval, and shielding are among the TBI parameters most manipulated in SCT. An ideal schedule would maximize malignant cell kill, hematopoietic ablation, and, in the case of allogeneic SCT, immunosuppression while limiting acute and chronic toxicity. In principle, higher total dose, higher dose rate, and larger fraction size are associated with greater hematopoietic ablative effect (and therefore potentially greater antileukemic efficacy) and with better immunosuppression. Fractionation, the division of the total dose of radiation over time usually in a twice-daily schedule, theoretically provides improved tolerance for nonhematopoietic tissues, leading to decreased acute toxicity and reduced late effects, but carries the risk for decreased antileukemic efficacy. However, a series of studies from the 1980s supports the use of fractionated radiation, and this remains the standard today. Studies from Seattle have demonstrated increased antileukemic efficacy with increased total dose, but lung, liver, and kidney tolerances were limiting. Parameters of total dose, fractionation, dose rate, and schedule have been largely extrapolated to pediatric HSCT from adults.
Busulfan
Developed in the mid-1970s, busulfan (BU)/cyclophosphamide (CY) rapidly became an established regimen for patients with acute myelogenous leukemia (AML) receiving either allogeneic or autologous SCT. The original regimen, called BU/CY4 (“big BU/CY,” BU 4 mg/kg/day × 4 days followed by CY 50 mg/kg/day × 4 days) was joined by BU/CY2 (“little BU/CY,” in which the CY was delivered as 60 mg/kg/day × 2 days), and this regimen has been used in patients with acute lymphocytic leukemia (ALL) or chronic myelogenous leukemia (CML). The BU/CY regimen has been used extensively in pediatric patients, particularly those with nonmalignant diseases, in an attempt to avoid radiation-associated toxicity. The current practice of using an intravenous formulation of BU and dose adjustment to achieve a target level has decreased toxicity; effects on efficacy are more difficult to demonstrate. Treosulfan, a related agent already approved in Europe, has limited nonhematologic toxicity, and pediatric trials are ongoing in national trials.
TBI versus BU
Several randomized studies and meta-analyses have compared BU/CY with TBI-based regimens. Overall, results are similar although suggestive that both relapse rates and hepatic veno-occlusive disease (VOD) may be less in the TBI-containing regimens. Although recent data support these observations, variability of the chemotherapy component of TBI-based regimens and potential differences in the antileukemic efficacy of BU/CY4 and BU/CY2 regimens render definitive generalizations difficult. Reports of decreased bioavailability, increased volume of distribution, and increased clearance rate of BU in children raise the question of how to interpret studies in which such data are unavailable. Alternative BU dosing, based on plasma levels, has been suggested, and comparison of “optimal” BU/CY with CY/TBI has not been reported. BU-containing regimens have often been reported to result in greater toxicity when compared with TBI-based regimens; thus increases in BU dose may further increase regimen-related morbidity. Monitoring of BU pharmacokinetics has generally, but not always, demonstrated an association of increased area under the curve with increased volume of distribution. Individualizing BU dosing may possibly increase the risk-benefit ratio, and the recent availability of an intravenous form of BU with more dependable pharmacokinetics now makes this achievable.
Alternative Myeloablative Regimens
The addition or substitution of a variety of chemotherapeutic agents to the backbone of CY/TBI, and less commonly BU/CY, has met with mixed success. Etoposide, cytarabine, and BU have been added to or substituted for CY in TBI-based allogeneic regimens. Etoposide (VP-16) and melphalan have also replaced CY in some BU-based protocols. Clofarabine, a purine nucleoside analogue, is a relatively new drug known to be very effective in relapsed/refractory pediatric ALL; ongoing studies have shown promise in using it in combination with BU to decrease relapse in advanced cases of pediatric leukemia. Fludarabine has become an increasingly used drug in allogeneic transplant preparative regimens. It has potent immunosuppressive properties and minimally extramedullary toxicity. Combined with BU (BU/FLU) it provides adequate ablation for full donor engraftment, and in the pediatric population it appears to have similar efficacy but less transplant-related toxicity and faster neutrophil engraftment.
Fluorouracil, high-dose methotrexate, doxorubicin, cisplatin, carboplatin, thiotepa, melphalan, and many other agents have been used in a variety of high-dose chemotherapeutic regimens supported by autologous SCT. Common conditioning regimens referred to by acronyms are CBV (CY, carmustine, VP-16); BACT (BCNU [carmustine], cytarabine [Ara-C], CY, 6-thioguanine); BEAM (BCNU, etoposide, cytarabine [Ara-C], melphalan); and CBP (CY, BCNU, cisplatin). In the allogeneic setting, alternative regimens have generally shown equivalent benefit in terms of disease control but often have increased toxicity. No regimen has established unequivocal superiority in terms of disease-free or overall survival when compared with “standard regimens.” Given the more limited follow-up and the extraordinary diversity of diseases and regimens, establishment of relative efficacy in the realm of autologous SCT is likely to be a prolonged process.
Nonmyeloablative Regimens
One of the target tissues of allogeneic T lymphocytes is the hematopoietic precursors. As a result, the infusion of donor lymphocytes can be sufficient to cause marrow aplasia. Indeed this is the basis of the marrow aplasia seen in inadvertent cases of transfusional GVHD. Observations made initially in the 1980s by the Seattle group have led to a new approach to conditioning based on the immunologic elimination of normal and malignant hematopoiesis. Because this technique minimizes the doses of conventional conditioning drugs and radiation, it has been called “nonmyeloablative” or reduced-intensity conditioning. Although allogeneic graft-versus–bone marrow can cause the necessary marrow aplasia and antileukemic efficacy, it does not supply the immunosuppression needed to avoid graft rejection. This is usually achieved by preinfusion conditioning with purine-analogue chemotherapy and/or low-dose TBI. A variety of agents including lower-dose BU, melphalan, and cytarabine have also been used for additional effect and partial HSC depletion. A major advantage of this approach is that the toxicity of conventional myeloablative approaches can be reduced. Comparisons of conventional versus nonmyeloablative regimens have shown reduced nonrelapse mortality, especially in older adults or patients with preexisting illnesses for whom HSCT is associated with significant toxic death rates. The safety and effectiveness of this approach has led to its wider application in recent years. However, the low toxicity of nonmyeloablative regimens is balanced against the increased risk for relapse in acute leukemias and the increased risk for chronic GVHD. The indications for nonmyeloablative transplant may therefore remain principally for slower-growing tumors. Because childhood hematologic malignancies rarely fall into this category, the use of nonmyeloablative transplant in children has been more limited. Moreover, the increase rates of graft rejection after standard HSCT for nonmalignant conditions such as thalassemia and sickle cell anemia have precluded the broad use of nonmyeloablative transplants in these conditions.
Sources of Stem Cells
Selection of an appropriate source of HSCs for the patient undergoing HSCT is a complex undertaking. HSCs can be obtained from the bone marrow, peripheral blood, or the umbilical vein of neonates. The majority of HSCTs in the pediatric setting are performed from allogeneic donors, but autologous peripheral blood is also used as a stem cell source for transplants, particularly in patients with solid tumors. For each disease entity, the clinician must consider what sources of stem cells are available and the relative risks and benefits associated with each potential stem cell source. For allogeneic donors, the principal consideration in donor selection is the genetic similarity between donor and recipient.
Typing
Genetic disparity between host and donor can lead to graft rejection or GVHD. The genes encoding the antigens most associated with these complications lie in a group of genes known as the major histocompatibility complex (MHC). In humans, the MHC maps to a region of the short arm of chromosome 6, known as the human leukocyte antigen (HLA) system. The MHC codes for many genes are still being identified, and the exploration of the functional importance of some of these gene products is still ongoing. Among the best characterized gene products are proteins comprising the class I and class II antigens. In humans, MHC class I molecules include HLA-A, HLA-B, and HLA-C and MHC class II molecules are called HLA-D. There are at least five subregions, including DR, DQ, and DP, but the number of genes transcribed differs between different HLA class II haplotypes.
The MHC class I antigens are present on nearly all human cells (with rare exceptions such as the erythrocyte and corneal endothelium). MHC class II antigen expression is more restricted, with expression limited to “professional” antigen-presenting cells, including dendritic cells, B cells, monocytes, macrophages, and Langerhans cells. Class II antigens can also be induced on activated T cells and endothelium. MHC genes are codominantly expressed, that is, each parent contributes half of the MHC antigens expressed on each cell of their offspring. MHC class I and II antigens normally function to present partially degraded products of intracellular proteins to T cells. In this way, damaged or virally infected cells can be identified by the immune system. However, in the setting of transplantation, the recognition of MHC molecules by T cells is known as alloimmunity and is the basis for GVHD and graft rejection.
The genes of the MHC region are highly polymorphic, and less than 30% of potential recipients of HSCs have HLA-identical siblings. Moreover, even HLA-identical sibling transplants can be associated with GVHD because of differences in the sequence of the intracellular proteins associated with MHC molecules caused by polymorphisms in non-MHC genes. These polymorphisms are mostly unidentified, and those that are known to elicit alloreactive T-cell responses are termed minor histocompatibility antigens. Although these antigens may not be directly responsible for organ and bone marrow rejection, human typing studies suggest that these minor antigens contribute to the generation of GVHD.
Historically, HLA typing was performed by using antibodies derived from postpartum sera, from persons who have undergone transplants or transfusions, or even from persons immunized for the purpose of generating specific serologic reagents. These antibodies were used in a standardized complement-dependent, microcytotoxicity assay with purified T or B lymphocytes as their target cell. The extensive cross-reactivity among products coded by the alleles of the HLA-A and HLA-B loci, known as cross-reactive groups, made precise delineation of the MHC class I antigens by this technique difficult. In contrast, HLA-D specificities were originally defined by their ability to stimulate T-lymphocyte proliferation in a mixed leukocyte culture.
Because of the difficulty in precise delineation of HLA-type by serologic methods, modern HLA typing now uses high-resolution techniques that resolve the DNA sequence of specific alleles. The first attempts to use molecular genetic techniques were based on application of restriction fragment length polymorphism analysis of genomic DNA by Southern blotting using HLA region probes. This soon gave way to current technology in which sequence-specific oligonucleotide probes and, in some cases, direct sequence analysis are performed. This has also led to a great increase in the number of identified HLA specificities. This fine analysis is increasingly being used to determine the haplotypes of patients and potential donors. The implications of more exact typing within families, where the haplotypes are “conserved,” are probably confined to the potential identification of minor histocompatibility antigen differences, allowing for better selection between possible donors. In the UD setting, high-resolution HLA matching has significantly contributed to the improved outcomes reported in UD HSCT in the past decade. Historically, an allogeneic donor must match the patient at five of the six major loci (e.g., A, B, DR) for a transplant to be feasible. Stem cells obtained from UCB are more permissive, and four of six matches are acceptable.
Allogeneic Stem Cell Transplantation
Most allogeneic transplants use HSCs from a matched sibling donor. However, with improvements in immunosuppression and ability to prevent or treat GVHD, UDs, UCB, and partially MHC-mismatched family donors are now increasingly used as sources of HSCs. There are now approximately 16 million UDs listed in over 72 registries worldwide, including registries of banked UD UCB collections.
The selection of an allogeneic HSC source is dependent on many variables. In malignant disease, the ease and speed with which a stem cell source can be obtained are important factors. For patients with aggressive malignancies, being able to proceed rapidly to transplant is particularly desirable. Increasing genetic disparity between donor and host—termed histoincompatibility— has historically contributed to both an increased rate of graft rejection and severe acute and chronic GVHD and is a critical factor in the choice of donor. Even in the setting of HLA-identical siblings, selection can be further refined according to donor gender and age, history of donor parity, donor infectious disease status, and other variables, all of which can contribute to the success of transplant.
For the majority of patients who do not have a matched sibling donor, potential sources include a family member who is phenotypically adequately matched at the HLA loci but is genotypically distinct or a volunteer UD donor who shares the majority of HLA alleles with the recipient. The likelihood of locating an appropriate UD donor is dependent both on the patient’s ethnicity (in as much as it determines histocompatibility antigen and haplotype frequency) and on the composition of the donor pool. For example, for whites of mid- and northern European descent the likelihood of finding a six-antigen matched UD approaches 50% to 70%. In contrast, the extreme genetic heterogeneity of the African-American population coupled with underrepresentation of this population in volunteer registries makes the likelihood of finding a similar matched donor 10% to 15%. Molecular typing techniques demonstrate that the allelic disparities in both whites and nonwhites is much higher than previously expected, thus making the ascertainment of truly “matched” UD less likely even as the donor pool expands. Although the implications of specific HLA mismatches are incompletely understood, most studies suggest that high-resolution HLA matching is associated with improved outcome in UD HSCT. Thus additional strategies to broaden the donor base by using alternative HSCs such as UCB that exhibit different biologic properties and allow greater HLA disparity have allowed for more patients with uncommon typing to find an acceptable donor.
Sources of HSC
In the past, most HSCT has used bone marrow mononuclear cells as a source of HSCs. Bone marrow is usually obtained by repeated aspiration of the posterior iliac crests while the donor is under general anesthesia. Multiple aspirations are performed to a total volume of 10 to 15 mL/kg recipient body weight. The marrow is filtered through a fine wire mesh to rid the product of aggregates and bony spicules. This is a surprisingly well-tolerated procedure and serious complications are rare, probably because the donor is generally a healthy individual undergoing an elective procedure. Potential short- and long-term complications include risks of anesthesia, blood loss and potential transfusion, pain, neurologic deficits, and psychosocial complications. In the most truly altruistic setting, UD marrow harvest, only 5% to 8% of donors later expressed any ambivalence about their participation. This was most marked if the transplant was subsequently unsuccessful. Once harvested, the red cells must be removed from the bone marrow if there is ABO incompatibility before infusion into the recipient. The marrow may be manipulated ex vivo to remove donor T lymphocytes (see later) or tumor cells. It is estimated that only 1% of the donor pluripotent HSC population is removed in a typical marrow harvest.
HSCs continuously recirculate from the marrow into the peripheral blood; therefore HSCs can also be harvested by collection of peripheral blood mononuclear cells, usually by leukapheresis. The frequency of cells that express the surface marker CD34 is widely used as a proxy for actual HSC frequency and allows the enumeration of stem cells. HSCs in the peripheral blood are rare, and granulocyte colony-stimulating factor (G-CSF) is usually administered to increased the detachment of HSCs from bone marrow niches and promote their egress into the peripheral blood, increasing their frequency. Moreover, studies in adults suggest that additional strategies to further mobilize marrow HSCs are possible. AMD3100 is a reversible inhibitor of the CXC chemokine receptor (CXCR4), a receptor on the surface of HSCs that promotes adhesion to the bone marrow milieu. Combining AMD3100 with G-CSF increases HSC yield in adults, but this approach has not been investigated in children. Complications of PBSC harvest have included bone pain, as a side effect of G-CSF, and inability to collect via peripheral access, necessitating central line placement. There is an increased risk for splenic rupture resulting from G-CSF administration in healthy individuals, although this is an extremely rare complication. The implications of mobilizing and harvesting PBSCs from pediatric donors have not been fully explored, although cytopenias and hemostatic changes of unknown short- and long-term significance have been observed in healthy donors.
Since the first description of its use in a child with Fanconi anemia in 1988, UCB has become an increasingly used HSC source. Placental blood is recovered from the umbilical vein by drainage or catheterization, and techniques to optimize UCB HSC collection are being investigated. Cord blood is immediately frozen and stored until needed. If indicated, in the related setting, determination of the HLA type of the fetus can be performed before delivery. Otherwise, typing and analysis is performed on intrapartum or postpartum samples.
Selection of a UD, whether bone marrow or UCB, is facilitated by the availability of epidemiologic information (e.g., age, sex) as well as HLA typing stored in a database accessible via the Internet. By comparing a patient’s HLA type with donors listed and categorized in the registries, one can estimate the likelihood of a successful search, devise search strategies for patients with uncommon HLA types, and sort among potential HSC sources. Although stored UCB can be readily supplied after confirmatory typing, the completion of a UD bone marrow donor search is more involved. Potential donors must be contacted and consent to proceed, and the donor center must arrange for appropriate confirmatory studies and medical and other evaluations and schedule the harvest. The time from formal search to BMT averages 4 months for UD, whereas compatible UCB, where typing occurs at time of cryopreservation, can be found in days to weeks.
Engraftment
After allogeneic HSCT, donor stem cells must expand to repopulate hematopoietic niches and differentiate to form mature blood cells. HSCT recipients show accelerated telomere shortening in peripheral blood cells, which is presumably due to increased replicative demand for donor stem cells. Donor hematopoietic engraftment after allogeneic HSCT can be documented both by analysis of chimerism as well as by the recovery of peripheral blood cell counts. However, sensitive DNA amplification technologies have demonstrated residual host hematopoiesis for variable periods of time, certainly up to 1 year. Mixed lymphoid chimerism is also well documented. The implications of mixed chimerism with respect to subsequent relapse remain controversial, probably because the current extremely sensitive assays generate data that are difficult to interpret uniformly and because outcome may well differ by disease type. For example, chimerism after BMT for CML is quite reliably related to subsequent relapse while this may not be true for other diseases.
The rate and probability of engraftment after allogeneic BMT varies with the source of HSCs, the number of HSCs given, the extent of preinfusion cell manipulation, and the GVHD prophylactic regimen used. In general, neutrophil recovery is achieved within 2 to 3 weeks and platelet recovery occurs 1 to 2 weeks thereafter. PBSC transplants are associated with a more rapid rate of engraftment than with bone marrow, most likely a result of the higher CD34+ cell dose obtained. Neutrophil engraftment occurs between 1 and 6 days earlier with PBSC transplant than with BMT and platelet engraftment between 4 and 7 days earlier. Neutrophil and platelet engraftment after UCB transplantation is significantly delayed in comparison with that seen in other stem cell sources. It is a consequence of the lower nucleated cell and CD34+ cell dose in UCB products. This has limited the application of this modality in adults, and currently using two unrelated UCB units for a given patient is being studied.
The use of methotrexate as GVHD prophylaxis is associated with delay in count recovery; conversely, recovery after T-cell–depleted BMT is more rapid. Use of colony-stimulating factors after BMT is associated with more rapid neutrophil recovery after related BMT, a finding confirmed in small pediatric series. No change in platelet recovery has been noted. Interestingly, preliminary data do not show any colony-stimulating factor–mediated acceleration of engraftment in patients undergoing UD BMT.
The number and quality of T cells that are present in grafts from peripheral blood, bone marrow, and UCB confer different rates of GVHD. GVHD, particularly chronic GVHD is more frequent in PBSC transplant than in bone marrow–derived HSCT, although this risk may be offset by an increased graft-versus-leukemia effect. In adults this increased rate of GVHD has not diminished the efficacy of PBSC transplant. However, in children, PBSC transplant has been associated with a worse outcome than when bone marrow is used as a cell source. The precise indications of PBSCs versus bone marrow as a source in pediatric HSCT therefore remains to be defined. Conversely, the rate of GVHD in UCB is lower than with either PBSC or bone marrow sources and allows for less stringent HLA-typing requirements.
Autologous Stem Cell Transplantation
The fundamental rationale supporting autologous HSCT is that very high doses of cytotoxic agents (dose intensification) increase tumor cell death and that a high-dose treatment regimen can be devised in which the major dose-limiting toxicity is myelosuppression. This toxicity can be ameliorated by infusion of previously collected and cryopreserved autologous stem cells obtained either by bone marrow harvest or by apheresis of peripheral blood. Using autologous cells to support and reconstitute the host after aggressive ablative or near-ablative therapy has been most thoroughly explored in both Hodgkin and non-Hodgkin lymphomas, less well developed in acute and chronic leukemias, and still considered experimental, albeit relatively widely applied, for a growing number of pediatric and adult solid tumors. As well, the relative merits and demerits of each autologous cells collected peripherally or from marrow are still not firmly established.
Contamination by Tumor Cells
A major limitation of autologous HSCT remains contamination of the stem cell product with residual malignant cells. Although the relative contributions of either residual tumor within the host or tumor reinfused with stem cells to subsequent relapse remain unknown, increasing evidence from gene-marking studies suggests that reinfused cells contribute to relapse. In addition, in vitro assays of tumor cell colony formation and minimal residual disease (MRD) detection by polymerase chain reaction suggest that contamination of the stem cell source by tumor cells is associated with an increased relapse rate after HSCT. No large randomized clinical trial of PBSC versus bone marrow with respect to disease-free survival (DFS) has been reported. However, paired samples of PBSCs and bone marrow have been examined in a variety of settings (e.g., breast cancer, non-Hodgkin lymphoma) in which PBSCs have revealed lower levels of tumor cell contamination in the steady-state setting. More recent data suggest that the advantage of PBSCs may be abrogated after mobilization, although impact on DFS remains unclear.
A variety of methodologies have been developed to eliminate, or purge, tumor cells from stem cell collections. These are based on either positive or negative selection. The major clinically applied positive selection strategy is that of stem cell enrichment, most often selection of CD34+ cells. Negative selection is based on technologies that deplete tumor cells to a greater degree than normal stem cells. Strategies include the use of pharmacologic agents (e.g., mafosfamide), immunologic reagents targeting tissue specific antigens (e.g., neural crest antigens in neuroblastoma), and manipulation of physical or culture properties of cells. Combinations of both negative and positive selection strategies may improve both the yield and purity of stem cell collections. Increasing evidence in multiple clinical settings demonstrates an association between minimal residual tumor contamination and relapse and, conversely, suggests that more effective purging strategies may be associated with improved DFS. However, randomized studies comparing purged and unpurged stem cells have yet to be reported.
Engraftment
Trilineage hematologic reconstitution has been achieved after the infusion of autologous bone marrow, after bone marrow and PBSCs, and after PBSC infusion alone. The most mature studies are of patients reconstituting hematopoiesis with autologous marrow where engraftment after bone marrow infusion is both reliable and durable; durability of PBSC-reconstituted hematopoiesis, undertaken more recently, is less fully evaluated. The best predictor of engraftment and rate of hematologic recovery has not been established. Although colony-forming units, burst-forming units-erythroid (BFU-E), nucleated cell count, and other measures have correlated with time to engraftment in some studies, correlations have not been universal and may additionally depend on the patient population, method of cell procurement, and subsequent cell manipulation. CD34+ counts have emerged as the most reliable and practical method for predicting engraftment after PBSC infusion, with retrospective analyses demonstrating that the risk for graft failure is substantially higher in patients who received less than 2 × 10 6 /kg CD34 + cells per kg.
Reconstitution of hematopoiesis after autologous bone marrow infusion has shown some tendency to be delayed when compared with the allogeneic setting. This delay is presumed due to the extent, nature, and duration of prior therapy. In addition, cell manipulation ex vivo (purging) may also contribute to delayed engraftment. Reconstitution after HSCT with autologous bone marrow can be hastened somewhat by the addition of hematopoietic growth factors to the post-SCT supportive care regimen, although the extent and nature of prior therapy may limit response to growth factors. Preliminary information in both the preclinical and clinical arenas suggests that prior aggressive chemotherapy may limit potential for hematologic recovery. Prior intensive chemotherapy supported by use of growth factors may also contribute to poor engraftment after autologous transplantation. In fact, despite recovery of peripheral blood counts, hematologic marrow reserves as assessed by in vitro measures of hematopoiesis may be blunted for many years after autologous HSCT.
Addition of mobilized PBSCs to bone marrow has resulted in a dramatic decrease in days of neutropenia and thrombocytopenia in most series with or without subsequent growth factor support. Further, use of mobilized PBSCs as the sole stem cell source has been almost universally associated with more rapid hematologic recovery. It is still debatable whether the use of hematopoietic growth factors in this setting further enhances the rate of engraftment, but available data suggest that it may. Whether the process of PBSC transplantation will result in durable hematopoiesis over decades is not yet evaluable, but reports of late graft failure are rare. Most studies suggest that the increased rate of engraftment observed with PBSCs results in decreased days in hospital, use of antibiotics, and associated supportive services. The overall impact of PBSCs versus bone marrow as a stem cell source on the outcome or cost of transplantation has yet to be determined.
Myelodysplasia Syndrome as a Late Complication
Chemotherapy, particularly with alkylating agents, may result in an increased risk for subsequent myelodysplasia syndrome (MDS). Many patients who come to autologous transplantation have received treatment with such agents. Over the past several years, increasing numbers of patients developing MDS after autologous transplantation have been reported, usually occurring from 4 to 7 years after HSCT. The actuarial incidence ranges from 5% to 10% in most series, although it has been reported to be as high as 18% at 5 to 6 years after HSCT in single center experiences. Identified risk factors have included etoposide use for stem cell mobilization, conditioning regimens utilizing TBI, and the amount of chemotherapy received before HSCT and/or the interval between diagnosis and HSCT. Whether the MDS results from previously damaged reinfused hematopoietic cells or from residual hematopoietic cells sustaining further injury during conditioning is unresolved. Cytogenetic analysis of bone marrow before autologous HSCT can reveal preexisting abnormalities and should be a standard component of pre-SCT evaluation. However, most patients developing MDS have been reported to demonstrate normal cytogenetics at the time of transplant. With more sensitive techniques currently available, such as fluorescence in situ hybridization, the majority of patients developing MDS post-SCT have been shown to harbor the same cytogenetic abnormality in pre-SCT specimens. Similarly, using an X-inactivation–based clonality assay in female patients, clonal hematopoiesis was demonstrated in a small percent of patients before HSCT; those patients were at significant risk for developing MDS after HSCT. This suggests that many cases of post-SCT MDS evolve from an abnormal clone already present before HSCT. The appropriate evaluation of hematopoietic status before embarking on autologous HSC collection and transplantation requires further study.
Immune Reconstitution
The period after HCST is characterized by profound immunodeficiency, and death from infectious complications is a major obstacle to the success of transplantation. Myeloablative conditioning results in the wholesale loss of immune cells that are necessary to confer protective immunity, including T, B, and natural killer (NK) cells. Although hematopoiesis can return within weeks of transplant, recovery of a functional immune system can take much longer and the risk for opportunistic infection remains high for up to a year even in uncomplicated transplants.
The degree of immunodeficiency varies with time, reflecting different contributions of donor and recipient immune function at different stages after transplant. Mature T, B, and NK cells are present in the donor inoculum of bone marrow, peripheral blood, or UCB and can contribute to some degree of host immunity in the immediate posttransplant period. The ability of these transferred cells to provide immune protection is dependent on how immunologically “experienced” the donor is. For instance, recipients of grafts from donors who are immune to cytomegalovirus (CMV), that is who have previously been infected with the virus and have mounted a protective immune response, have a lower rate of CMV disease, presumably because of transferred immunologic memory to that virus. Similarly, recipients whose donors have been vaccinated with a pneumococcal vaccine respond to pneumococcal vaccines more rapidly and with higher antibody titers after transplant, again suggesting that donor immunity can be transferred to the recipient. However, full immune reconstitution, with the ability to mount a protective immune response to new pathogenic challenges, is dependent on the differentiation of new immune cells from precursors in the donor graft inoculum.
In most patients, NK cells are the first lymphoid population to recover. In the first month after transplant, NK cells can represent the main lymphoid cell in the peripheral blood. T cells recover much more slowly, and normal numbers of CD4 and CD8 T cells are not achieved for 6 to 12 months after HSCT. The delay in T-cell neogenesis is because the development of new T cells is a complex process that takes months to complete. During the early period after transplant, T cells with a naïve phenotype predominate in the peripheral blood, suggesting repopulation of the T-cell compartment with recent thymic emigrants. Increased thymic output contributes to the expanding peripheral T-cell pool after transplant for 12 to 24 months after HSCT. This thymic output occurs even in adult patients whose thymuses have presumably involuted. The rate of increase in T cells is usually much more rapid in pediatric HSCT patients, consistent with the presence of more robust thymic function. The advantage of a functional thymus in immune reconstitution has been demonstrated by the marked decrease in the fraction of naïve peripheral blood T cells in a 15-year-old HSCT patient who had had a previous thymectomy compared with thymus-bearing HSCT patients. By using quantitative molecular measures of T-cell repertoire, several studies have shown that it can take as long as 12 to 24 months after HSCT for a full range of T-cell receptor diversity to be present in the T-cell compartment. This underscores the critical role of naïve thymic emigrants in restoring the full complement of T-cell receptor specificities.
Several factors influence the length of time that immunologic recovery takes. Age of BMT recipients profoundly affects the rate of immune reconstitution, with an inverse correlation between recipient age and the absolute number of T cells 1 year after HSCT. Using mobilized PBSCs as a source of stem cells appears to be associated with a faster rate of recovery than using HSCs from bone marrow, possibly as a result of a larger cell dose. Increasingly intensive conditioning regimens are delaying immune recovery in autologous recipients to a degree comparable with allogeneic HSCT. Although hematopoietic engraftment appears to be delayed in UCB recipients, immune reconstitution in children after UCB HSCT is comparable to those receiving unrelated BMT. The presence of GVHD can significantly delay immune reconstitution both as a result of disruption of normal T-cell development and from the added immunosuppression that is required for management of the disease. The consequence of factors that delay immunologic recovery is significant: slow recovery of immune function is associated with an increase in the cumulative incidence of infections and nonrelapse mortality.
Peritransplant Supportive Care
Transfusion Support
Virtually all patients undergoing HSCT require red blood cell and platelet transfusions during transplant, and some patients require transfusions for several months after transplant. All blood products should be gamma irradiated to prevent transfusion-induced GVHD that can result from donor T lymphocytes in the cellular product. Multiple processes including psoralens photochemical treatment and leukocyte filtering have been investigated for prevention of transfusion-associated GVHD, but gamma irradiation remains the standard of care. Additionally, it is recommended that blood products are either filtered to achieve white blood cell reduction or CMV seronegative. Prior studies indicated that leukocyte-reduced blood products are equivalent to CMV-seronegative blood products for the prevention of transfusion-transmitted CMV. However, a meta-analysis of the available literature suggests that the risk for transfusion-transmitted CMV is greater with white blood cell–reduced blood products when compared with CMV-seronegative products.
Whether platelet and red blood cell transfusions should be given prophylactically during the period of cytopenia remains an area of controversy. There are no studies in the modern transplant era evaluating the use of prophylactic platelet transfusion compared with therapeutic transfusions. More recent studies have addressed different therapeutic triggers for prophylactic transfusions, specifically 10 ×10 9 /L versus 30 or 20 × 10 9 /L. A review of studies of prophylactic platelet transfusion triggers found no statistically significant differences between groups with regard to mortality or severe bleeding events. Although no statistical significance was found, these studies were small and no recommendations can supplant the need for clinical judgment in individual situations.
Nutrition Support
Provision of nutritional support during HSCT requires an appreciation of the metabolic needs of the individual patient, which is influenced by the body composition and complications of transplant. Patients who are underweight have been shown to be at an increased risk for death compared with patients of normal weight in the early period after transplant. The impact of obesity on transplant outcome is debated, with some studies noting no effect and others reporting lower survival in obese patients. Both the direct effects of conditioning regimens (e.g., mucositis) and complications of HSCT (e.g., GVHD, VOD, infection) can induce gastrointestinal damage that compromises the nutritional status of HSCT patients. Enteral feeding has been shown to be effective and safe and to have fewer complications than parenteral nutrition during HSCT but is not always practical for children. Several studies have demonstrated the efficacy of parenteral nutrition when enteral nutrition is no longer possible. However, in addition to expense, parenteral nutrition has attendant potential complications, including vascular access difficulties, abnormal liver function, and episodes of hyperglycemia and hyperlipidemia. A review of literature does not support the uniform use of parenteral nutrition as nutritional support during HSCT. The authors propose that the use of parenteral nutrition be reserved for patients unable to tolerate enteral feeding. Some patients may be able to maintain their nutritional needs orally throughout transplant, and both enteral and parenteral nutrition routes may be used on occasion. Resumption of enteral feeding should be encouraged, and continued monitoring of nutritional intake and weight is necessary not only through engraftment but also after discharge from the hospital. Malnutrition appears to be relatively frequent after HSCT, and even patients with no or limited GVHD may have significant nutritional problems.
Infection Prophylaxis and Treatment
Infection risk is greatest in the allogeneic and T-cell–depleted settings, where, despite phenotypically normal circulating lymphocytes, cellular immunity is depressed for at least 1 year after HSCT. Guidelines for infection prophylaxis and treatment are published regularly by the American Society of Blood and Marrow Transplantation and provide a standard approach to an ever-evolving area.
Viral infections, both newly acquired and reactivating from a pre-HSCT exposure, present a major risk to patients. Both CMV-seropositive patients and CMV-seronegative patients who have received grafts from seropositive donors are at risk for CMV, although the risk for reactivation is greatest for seropositive recipients with a seronegative donor, owing to patient reactivation of latent virus in the context of naïve donor T cells. A large multicenter trial found that patients receiving a UD HSCT from CMV-positive donors had an improved overall 5-year survival compared with patients receiving cells from CMV-negative donors. T-cell depletion abrogated this effect, which suggested that the improved survival was due to transfer of donor immunity. The clinical manifestations of CMV after HSCT include pneumonia/interstitial pneumonitis, colitis, hepatitis, and CMV-related cytopenias. Before the use of ganciclovir for CMV prophylaxis and treatment, more than a third of allogeneic SCT patients developed CMV infections. Almost 20% developed pneumonia, with a mortality rate of 85%. With the availability of effective antiviral therapies, almost all patients at risk for CMV disease receive prophylaxis during the HSCT admission. Randomized studies have found acyclovir, valacyclovir, and ganciclovir prophylaxis initiated at engraftment to be effective at reducing CMV disease before day 100. The use of prophylactic acyclovir has been shown to decrease overall mortality for at-risk patients. These studies found that the use of prophylactic ganciclovir after engraftment was limited by drug-induced cytopenias and other toxicities. There are multiple studies of preemptive CMV treatment based on the results of positive culture, polymerase chain reaction assay, antigen testing, or bronchoalveolar lavage fluid evaluation. A review of the published CMV literature since 1995 found no advantage to prophylactic treatment over preemptive treatment in response to screening tests. Additionally, this review found that preemptive therapy based on polymerase chain reaction tests or antigen assay was superior to culture or bronchoalveolar lavage–based strategies. Both ganciclovir and foscarnet are considered to be first-line therapy for CMV reactivation or treatment of disease. Ganciclovir and foscarnet are similar in efficacy and primarily differ in terms of side-effect profile. CMV-seropositive patients undergoing autologous HSCT have not generally received either screening or prophylaxis, although CMV disease occurs in a small percent of these patients.
Up to 50% of patients undergoing either autologous or allogeneic HSCT experience varicella-zoster virus reactivation, usually within the first year after SCT. The vast majority of patients respond to acyclovir treatment. In children, the onset may be earlier, with fewer cases of visceral involvement. In general, pediatric patients respond well to antiviral therapy and fatalities are rare. Treatment with acyclovir for 1 year after HSCT is the current recommendation for any patients with a pre-HSCT history of varicella infection. Varicella vaccination is currently considered optional after autologous or allogeneic HSCT. A pilot study of the live-attenuated vaccine in autologous patients found the vaccine to be safe, but efficacy data are still pending.
Fungal infection remains a significant problem, especially in those receiving corticosteroids for treatment or prophylaxis of GVHD. A randomized trial demonstrated that administration of prophylactic fluconazole for 75 days after allogeneic HSCT yields ongoing protection against disseminated candidal infections and improved survival. However, prophylactic fluconazole has been associated with the emergence of resistant species such as Candida krusei. Low-dose amphotericin B has also been used prophylactically and is as effective as fluconazole but more toxic. The use of liposomal amphotericin has improved the toxicity profile. Voriconazole has a broader spectrum of antifungal activity than fluconazole, but a randomized trial comparing the two agents as prophylactic therapy failed to show a survival difference in allogeneic HSCT patients.
All HSCT patients are at risk for the development of Pneumocystis jirovecii after transplant. Standard practice is to administer prophylaxis to recipients of autologous HSCT for 6 months after day 0 and for 6 months after discontinuation of immunosuppression for recipients of allogeneic transplant.
Veno-Occlusive Disease of the Liver
Veno-occlusive disease, also known as sinusoidal obstruction syndrome, is a clinical syndrome occurring in the early posttransplant period and characterized by hepatomegaly, right upper quadrant pain, jaundice/increased bilirubin level, and fluid retention. VOD can occur after either autologous or allogeneic SCT and results from chemotherapy/irradiation-induced damage to endothelium and hepatocytes. Injury to the sinusoidal endothelial cells and hepatocytes in zone 3 of the liver acinus appears to be the initial event, resulting in subendothelial edema and endothelial cell damage and subsequent microthromboses, fibrin deposition, and the expression of factor VIII/vWF within venular walls. Later features include collagen deposition within venular lumens with eventual luminal obliteration and hepatocyte necrosis. Identified risk factors for developing VOD include previous chemotherapy regimens containing gemtuzumab ozogamicin, BU-based conditioning regimens (especially when combined with CY and methotrexate), prior intensive chemotherapy (as seen in advanced-stage malignancies), total parenteral nutrition for more than 30 days, and transplantation from UDs. Pretransplant iron overload may also potentiate the development of VOD, and a pre-HSCT ferritin level of more than 1000 is an additional identified risk factor in some studies.
The first clinical signs of VOD are hepatomegaly and right upper quadrant pain with fluid retention, typically occurring 10 to 20 days after chemotherapy administration. Other clinical signs include hyperbilirubinemia, peripheral edema, ascites, sodium avidity, and pulmonary infiltrates/effusion/edema. Patients with VOD are at increased risk for developing renal insufficiency and failure. It is hypothesized that portal hypertension from sinusoidal injury leads to decreased renal perfusion and tubular injury, resulting in renal damage. Hepatorenal syndrome occurs in approximately half of patients with severe disease and is associated with changes in mental status.
It is essential to exclude other causes of liver disease (e.g., sepsis, portal vein thrombosis) before a diagnosis of VOD can be made. Liver biopsy is the gold standard for diagnosis and along with hepatic venous pressure measurements can still provide valuable diagnostic information, although it is associated with considerable morbidity in this critically ill population. Doppler ultrasonography can help confirm the diagnosis, but classic findings are not always present early in course of the disease. If clinical concern is high, repeating the ultrasound evaluation after several days may be helpful. However, the presence of ascites, gallbladder wall thickening, hepatomegaly, decreased or reversed flow in the portal vein, and increased mean hepatic artery resistive index are support of the diagnosis. Recent clinical guidelines suggest that clinical criteria are sufficient to make the diagnosis while ultrasonography or liver biopsy can be reserved for cases with high levels of diagnostic uncertainty.
Reported overall survival rates for pediatric patients with established VOD range between 53% and 100%. Predictors of mortality in children with VOD include the use of allogeneic donors other than a matched sibling, concomitant moderate-to-severe hepatic or cutaneous GVHD, and higher VOD severity (e.g., higher peak bilirubin, higher maximal weight gain, presence of pleural effusion, requirement for intensive care unit admission).
Treatment and prophylactic options for patients with VOD have evolved over the past decade. The most straightforward preventative technique is to identify patients at highest risk and to consider alternative conditioning regimens when feasible. Ursodeoxycholic acid has been used as a preventative agent with inconclusive results. Other strategies such as the use of fresh-frozen plasma, prostaglandin E 1 , N -acetylcysteine, and antithrombin III are potentially effective but require further study before being considered a standard approach. Because the majority patients can recover spontaneously, supportive care with meticulous fluid and blood product management remains the mainstay of therapy for moderate VOD. Based on histologic observations of microthrombi and fibrin deposition coupled with laboratory evidence of abnormalities in the coagulation cascade, many therapeutic strategies have been directed at promoting thrombolysis or fibrinolysis. Multiple small studies have investigated the use of thrombolytic agents and anticoagulants (typically tissue plasminogen activator and heparin) for VOD prevention and/or treatment. Although these strategies appear to be generally safe, they have not shown significant reduction in the incidence or outcome of patients with severe disease and have been associated with serious bleeding in some cases.
Defibrotide is a single-stranded polydeoxyribonucleotide that has antithrombotic and thrombolytic activity. It has been demonstrated to be effective with minimal toxicity for both the prevention and treatment of VOD. The mechanism of action is unclear, but it appears to promote angiogenesis and promote sinusoidal endothelial regrowth and has thrombotic/thrombolytic properties. Defibrotide prophylaxis has been shown to be safe in adults and children; efficacy in preventing VOD has been demonstrated in small studies, but there is still a paucity of large studies or randomized trials. Response rates between 35% and 50% have been reported for patients with severe VOD treated with defibrotide, and it is not associated with increased risk for major bleeding.
Refractory VOD portends poor outcome with limited therapeutic options. Liver transplant has resulted in clinical improvement in about 30% of the small numbers of patients stable enough to undergo the procedure; the immediate- and long-term issues are formidable, however. Transjugular intrahepatic portosystemic shunting (TIPS) has been performed safely in patients with severe VOD, although it has not been shown to improve overall survival and should be reserved for instances in which fluid retention and ascites are relatively isolated issue.
In summary, VOD is an early complication of HSCT that occurs more frequently in patients with prior hepatic injury, intensive prior therapy, or exposure to conditioning regimens with known hepatotoxicity. The diagnosis is made on clinical criteria, including hepatomegaly, ascites, right upper quadrant pain, and increased bilirubin level; ultrasound findings can be used to further support the diagnosis. There is no standard approach to prophylaxis. Moderate VOD can be self-limiting, but multiple studies have shown a decrease in mortality from severe VOD when defibrotide therapy is employed.
Immune Reconstitution
The period after HCST is characterized by profound immunodeficiency, and infection complications remain an obstacle to improved HSCT outcomes. A common feature of transplant regimens, particularly for unrelated HSCT, is the use of chemotherapeutic agents and/or antibodies that effectively deplete the host of many lineages of the immune system. Myeloablative conditioning therefore results in the loss of immune cells, including T, B, and NK cells that are necessary to confer protective immunity, although some host immune populations such as resident macrophages persist after conditioning. Although hematopoiesis can return within weeks of transplant, recovery of a functional immune system can take much longer, and the risk for opportunistic infection remains high for up to a year even in uncomplicated transplants. The rapidity and extent of immune reconstitution after transplantation is therefore an important determinant in outcome of HSCT.
The kinetics of recovery of the cells in the immune system after HSCT tends to follow a relatively predictable pattern. Recipients of autologous HSC rapidly recover CD3 T-cell counts within 6 to 8 weeks after transplant, whereas allogeneic HSCT recipients do not normalize their T-cell counts until 12 weeks or later. CD8+ T cells recover more rapidly than do CD4+ T cells, resulting in an inversion of the normal CD4+:CD8+ T-cell ratio, although the clinical significance of this is not fully understood. NK cells reappear early after HSCT and may represent the major lymphocyte population present in the initial weeks after HSCT. This timetable of immune recovery dictates the vaccination schedule of patients after HSCT, and most centers do not commence reimmunization for HSCT patients until 6 months after transplant. The rate of recovery of the immune system also has significant implications for the rate of infection, because slow recovery of immune function is associated with an increase in the cumulative incidence of infections and nonrelapse mortality.
The recovery of the T-cell compartment in HSCT patients is from both expansion of mature T cells delivered in the donor inoculum and from de novo differentiation of T cells from early stem cell and lymphoid progenitors. During the early period after transplant, T cells with a naïve phenotype predominate in the peripheral blood, suggesting repopulation of the T cell compartment with recent thymic emigrants. The relative numbers of recent thymic emigrants can be quantified in the peripheral blood T-cell pool by the quantitative detection of genomic DNA that is present only in thymocytes (T-cell receptor excision circle [TREC] DNA). These methods have shown that increased thymic output contributes to the expanding peripheral T-cell pool after transplant for 12 to 24 months after HSCT. This thymic output occurs even in adult patients whose thymuses have presumably involuted. The rate of increase in T cells is usually much more rapid in pediatric HSCT patients, consistent with the presence of more robust thymic function. The advantage of a functional thymus in immune reconstitution has been demonstrated by the marked decrease in the fraction of naïve peripheral blood T cells in a 15-year-old HSCT patient who had had a previous thymectomy relative to thymus-bearing HSCT patients. The delay in recovery of the T-cell compartment is reflected in a prolonged time to manifest a normal range of T-cell diversity. Several studies have shown that it can take as long as 12 to 24 months after HSCT for a full range of T-cell receptor diversity to be present in the T-cell compartment.
Mature T, B, and NK cells are present in the donor inoculum of bone marrow, peripheral blood, or UCB and can contribute to some degree of host immunity in the immediate posttransplant period. The ability of these transferred cells to provide immune protection is dependent on how immunologically “experienced” the donor is. For instance, recipients of grafts from donors who are CMV immune, that is, who have previously been infected with the virus and have mounted a protective immune response, have a lower rate of CMV disease, presumably because of transferred immunologic memory to that virus. Similarly, recipients whose donors have been vaccinated with a pneumococcal vaccine respond to pneumococcal vaccines more rapidly and with higher antibody titers after transplant, again suggesting that donor immunity can be transferred to the recipient. However, full immune reconstitution, with the ability to mount a protective immune response to new pathogenic challenges, is dependent on the differentiation of new immune cells from precursors in the donor graft inoculum.
Several factors influence the length of time that immunologic recovery takes. Age of BMT recipients profoundly affects the rate of immune reconstitution, with an inverse correlation between recipient age and the absolute number of T cells 1 year after HSCT. The source of HSC also has a marked effect on the rate of immune reconstitution. The use of PBSC is associated with a faster rate of recovery than using HSC from bone marrow, possibly as a result of a larger cell dose. However, increasingly intensive conditioning regimens are delaying immune recovery in autologous recipients to a degree comparable with allogeneic HSCT. Although early data suggested that immune reconstitution in children after UCB HSCT is comparable to those receiving unrelated BMT, subsequent studies have shown that UCB HSCT is associated with a marked delay in the recovery of T-cell numbers and function. The presence of GVHD can significantly delay immune reconstitution both as a result of disruption of normal T-cell development and from the added immunosuppression that is required for management of the disease.
Graft Rejection
Primary graft rejection, defined as the failure to recover hematopoietic function of the stem cell graft by day (+) 100, is an uncommon, life-threatening event. Rejection occurs after approximately 4% of UD stem cell transplants and is even less common in RD transplants. It is an immune-mediated process that occurs because of genetic disparity between the donor and recipient. Although both NK and T cells are considered mediators of graft rejection, it is characterized by the presence of recipient T cells and the gradual loss of donor cells. The risk for graft rejection is increased in patients who are mismatched at HLA-A, HLA-B, or DRB1 loci, receive a low cell dose, have a positive crossmatch for antidonor lymphocytotoxic antibody, receive T-cell depletion as GVHD prophylaxis, have aplastic anemia, especially if previously multiply transfused, or have storage disorders or osteopetrosis. An altered host microenvironment can also contribute to graft rejection.
Adjustments of both chemotherapeutic and radiotherapeutic components of conditioning regimens, the use of monoclonal antibodies targeting selected populations of recipient T cells, and increases in cell dose (including the use of PBSCs in addition to bone marrow) are among the interventions that have shown some promise in preventing graft rejection. Depletion of host T cells with antithymocyte globulin or the use of CD52 monoclonal antibodies to deplete residual recipient lymphocytes are commonly used options to decrease graft rejection. A number of approaches have been exploited to overcome the increased incidence of graft failure associated with T-cell depletion. First, simply increasing the number of stem cells in the graft has been successful, implying that residual host hematopoietic elements are involved in mediating graft rejection. However, such intensification is associated with increased GVHD disease. Alternatively, approaches to increasing the number of stem cells in the graft using manipulation of Notch or prostaglandin pathways are being explored. Additionally, because the risk for graft failure appears to increase with more exhaustive T-cell depletion, less extensive T-cell removal from the graft with and without T-cell add-back has been attempted. More recently attempts to reduce rejection with the use of mesenchymal stem cells have been explored.
Therapeutic options for patients with graft rejection include second allogeneic transplant, the infusion of frozen autologous stem cells, the use of hematopoietic growth factors, particularly granulocyte-macrophage colony-stimulating factor (GM-CSF), modification of the host’s immunologic status, and the infusion of donor leukocytes. Infusion of stem cells with or without additional conditioning can be an effective intervention for the treatment of primary graft failure. It has been studied using bone marrow or PBSCs, although recently the use of unrelated UCB units has been explored. The use of allogeneic PBSCs is appealing in the second transplant setting because PBSCs have been associated with shorter duration of the neutropenic phase and a higher neutrophil recovery rate. Graft rejection is a potentially morbid, uncommon complication of HSCT with limited effective therapies.
Graft-Versus-Host Disease
Acute Graft-versus-Host Disease
One of the most significant barriers to successful HSCT is acute graft-versus-host disease (aGVHD). aGVHD is a syndrome caused when immunologically competent cells contained in the bone marrow or peripheral blood recognize host tissues, resulting in inflammation and tissue damage. Normally, allogeneic cells contained in simple blood transfusions are rapidly eliminated by the host immune system. However, in the setting of HSCT, the immune system of the recipient is suppressed, allowing donor immune cells to persist. The syndrome of aGVHD was first reported in 1954 by Barnes and Loutit, who observed that lethally irradiated animals given allogeneic spleen cells developed skin abnormalities and diarrhea and died of a wasting illness. It became clear that for aGVHD to develop, certain criteria had to be in place, and these were summarized by Billingham in 1966 : (1) “the graft must contain immunologically competent cells”; (2) “the host must possess important transplantation alloantigens that are lacking in the donor graft, so that the host appears foreign to the graft and is, therefore, capable of stimulating it antigenically”; and (3) “the host itself must be incapable of mounting an effective immunological reaction against the graft, at least for sufficient time for the latter to manifest its immunological capabilities; that is, it must have the security of tenure.”
In general, the greater the degree of genetic difference between donor and recipient, the higher the risk for and severity of aGVHD. The main genetic loci that determine the compatibility of donor and recipient are HLA classes I and II located on chromosome 6. Each of these loci encode polymorphic glycoproteins—major histocompatibility antigens—that are expressed on the surface of human tissues in a complex with small peptides from degraded intracellular proteins. HLA class I molecules (HLA-A, HLA-B, and HLA-C) are expressed on all nucleated cells, whereas class II antigens (HLA-DR, HLA-DQ, and HLA-DP) are primarily expressed on hematopoietic cells. T-cell receptors on the surface of T cells engage with allogeneic MHC molecules, resulting in the activation and expansion of alloantigen-specific T cells. The recognition of allogeneic MHC molecules is bidirectional: recipient T cells recognize donor antigens and can cause graft rejection; conversely, donor T cells can recognize recipient antigens and are responsible for the syndrome of aGVHD.
The mechanisms by which T cells cause GVHD include both direct effector mechanisms (i.e., killing of host cells by alloantigen-specific T cells) and also the release of inflammatory mediators that cause tissue damage. The presence of tissue damage either from immunologic events or from direct effects of conditioning can propagate the inflammatory response, triggering further immune responses, in a positive feedback loop that has been called a “cytokine storm.”
Despite the pathologic effects of aGVHD, the allogeneic immunologic effect may also confer benefits. Patients with hematologic malignancies who develop mild-to-moderate GVHD have a decreased risk for disease relapse. This is believed to be because graft inocula contain immunologic cells that are capable of recognizing malignant cells and mediating a graft-versus-leukemia and, perhaps, a graft-versus-tumor effect, although whether T cells that recognize tumor cells are distinct from those that simply recognize allo-MHC is not fully understood. Conversely, T-cell depletion from the marrow inocula reduces the risk for GVHD but can lead to an increased risk for both graft rejection and disease recurrence.
The clinical syndrome of aGVHD develops within 100 (and usually within 60) days of allogeneic SCT. Chronic GVHD (cGVHD) is a distinctive clinical syndrome that occurs after day 100 and is discussed later. aGVHD classically involves the skin, liver, and lower gastrointestinal tract, although the degree of involvement of each organ varies. The first manifestation of aGVHD is often a rash, presenting as a maculopapular eruption, commonly involving the palms and soles, back of neck and ears at first, and later the trunk and extremities. Biopsy specimens from involved areas demonstrate epidermal basal cell vacuolization, followed by epidermal basal cell apoptotic death with lymphoid infiltration. Characteristic eosinophilic bodies may be seen. The specificity of skin biopsy is not high, and it is often difficult to differentiate aGVHD from drug allergy or reaction to the conditioning regimen. For this reason, skin biopsy specimens obtained early on in the transplant course are often nondiagnostic. Bullae formation with epidermal separation and necrosis is seen in advanced stages of skin GVHD.
Hepatic GVHD often presents as cholestatic jaundice, which must be differentiated from other causes of liver disease frequently seen in HSCT patients, including VOD, infection, and drug toxicity. Liver biopsy may be helpful because characteristic pathologic changes can be observed that aid diagnosis, although the risk for bleeding complications is often high.
Gastrointestinal involvement presents as watery diarrhea with a “seedy” component and may progress to include crampy abdominal pain, bleeding, and even ileus. By convention, stool volume is used to quantitate the severity of gut involvement. Rectal or colonic biopsy may reveal crypt cell necrosis with lymphocytic infiltration, and, with increasing severity, crypt abscess or loss and mucosal denudation.
The clinical signs of aGVHD are not pathognomonic and overlap with a broad range of other complications frequently encountered during HSCT. For that reason, tissue biopsy samples of affected organs are usually obtained to confirm diagnosis by histology and exclude other infectious causes. Several groups have sought to develop better diagnostic tests for aGVHD, focusing on proteins found in the plasma that correlate with and predict the presence of GVHD; however, none has yet become integrated into clinical care.
The severity of aGVHD is graded based on staging the involvement of individual organs and the clinical performance of the patient and then determining an overall grade for the disease. The modified Glucksberg criteria, introduced in 1974, is the system historically used for assessing aGVHD ( Tables 8-1 and 8-2 ). The International Bone Marrow Transplant Registry (IBMTR) constructed a severity index based on organ involvement alone with no need for subjective assessment of performance ( Table 8-3 ). This has been shown to correlate well with outcome. Skin, liver, and gut are staged individually for severity of involvement, after which an overall grade of GVHD is assigned.
Stage 0 | Stage 1 | Stage 2 | Stage 3 | Stage 4 | |
---|---|---|---|---|---|
Skin | No rash | Rash <25% body surface area | 25-50% | >50% generalized erythroderma | Bullae and desquamation |
Intestines | <10 mL/kg/day | 10-19.9 mL/kg/day | 20-30 mL/kg/day | >30 mL/kg/day | Severe abdominal pain, ileus, blood or melena |
Upper Gastrointestinal Tract | Severe nausea/vomiting | ||||
Liver | Bilirubin ≤2 mg/dL | Bilirubin 2.1-3 mg/dL | Bilirubin 3.1-6 mg/dL | Bilirubin 6.1-15 mg/dL | Bilirubin >15 mg/dL |
Overall Clinical Grade | Organ System | Clinical Stage |
---|---|---|
I (mild) | Skin | 1-2 |
Liver | 1 | |
Gastrointestinal | 0 | |
II (moderate) | Skin | 1-3 |
Liver | 1-2 | |
Gastrointestinal | 1 | |
III (severe) | Skin | 2-4 |
Liver | 2-4 | |
Gastrointestinal | 2-4 | |
IV (life threatening) * | Skin | 2-4 |
Liver | 2-4 | |
Gastrointestinal | 2-4 |
Severity Index | Skin | Liver | Gastrointestinal | Upper Gastrointestinal |
---|---|---|---|---|
A | 1 | 0 | 0 | 0 |
B | 2 | 1-2 | 1-2 | 1 |
C | 3 | 3 | 3 | — |
D | 4 | 4 | 4 | — |
Several risk factors have been identified for the development of aGVHD. Increasing genetic disparity between the donor and host increases the incidence and severity of GVHD and is the most important single predictor of aGVHD. A large retrospective study of adults undergoing HSCT showed that risk factors for aGVHD grades II through IV included transplantation from UDs, mismatched RDs or UDs, the use of a TBI-based conditioning regimen, and the use of a female donor. More recently, other genetic risk factors have been evaluated. The existence of polymorphisms in genes between donor and host offers a plausible mechanism to explain variations in magnitude of the inflammatory response to tissue injury and hence GVHD. Indeed, specific polymorphisms in the recipient interleukin (IL)-10 promoter region and IL-10 receptor beta gene (IL10RB) have been found to be associated with a lower rate of GVHD. Many other candidate genes are currently being examined for similar associations. Moreover, the falling cost and growing scale of genomic sequencing technologies suggest that an increasingly complex and accurate genomic prediction of GVHD risk is likely to evolve over the coming years.
Almost all patients receiving an allogeneic HSCT receive immunosuppressive therapy as prophylaxis to prevent GVHD. Prophylactic regimens originally developed in the early years of the development of HSCT included methotrexate, cyclosporine, and corticosteroids. A common prophylactic regimen includes cyclosporine given intravenously at a dose of 1.5 mg/kg every 12 hours until oral administration at a dose of 6.25 mg/kg every 12 hours is tolerated. Doses are adjusted to achieve desired blood levels. Cyclosporine is continued on a tapering schedule through day 180 after transplant. Methotrexate is given at 15 mg/m 2 on day 1 and at 10 mg/m 2 on days 3, 6, and 11 after marrow infusion. Tacrolimus, which, like cyclosporine, is a calcineurin inhibitor, was tested in combination with methotrexate and found to decrease aGVHD but not overall survival. Mycophenolate mofetil (MMF) inhibits T-cell proliferation by interfering with the purine synthetic pathway and is sometimes used instead of methotrexate to minimize toxicity (especially mucositis). Sirolimus, originally identified as an antifungal agent, has potent immunosuppressive properties and, in combination with tacrolimus, is an effective prophylactic regimen. However, the risk for VOD is increased by the use of sirolimus in some settings.
Despite prophylaxis, 10% to 30% of children undergoing HSCT will develop aGVHD. For these patients, frontline therapy consists of systemic corticosteroids, typically at a dose of 2 mg/kg/day of methylprednisolone, together with calcineurin-inhibition with cyclosporine or tacrolimus ( Fig. 8-1 ). There is no advantage to initial therapy with higher doses of corticosteroids. For patients who respond to initial therapy, corticosteroids are tapered by 10% every 3 to 5 days and then more slowly after doses are decreased to less than 1 mg/kg/day. However, because approximately 35% of patients do not improve with initial corticosteroid therapy, several other agents have been used in combination with corticosteroids to increase the efficacy of frontline treatment. A recent, randomized phase II study in adults undergoing HSCT demonstrated that the addition of MMF showed improved efficacy compared with etanercept (which inhibits tumor necrosis factor [TNF]), denileukin (which targets cells expressing the IL-2 receptor), or pentostatin (which inhibits purine synthesis).
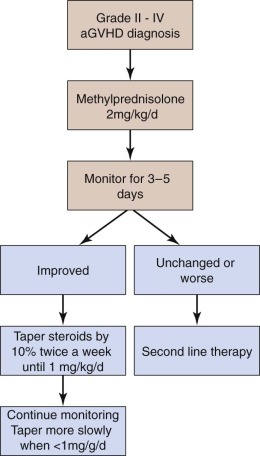
If aGVHD progresses or fails to improve after 5 to 7 days of initial corticosteroid therapy, it is considered to be corticosteroid refractory and additional immunosuppressive therapies are added. A variety of other pharmacologic agents have been tried, including methotrexate, MMF, IL-2 receptor–targeting agents, etanercept, infliximab, antithymocyte globulin, and sirolimus. However, none is clearly superior; and, instead, most clinicians select second-line aGVHD therapy based on the effects of previous treatment regimens used in a given patient and on the spectrum of toxicities associated with each agent.
Chronic Graft-Versus-Host Disease
GVHD was initially characterized as occurring in two phases: aGVHD generally presented within the first 100 days after transplant, whereas cGVHD occurred after day 100 ( Table 8-4 ). cGVHD, defined by both clinical and histopathologic criteria, can present as early as day 50 to 60 days after marrow infusion. The pathogenesis of cGVHD is not completely elucidated. It is believed to involve donor T-cell recognition of minor histocompatibility antigens or loss of peripheral T-cell tolerance, leading to symptoms that are very similar to those present in autoimmune disorders. The morbidity and mortality of cGVHD is most severe in patients who have progressive disease after aGVHD and tends to be less severe in those in whom it occurs de novo. Risk factors for development of cGVHD include HLA disparity between donor and host, prior aGVHD, increasing patient age, and use of a TBI-based conditioning regimen. Age is also a risk factor: patient age older than 15 years or donor age older than 5 years is associated with significantly increased risk. In addition, the use of adult female donors for male recipients increases the likelihood of cGVHD, likely related to donor antibody responses to antigens coded for by genes on the recipient Y chromosome. The impact of pediatric female donors on the incidence of cGVHD has not been evaluated.
Acute GVHD | Chronic GVHD | |
---|---|---|
Onset Day | 7-60 (up to 100) | Day >100 (may be seen from day 60 on) |
Manifestations | ||
Skin | Erythematous rash | Sclerodermatous changes |
Gastrointestinal | Secretory diarrhea | Dry mouth; sicca syndrome |
Liver | Hepatitis/cholestasis | Cholestasis/cirrhosis |
Other | Fever | Pulmonary dysfunction, alopecia, thrombocytopenia, xerostomia |
cGVHD is a disease with a constellation of clinical and pathologic features. The syndrome resembles autoimmune systemic collagen vascular diseases with protean manifestations potentially involving almost every organ ( Table 8-5 ). Pathologic changes in the skin result from epithelial cell damage characterized by basal cell degeneration and necrosis, and, later, epidermal atrophy and dermal fibrosis. Skin involvement may present as lichen planus, areas of local erythema, and areas of hyperpigmentation or hypopigmentation. The skin may be dry, freckled, or ulcerated; and findings are aggravated by exposure to sunlight. Sclerodermatous changes may evolve to flexion contractures that can greatly impair function and may be helped by surgical release procedures. Hair follicles can be involved with resulting partial or total alopecia. Ocular abnormalities are common, and patients present with sicca syndrome, including dry eyes and photophobia; decreased tearing is quantitated by a Schirmer test. Dry mouth is a common presenting complaint of oral GVHD, and decreased saliva production predisposes to caries. Tongue depapillation or scalloping of the lateral margins of the tongue can be seen. The tongue, buccal mucosa, and gums may have lichen planuslike lesions, which must be differentiated from oral candidiasis. A biopsy specimen typically shows lichenoid changes with mononuclear infiltrates, epithelial cell necrosis, and salivary gland inflammation, lymphocytic infiltrate, and fibrosis. Involvement of the liver with cGVHD is manifest by obstructive jaundice, which may progress, if untreated, to bridging necrosis and cirrhosis. Gastrointestinal signs include inanition with progressive weight loss, chronic malabsorption, diarrhea, anorexia, nausea, and vomiting. Biopsy demonstrates single cell apoptosis with crypt destruction and may show fibrosis of the lamina propria. Early cGVHD may present as diffuse myositis and tendonitis. Progressive joint involvement, which may result from the myositis and tendonitis or simply from overlying skin fibrosis, results in decreased range of motion and flexion contractures. Perhaps the most debilitating effect of cGVHD occurs with pulmonary involvement, manifest as obstructive lung disease progressing to bronchiolitis obliterans. Persistent immunodeficiency with profoundly depressed B- and T-cell responses predisposes to recurrent and severe infections. Patients may have reduced IgG2 and IgG4, decreased mucosal IgA, diminished delayed-type hypersensitivity, and functional asplenia. They are susceptible to opportunistic infections with encapsulated organisms (primarily pneumococcus), P. jirovecii, varicella-zoster, and herpes simplex and may have a variety of chronic infections, including sinusitis, bronchitis, and conjunctivitis.
Organ System | Symptoms and Signs | Biopsy Findings |
---|---|---|
Systemic | Recurrent infections with immunodeficiency | |
Skin | Lichen planus, scleroderma, hyperpigmentation or hypopigmentation, dry scale, ulcerated, erythema, freckling Flexion contractures | Epithelial cell damage, basal cell degeneration and necrosis, epidermal atrophy and dermal fibrosis |
Hair | Alopecia | |
Mouth | Sicca syndrome, depapillation of tongue with variegations, scalloping of lateral margins | Lichenoid changes with mononuclear infiltrates, epithelial cell necrosis, salivary gland inflammation, lymphocyte infiltration, fibrosis |
Joints | Decreased range of motion, diffuse myositis/tendonitis | |
Eyes | Decreased tearing, injected sclerae, conjunctivae | |
Liver | Increased alkaline phosphatase > aminotransferases and bilirubin Cholestasis, cirrhosis | Focal portal inflammation with bile ductile obliteration, chronic aggressive hepatitis, bridging necrosis, cirrhosis |
Gastrointestinal | Failure to thrive (children), weight loss (adults) Esophageal strictures, malabsorption, chronic diarrhea | Crypt destruction, single-cell dropout, fibrosis of lamina propria |
Lung | Cough, dyspnea, wheezing Bronchiolitis obliterans, chronic rales, pneumothorax | |
Hematology | Refractory thrombocytopenia, eosinophilia, Howell-Jolly bodies |
Staging of cGVHD is based on degree of organ involvement ( Box 8-2 ). Limited cGVHD has localized skin involvement with or without hepatic dysfunction. All other manifestations of cGVHD are classified as extensive cGVHD. Limited cGVHD may resolve spontaneously without specific therapy, and these patients have a favorable outcome. Patients with lichenoid skin changes, progressive presentation of cGVHD, and hyperbilirubinemia have a worse prognosis. Recent work has focused on developing a more detailed comprehensive scale to measure symptoms of cGVHD so that response to therapy can be more clearly assessed.
Limited Chronic GVHD
Localized skin involvement and/or
Hepatic dysfunction due to chronic GVHD
Extensive Chronic GVHD
Generalized skin involvement or
Localized skin involvement and/or hepatic dysfunction due to chronic GVHD, plus:
Liver histology showing chronic aggressive hepatitis, bridging necrosis, or cirrhosis, or
Eye involvement: Schirmer test (<5 mm wetting), or
Involvement of minor salivary glands or oral mucosa demonstrated by buccal biopsy, or
Involvement of any other target organ
It is essential that patients with cGVHD are managed by a multidisciplinary team with expertise in this complicated and often frustrating condition. Corticosteroids are usually the first approach to therapy, and a regimen of alternating-day cyclosporine and prednisone was shown to be effective in more than half of patients and to have an acceptable toxicity profile. There is no standard approach for patients who are refractory to initial therapy or whose disease flares during taper of immunosuppression. A number of other therapies are being tested, including high-dose pulse corticosteroids, extracorporeal photopheresis, daclizumab (a humanized IL-2 receptor antagonist), mycophenolic acid, rapamycin, rituximab, imatinib, and pentostatin. Recent studies have also examined the use of IL-2 in the treatment of GVHD, which may work by selectively expanding regulatory T cells.
All of the therapeutic options significantly impact immune function, and most fatalities occur from infectious complications versus specific organ toxicity from cGVHD. Meticulous and preemptive management of the complications associated with cGVHD and its therapy is a crucial component of care. Recommendations for patient education, prophylactic interventions, and follow-up are extensively outlined in the National Institutes of Health Ancillary Therapy and Supportive Care Working Group document.
Organ Toxicity Associated with Hematopoietic Stem Cell Transplantation
Ophthalmologic Complications
A number of different ophthalmologic complications can occur as sequelae of HSCT. Decreased lacrimation secondary to cGVHD may contribute to ocular sicca syndrome and the related complications of photophobia, punctate keratopathy, scar formation, and corneal perforation. Dry eye syndrome can occur independent of GVHD and is attributed to immunosuppression and conditioning with TBI. Therapy includes topical or systemic immunosuppression, lubrication, artificial tears, and lacrimal duct (punctual) plugging, which can be useful even in young children. Fluid-ventilated scleral lenses have been used to treat adolescents and adults with sicca syndrome and have decreased corneal abrasions and improved vision. Patients remain at risk for ocular infections, particularly with herpes simplex viruses. Cyclosporine use is associated with a number of eye complaints, including a well-described syndrome of headache, hypertension, seizures, visual impairment, or any combination of these symptoms, as well as temporary blindness (see “ Neurologic Complications ” for a detailed description). In addition, transplant-related retinopathy can be manifested as optic disc edema, either asymptomatic or accompanied by visual blurring, in patients taking cyclosporine. Ischemic fundal lesions, detected as cotton-wool spots and, in some patients, as optic disc edema, have been reported in patients treated with both TBI and cyclosporine and were not observed in patients who did not have concordance for these risk factors. Lesions appeared 3 to 6 months after SCT, with complaints of decreased visual acuity or other visual disturbances. Most patients discontinued cyclosporine, and half were treated with systemic corticosteroids. The majority recovered visual acuity, and their ischemic lesions resolved over time, but little further information is available about the course or long-term prognosis of this complication.
Retinal hemorrhages are relatively common after transplantation and in one study were found in 12% of children undergoing scheduled ophthalmologic examination after transplant. In this study, the hemorrhages were located intraretinally, did not threaten vision, and developed in the acute transplant period in the setting of thrombocytopenia. In all cases the hemorrhages ultimately resolved when the platelet count recovered.
Occlusive microvascular retinopathy has also been described after autologous SCT in patients receiving high-dose cytarabine and TBI, thus suggesting that particular high-dose chemotherapy regimens may predispose to vascular injury at otherwise safe radiation doses. Other infrequent complications of transplantation include chorioretinal lesions, vitritis, increased ocular pressure, and retinal hemorrhages.
Cataracts are a common complication of HSCT. Radiation is a well-described predisposing factor. GVHD and treatment with corticosteroids are inconsistent risk factors across studies. Although historically the incidence was as high as 80% in patients receiving single-dose TBI, patients receiving fractionated TBI or chemotherapy-only regimens still have a 20% to 50% incidence of cataracts 5 to 6 years after transplantation. The relative risk for cataracts and the need for subsequent cataract surgery may also be related to previous therapy or to glucocorticoid use for prophylaxis or management of GVHD.
Overall, reports of large pediatric populations have shown that some type of ophthalmologic abnormality occurs in up to 50% of patients, although visual function remains excellent in virtually all patients. Thus yearly examination by an ophthalmologist is essential, including a Schirmer test to rule out xerophthalmia and a thorough retinal evaluation.
Dental Complications
Disturbances in dental development have been described in children conditioned with TBI, particularly if HSCT took place before 6 years of age. Characteristic findings include short dental roots, absence of root development, and microdontia. A review of 52 pediatric patients younger than 10 years at HSCT found that all patients had disturbances in dental root growth, with the most pronounced changes occurring in those 3 to 5 years old at the time of transplantation. Subsequent decreased alveolar bone growth may lead to further compromise of dental development.
Oral sicca syndrome (occurring as a complication of either TBI or cGVHD, or both) may result in significant morbidity, including chronic oral mucosal injury, poor oral hygiene, and subsequent dental decay. Patients report frequent thirst, altered taste, difficulty eating and swallowing, and oral sensitivity. The salivary glands are a common site of cGVHD and results in both quantitative and qualitative deficiencies of the major and minor salivary glands resulting in decreased salivary flow. Decreased salivary production alters the biochemical and immunologic composition of the saliva and can contribute to the development of caries. The primary treatment of sicca syndrome includes sialogogue therapy—stimulants such as sugar-free gum or prescription medications. Patients with oral sensitivity should avoid foods that cause discomfort and heavily flavored toothpastes or alcohol-based mouthwashes.
Children with oral GVHD and certain underlying diseases such as Fanconi anemia are at heighted risk for oral cancers. These patients should receive routine evaluation by an oral medicine specialist comfortable with oral cancer screening. The effects of prophylactic interventions are not well reported, but routine oral hygiene including brushing and flossing and twice-yearly evaluation by a dentist knowledgeable about potential HSCT-related issues is recommended.
Pulmonary Complications
Acute and late noninfectious pulmonary complications are common and occur in as many as 50% of pediatric HSCT patients. Pulmonary illness complicates both autologous and allogeneic HSCT; however, these toxicities are more common after allogeneic transplant. Patients with pulmonary complications have a higher overall mortality compared with patients who do not develop pulmonary complications. The spectrum of noninfectious lung disease includes idiopathic pneumonia syndrome (IPS), diffuse alveolar hemorrhage (DAH), bronchiolitis obliterans syndrome (BOS), bronchiolitis obliterans organizing pneumonia (BOOP), and periengraftment respiratory distress syndrome (PERDS).
IPS is a potentially fatal acute complication of transplantation that is characterized by respiratory compromise, hypoxia, and diffuse radiographic change in the absence of an identifiable infectious cause. In 2010 the American Thoracic Society updated the definition of IPS as an idiopathic syndrome of pneumopathy after HSCT with evidence of widespread alveolar injury and in which infectious causes and cardiac dysfunction, acute renal failure, or iatrogenic fluid overload have been excluded. They further classified it into specific entities based in large part on the primary anatomic sites of inflammation and dysfunction. Subtypes of IPS include acute interstitial pneumonitis, DAH, delayed pulmonary toxicity syndrome (chemotherapy induced), and PERDS. IPS typically presents between 6 to 7 weeks after allogeneic HSCT, but studies have reported cases presenting earlier and later. Risk factors for IPS allogeneic HSCT include allogeneic transplant, full-intensity conditioning, the use of TBI in the conditioning regimen, the presence of aGVHD, older recipient age, and an underlying diagnosis of acute leukemia or MDS. The treatment of IPS includes supportive respiratory care with oxygen, diuretics, and often mechanical ventilation. The mainstay of medical therapy is systemic corticosteroids. Recently the soluble TNF-binding agent etanercept has shown promise if started early in the course of illness along with a corticosteroid. Despite advances in ventilatory management, supportive care, and medical therapy the mortality rate for IPS remains high. It is reported to be as high as 80% for all patients and 95% for ventilated patients. It should be noted that most reports of IPS include adult patients and it is likely that mortality rates are lower when children are considered independently.
DAH is an acute complication that typically develops in the periengraftment period in both allogeneic and autologous transplant patients. It is characterized by shortness of breath, cough, and hypoxemia, often with fever. The diagnosis of DAH is based on progressively bloodier return of bronchoalveolar fluid in the appropriate clinical setting. Frank hemoptysis is rare. Chest imaging demonstrates bilateral, diffuse infiltrates with potential areas of consolidation typically in the central lung. Risk factors for DAH include older age, transplantation for metabolic disease or solid tumor, severe mucositis, and renal insufficiency. DAH is treated with respiratory supportive care, transfusional support, and high-dose systemic corticosteroids (2 mg/kg/day to 1 g/m 2 /day) with slow taper. Even in the setting of rapid and complete treatment the mortality from DAH ranges from 60% to 100%, with death usually the result of multiorgan failure.
PERDS is a subset of IPS that occurs within 5 days of engraftment and is the respiratory component of clinical engraftment syndrome. Other components of engraftment syndrome include rash, fever, capillary leak, and diarrhea. Diagnostic criteria for PERDs include fever, hypoxia, and/or pulmonary infiltrates identified by imaging that occurs within 5 days of engraftment without evidence of infection or pulmonary dysfunction. There can be overlap with DAH, and approximately 30% of patients with PERDS have DAH. PERDS that occurs independently of DAH responds well to therapy with systemic corticosteroids (1 mg/kg/day), and death is uncommon.
BOOP (also called cryptogenic organizing pneumonia) presents as dry cough, fever, and dyspnea and is pathologically characterized by presence of granulation tissue within the alveolar ducts and alveoli. BOOP can present in the immediate posttransplant course or as late as 2 years after HSCT. Pulmonary function testing shows a restrictive defect, decreased diffusing capacity for carbon monoxide (DL co ), and hypoxemia. Chest imaging demonstrates patchy airspace consolidation and ground-glass and nodular opacities in a bilateral or unilateral pattern. Although clinical findings may support the diagnosis of BOOP, the diagnostic standard requires tissue either obtained by transbronchial or surgical biopsy. Unlike BOS, described next, BOOP responds well to systemic corticosteroids and most patients are long-term survivors.
Obstructive lung disease has been reported in up to 20% of children after allogeneic HSCT, which is similar to the incidence in adult patients. BOS is a noninfectious, potentially life-threatening complication of HSCT. It is a pathologic process that leads to the obstruction and eventual obliteration of the small airways and is characterized by chronic airflow obstruction that is unresponsive to bronchodilator therapy. The etiology of BOS is not fully understood. It is believed that immunomodulatory and inflammatory mechanisms play roles in its development. This is supported by an association between BOS and cGVHD. BOS has been associated most firmly with cGVHD, although associations with viral infections prior to day +100 and gastroesophageal reflux have also been reported. Pulmonary function tests reveal an obstructive pattern with reduction in the forced expiratory volume in 1 second (FEV 1 ) and ratio of FEV 1 to forced vital capacity (FVC). High-resolution computed tomographic examinations with inspiratory and expiratory views have been shown to be sensitive and specific indicators of BOS in HSCT patients. Characteristic findings include air trapping, a mosaic pattern of lung attenuation, and bronchiectasis. BOS is traditionally treated with immunosuppressive agents, with corticosteroids being first-line treatment, although corticosteroid-resistant disease is common and portends a poor prognosis. Azithromycin has been shown to be a useful adjunct to customary immunosuppression in some patients. Published mortality rates for BOS after HSCT range between 21% and 100%. The prognosis after developing BOS depends in part on the tempo of the disease and the response to first-line therapy. Rapid onset BOS and lack of response to first-line immunosuppression are poor prognostic indicators.
Restrictive and diffusion abnormalities have been reported in pediatric patients after SCT. These findings improved over time but do not normalize. Risk factors for restrictive lung disease include sclerodermatous type GVHD, single-fraction TBI, abnormal chest wall growth due to radiation, and certain chemotherapeutic agents. Treatment depends on the underlying cause of the pulmonary disease. Because restrictive changes can be asymptomatic, assessment of the frequency of this complication depends on the intensity of post-SCT follow-up. Pulmonary compromise can influence the morbidity and mortality of survivors of HSCT. When developmentally appropriate, all HSCT recipients should have pulmonary function testing at 1-year post transplant. Providers should have a low threshold for screening patients who have risk factors for pulmonary complications earlier and more frequently. High-resolution chest tomography should be considered in high-risk patients who are unable to successfully complete pulmonary function testing.
Hematologic Complications
Patients who undergo ABO-incompatible allogeneic HSCT are at risk for immune hemolytic anemias. In the setting of major ABO incompatibility (e.g., recipient O and donor A or B), preexisting patient isohemagglutinins can cause lysis of donor red cells. This can potentially cause an acute hemolytic reaction during stem cell infusion, and thus anti-A or anti-B isoagglutinins must be removed from the patient before infusion via pheresis or the stem cell product must undergo red cell depletion. Although the acute issues surrounding transplantation in the setting of major ABO incompatibility are easily managed and not associated with any impact on outcome, pure red cell aplasia may develop in up to a fourth of at-risk patients in the months after HSCT. This occurs when long-lived patient lymphocytes continue to produce antibodies capable of destroying red cell precursors in the graft. Patients who are type O and donors who are type A are at greatest risk, presumably related to the level of isohemagglutinin titers. A relationship of red cell aplasia to posttransplant immunosuppression with cyclosporine alone has been suggested because calcineurin inhibitors permit longer persistence of patient B cells than methotrexate does. Some cases of aplasia will resolve spontaneously over time; plasma exchange with donor-type plasma replacement has also been used, and, more recently, rituximab, a chimeric anti–B-cell antibody, has been used with success. Minor ABO incompatibility (e.g., patient A, donor O) is managed by plasma depletion of the graft before infusion. This situation can also lead to low-level hemolysis in the first months after HSCT as newly formed B lymphocytes produce isohemagglutinins targeting residual patient red cells. Occasionally, in the months to years after transplantation, autoimmune hemolytic anemia, autoimmune thrombocytopenia, or autoimmune neutropenia develops as a result of immune dysregulation after HSCT. Corticosteroids and intravenous immune globulin are usually the first therapeutic intervention, but rituximab has been used in this setting as well.
Renal Complications
Chronic kidney disease (CKD) is a common complication in long-term survivors of HSCT, occurring in 20% to 60% of adult transplant recipients and as many as 62% of pediatric HSCT survivors. The incidence of chronic nephropathy is probably underestimated. Up to 25% of adults undergoing allogeneic HSCT were affected at 10 years; posttransplant hypertension was the most significant predictor of CKD. Assessment of renal function by determination of the glomerular filtration rate revealed renal impairment in 41% of pediatric patients 1 year after HSCT and in 11% at 7 years, with exposure to TBI being the most significant risk factor. HSCT survivors with CKD can progress to end-stage renal disease (ESRD). Survivors of HCT who develop ESRD have decreased survival compared with other patients with ESRD who did not did not undergo HCT. Factors that contribute to CKD and ESRD may begin during the acute transplant period.
The long-term impact of transplantation on renal function in pediatric patients is not known, but given the natural decline in renal function with age, monitoring function over time should be part of routine care for survivors of pediatric HSCT.
It has been demonstrated that the presence of proteinuria, a marker of nephropathy, at transplant day 100 increases the risk for nonrelapse mortality by as great as sixfold by 1 year after HSCT. Albuminuria is considered to be a marker of endothelial dysfunction and/or inflammation. It is postulated that albuminuria reflects a systemic, generalized endothelial injury and is associated with increased risk for nonrelapse mortality in the first transplant year. Albuminuria has been identified as a risk factor for cardiovascular and noncardiovascular mortality in the general and other patient populations. However, little is known about risk factors for CKD progression or why CKD and proteinuria increase nonrelapse mortality in the HSCT patient population. As this is explored in prospective studies it is suggested that children with albuminuria are considered for referral to a nephrologist experienced with posttransplant care.
HSCT-associated thrombotic microangiopathy including hemolytic uremic syndrome and thrombotic thrombocytopenic purpura are complications that occur in 25% to 35% of HSCT. These entities are characterized by vascular endothelial cell injury resulting in microangiopathic hemolytic anemia, thrombocytopenia, fibrin deposition in the microcirculation, and tissue damage. Hemolytic uremic syndrome may develop at a median of 5 months after HSCT. This disorder is generally manifested as moderate hemolysis and renal dysfunction, although some patients have more aggressive findings consisting of severe hypertension and seizures. Factors implicated in the development of this syndrome include exposure to radiation and use of calcineurin inhibitors. The majority of reported cases appear to resolve spontaneously over time, although modest persistent anemia and decrements in renal function have been described. Thrombotic thrombocytopenic purpura is rare in pediatric patients and may result from more pervasive endothelial damage than seen in hemolytic uremic syndrome. It usually occurs in the first months after HSCT and can have associated fever, bloody diarrhea, and neurologic symptoms. Unlike classic thrombotic thrombocytopenic purpura, HSCT-associated thrombotic thrombocytopenic purpura is not accompanied by deficient von Willebrand multimer proteolysis and plasma exchange therapy is generally unsuccessful.
Neurologic Complications
Patients undergoing HSCT may experience a variety of neurologic complications either directly related to treatment or the underlying diagnosis, as a consequence of immunosuppression, or as a result of medications used in supportive care.
Neurologic side effects are well described when cyclosporine and tacrolimus are used for GVHD prophylaxis. Commonly described side effects include essential tremor and paresthesias, both of which typically resolve when the dose is decreased or formulation changed. Seizures, visual disturbances, and encephalopathy are more significant but rarer neurologic complications. Posterior leukoencephalopathy is a syndrome associated with seizure, transient blindness, visual hallucinations, and motor deficits. It has been postulated that both seizures and acute visual loss arise from vasogenic edema accompanying cyclosporine-associated hypertension. Hypomagnesemia and elevated calcineurin inhibitor levels can be aggravating factors. These changes affect white matter, most commonly in the occipital area, and are accompanied by characteristic radiologic findings on magnetic resonance imaging (MRI). In general, symptoms abate rapidly on discontinuation or decreases in the dose of cyclosporine. A rare, severe complication of cyclosporine is manifested by parkinsonian motor features, ataxia, and/or quadriparesis. These changes may improve with discontinuation of the medication or may be permanent. There are fewer neurologic side effects reported for corticosteroids. However, mood swings or even frank psychosis accompanying corticosteroid therapy are well described.
Acyclovir is used for both prophylaxis and treatment of infection with herpes simplex viruses. Confusion, tremors, delusion, and psychosis have been associated with this drug. Similarly, mental status changes, paresthesias, and, uncommonly, seizures have been reported with the antiviral agents ganciclovir and foscarnet. Impaired renal function, often seen in HSCT patients, may potentiate the occurrence of these symptoms.
Cerebrovascular accidents, including cerebral hemorrhage, may also be observed after SCT and are usually associated with refractory thrombocytopenia. Large intracranial hemorrhages are generally fatal; patients with isolated subdural hematomas typically do well.
Central nervous system (CNS) infectious complications related to immunosuppression, cGVHD, or both can arise after HSCT. The greatest risk for CNS infection is between the second and sixth months after transplantation. The manifestations of CNS infection can differ from those in an immunocompetent patient in that localizing signs and symptoms may be less dramatic. Autopsy series demonstrate that up to 15% of necropsy patients harbor CNS infections, often clinically unsuspected. Aspergillus species and Toxoplasma gondii are the most common organisms found. Bacterial meningitis or encephalitis/meningoencephalitis caused by a variety of viruses, including CMV, herpes simplex types 1 and 6, adenovirus, and varicella-zoster, can also occur. Routine antiviral prophylaxis and CMV screening and treatment of reactivation have reduced the incidence of viral CNS infections. Opportunistic CNS infections in HSCT recipients are often fatal, and a high level of suspicion is warranted in patients with even minimal neurologic symptoms.
Leukoencephalopathy is a poorly understood sequela of HSCT that is manifested as progressive neurologic deterioration in the months after transplantation. Clinical findings include lethargy, slurred speech, ataxia, seizures, decreased sensorium, dysphagia, spasticity, or even decerebrate posturing. Consistent white matter changes are found on MRI. In a series of 415 patients from Seattle, leukoencephalopathy was observed in 7% of patients, but only in those who had received CNS radiation, intrathecal therapy, or both before HSCT in conjunction with intrathecal methotrexate after HSCT. More recently, a 4% incidence of irreversible leukoencephalopathy was reported in children receiving cyclosporine; a third of the patients died during the period of neurologic decline. Cyclosporine levels were usually therapeutic in the affected patients, and it remains unclear what the risk factors are for this rare but devastating complication.
CNS involvement in GVHD is highly debated. There are case reports of potential clinical and pathologic cases of possible CNS GVHD involvement in the literature. The clinical presentation is variable and can include seizure, encephalopathy, and focal neurologic dysfunction. MRI may show infarction, leukoencephalopathy, and/or hemorrhages. Definitive diagnosis requires pathologic findings in the brain similar to GVHD changes in systemic organs. Systemic immunosuppression is the mainstay of treatment.
The effects of different conditioning regimens on neuropsychologic functioning have been incompletely investigated. In particular, analysis of very young patients is limited. Nonetheless, it is clear that a significant proportion of young children, including those younger than age 2 years, can undergo HSCT with chemotherapy and TBI and subsequently have normal neurologic development, perform well in areas such as sensorimotor and cognitive functioning, and have no decrement in academic achievement. Age at HSCT appears to be the most consistent risk factor predicting subsequent cognitive decline.
Endocrine Disorders
Much of the endocrine dysfunction after HSCT results from irradiation and is attenuated in both incidence and severity when radiation is fractionated or when non–radiation-containing conditioning regimens are used. Thyroid dysfunction is one of the most frequent late complications of HSCT reported in between 0% and 52% of transplant survivors. After fractionated TBI, the incidence of compensated hypothyroidism ranges from 13% to 39% and that of overt hypothyroidism is around 15%, with diagnosis occurring at a median of 4 years after HSCT. Patients treated with BU-based regimens have a lower, but still significant incidence of thyroid complications: 20% compensated and 9% overt hypothyroidism. Undergoing transplant when younger than age 10 years is a risk factor for thyroid dysfunction, suggesting that the developing thyroid has increased susceptibility to toxicity. Given that the incidence of thyroid dysfunction increases over time, clinical suspicion and yearly monitoring of thyroid function tests are an important part of long-term care for these patients.
Growth disturbance is a late effect specific to pediatric transplantation and is a common problem. However, the incidence of growth disturbance is unclear because multiple different definitions are used in the literature with published incidences between 20% and 82%. Linear growth after HSCT is affected by many factors: nutritional status, thyroid and/or growth hormone disturbance, previous therapy, particularly corticosteroids or cranial or spinal radiation therapy, age at transplantation, conditioning regimen, and development of cGVHD with associated long-term corticosteroid exposure. Growth is most disrupted in children who underwent cranial irradiation before HSCT, followed by a TBI-based conditioning regimen due to disturbance of the pituitary axis. This group of children is also at greatest risk for growth hormone (GH) deficiency. If the GH levels are deficient, GH replacement can have a positive impact on final height, particularly in children younger than 10 years at the time of HSCT. Therapy is not associated with an increase in relapse; however, a higher incidence of osteochondromas/exostosis was seen in GH-treated patients. Nonetheless, many children do not demonstrate GH deficiency, and the role of exogenous hormone in these children or in children older than 10 at the time of diagnosis of GH deficiency is not clear. Overall, the mean loss of height appears to be approximately 6 cm, as predicted by both genetic height and height at transplantation. Still, nearly 80% of those undergoing HSCT in childhood will achieve a height that is within 2 standard deviations of adult norms.
Gonadal failure after HSCT is common; predictors include previous gonadotoxic therapy and the preparative regimen used for transplantation. For example, children with aplastic anemia who receive CY as their only cytotoxic agent almost always progress normally through puberty, whereas those receiving a BU- or TBI-based ablative regimen are very likely to have delayed development. Thus these children must be carefully monitored by a pediatric endocrinologist as they approach adolescence so that hormone replacement can be appropriately administered. Females who are postpubertal at the time of HSCT also need to be evaluated for hormone replacement therapy. In addition, they are at risk for gynecologic complications, including reduced uterine size, decreased vaginal and cervical size, atrophic vulvovaginitis, introital stenosis, and loss of pubic hair. No comparable data regarding these complications exist for those prepubertal at the time of HSCT. In postpubertal males, HSCT with or without radiation-based conditioning often results in azoospermia, and sperm banking should be offered to all patients with sufficient spermatogenesis. Significant Leydig cell damage resulting in decreased testosterone and decreased testicular volume is less common. In both sexes, spontaneous recovery of gonadal function has occurred years after HSCT. Fertility resulting in liveborn infants has been reported for both male and female SCT patients, particularly after conditioning with CY alone. Successful pregnancies are much less common after BU- and TBI-based regimens, and there is an increased risk for spontaneous abortion, preterm labor, and delivery of low- or very-low-birth-weight infants. There does not, however, appear to be an increased incidence of congenital anomalies in the offspring. Concern regarding fertility is one of the major issues for HSCT survivors up to a decade after transplantation.
Bone Problems
The most prominent bony complications after HSCT are decreased bone mineral density (BMD) and osteonecrosis. HCT may result in decreased bone mineral density, which may lead to osteopenia and osteoporosis with associated increased risk for fracture, loss of independence, immobility, chronic pain, and an overall decreased quality of life. Because many patients are asymptomatic, the incidence of osteopenia/osteoporosis in children and the impact on future bone health are not well understood. Small series of pediatric patients indicate that up to 50% of survivors of childhood allogeneic HSCT will have abnormal BMD and thus have an excess risk for fractures and other skeletal complications as they age. The Bone Marrow Transplant Survivor Study reported a prevalence of osteoporosis of 9% in long-term survivors (median age 36.5 years) of HSCT for acute leukemia. This was significantly higher than the prevalence of 2.2% in the siblings of the transplant patients. Allogeneic transplant and female sex was associated with increased risk for osteoporosis. There are few reports of posttransplant BMD changes in children, and most are from small, single-center, and retrospective studies. The single prospective analysis of 29 children after HSCT demonstrated that most bone mineral loss occurs within the first 6 months after transplantation. Studies of adult HSCT patients demonstrate that posttransplant bone turnover is characterized by increased resorption and decreased bone formation and that alteration in BMD is preceded by changes in markers of bone turnover such as serum osteocalcin, serum alkaline phosphatase, and urine amino-terminal telopeptide (N-telopeptide, NTX). It is not known if these patterns are the same in children, and a detailed understanding of how bone mineral status changes during and after HSCT is lacking.
Children are at risk for decreased BMD after HSCT due to factors related to their diagnosis and treatment including the underlying disease, pre-HSCT therapy (especially with corticosteroids), conditioning chemotherapy and TBI, osteotoxic medications, and presence of and treatment for GVHD. Studies of adult patients have shown that the chemotherapy and radiation used in HSCT conditioning can mediate skeletal toxicity in two ways. First, damage can occur through direct insult to osteoprogenitor cells. Second, cytokine release during the initial weeks after HSCT can activate osteoclasts resulting in increased bone resorption. The RANK/RANKL/OPG pathway is believed to be the primary mediator of osteoclastic resorption after conditioning. Receptor activator of the nuclear factor-κB ligand (RANKL) and RANKL/OPG (osteoprotegerin) ratio increase after transplant-stimulating osteoclastogenesis.
Osteonecrosis or avascular necrosis, the death of a segment of bone, is a potentially debilitating manifestation of bone disruption and can result in significant pain or fractures. Corticosteroid use and the presence of cGVHD appear to be the major risk factors, and children older than age 10 years appear to be at more risk than younger children. Joints of the lower limb, particularly the hip, are most often involved, and up to half of patients ultimately require joint replacement. Radiation-induced bone abnormalities, including osteochondromas and metaphyseal growth abnormalities, can occur after TBI-containing transplant regimens; younger patients appear to be at greater risk. Childhood survivors of HSCT should be evaluated regularly to ensure that hormonal and nutritional status is optimized. The role of bisphosphonates in the treatment of these conditions is being investigated. Early data indicate that bisphosphonates can improve BMD, but long-term effects of this intervention in the pediatric population are unknown.
Clinical Applications of Hematopoietic Stem Cell Transplantation
Myelodysplasia/Juvenile Myelomonocytic Leukemia
The myelodysplastic syndromes (MDSs) are a heterogeneous group of clonal stem cell disorders that can progress to acute leukemia. These disorders are much more common in adults but also account for approximately 3% of childhood hematologic malignancies. This figure may be an underestimate because a subset of children presenting with de novo AML presumably had an antecedent MDS. The FAB classification system is based on the proportion of leukemic blasts in the marrow; most pediatric patients have either refractory anemia (RA), refractory anemia with excess blasts (RAEB), or RAEB in transformation (RAEB-T). Juvenile myelomonocytic leukemia (JMML), a rare disorder of infants and young children, shares similarities with MDS, and a new classification system has been proposed that allocates pediatric patients with clonal myeloproliferative diseases into three groups: MDS, JMML, and myeloid leukemia of Down syndrome. MDS and JMML are disorders arising from pluripotent stem cells, and thus allogeneic HSCT remains the only curative approach.
Even though patients with JMML are generally very young and should tolerate therapy, induction chemotherapy prior to HSCT is generally not recommended because remission is unlikely to be attained and toxicity is unexpectedly high. Thus proceeding straight to HSCT is the recommended course. A BU- versus TBI-based conditioning regimen has been associated with a lower rate of relapse. As transplant-related mortality has decreased, survival has improved, with recent consortium data reporting an approximately 50% 5-year DFS; relapse remains the greatest obstacle to cure. Cis -retinoic acid appears to decrease the spontaneous proliferation of leukemic progenitor cells in JMML as well as abrogate their selective hypersensitivity to GM-CSF. It is often given after HSCT as therapy for MRD, although efficacy has not been firmly established. A small subset of patients may exhibit a milder phenotype of the disease, and rare reports exist of improvement without specific intervention. RAS mutations may be more common in this group, or children may have had a viral illness mimicking JMML. The vast majority of children, however, exhibit persistent or progressive disease and should move expeditiously to HSCT once other diseases in the differential diagnosis have been excluded.
The role of induction therapy before HSCT for MDS is unclear and has never shown a convincing impact on outcome. Up to one half of patients can achieve remission after AML-directed therapy ; however, and this approach may decrease relapse in patients with increased blasts at presentation. Conditioning with both TBI- and BU-based regimens has been used, with most studies showing no difference in outcome, although some favor BU. Overall, the strongest predictor of survival is stage of disease. A child undergoing transplantation for RA/RAEB has an event-free survival (EFS) of around 70%; a patient with RAEB-T was five times more likely to experience relapse. Currently, approximately half of pediatric patients with MDSs can be cured, and thus allogeneic HSCT should be considered early in the disease course. A retrospective review of pediatric patients from a single institution demonstrated a 80% DFS for those receiving no induction therapy and going to HSCT within 140 days of diagnosis.
Acute Lymphoblastic Leukemia
Improvements in chemotherapy regimens and supportive care have resulted in a steady increase in the cure rate for ALL, and approximately 85% of children are now cured by current risk-adapted chemotherapy regimens. However, for patients with ALL who cannot be cured by standard-dose chemotherapy, HSCT remains the mainstay of treatment. Because HSCT can be very effective for patients with relapsed disease, there has been an increasing focus on identifying the clinical and biologic characteristics of patients who are at highest risk for relapse after standard-dose chemotherapy so that they can be referred for HSCT at the earliest possible point in their course before relapse occurs.
In newly diagnosed children with ALL, there are many clinical and biologic characteristics, referred to as “risk features,” that are used to adapt the duration and intensity of chemotherapeutic regimens ( Fig. 8-2 ). However, a smaller number of features are used to identify newly diagnosed patients for whom allogeneic SCT should be the standard approach once remission first remission has been achieved. Patients with leukemia marked by a cytogenetic abnormality resulting from a reciprocal translocation of chromosome 9 and 22 (i.e., t[9;22] ) known as Philadelphia chromosome–positive ALL (Ph+) have historically had a dismal prognosis when treated with standard-dose chemotherapy. For these patients, matched-sibling HSCT in first remission significantly improved survival. A large European cohort found a relative risk of 0.3 for relapse or any adverse event with transplantation versus conventional chemotherapy and a DFS of more than 60%. Currently, most patients with Ph+ ALL undergo transplant in first remission using the best available donor, with 5-year EFS of 50% after mismatched family or UD transplant. However, the treatment approach to Ph+ ALL has been evolving since the advent of tyrosine kinase inhibitor (TKI) therapy (see Chapter 51 ), and the current recommendations for pediatric Ph+ ALL in first remission now suggest treatment with a combination of intensive CT plus TKI. An emerging consensus is that HSCT should be reserved for newly diagnosed patients with Ph+ ALL who do not show molecular evidence of response.
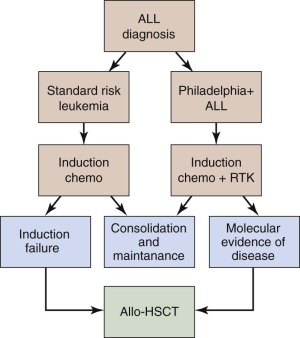
The role of transplant in first remission is less clear for other adverse cytogenetic abnormalities, including patients with t(4;11) translocation or hypodiploid ALL. Extreme hypodiploidy of less than 44 chromosomes is uncommon but results in an EFS of only 30% with standard chemotherapy. This has led to the inclusion of patients with hypodiploidy in trials of HSCT in first complete remission, although the numbers of hypodiploid cases are too small to make definitive statements about the impact of HSCT on outcome.
Historically, the outcome of patients with the T-cell phenotype of ALL has been worse than patients with precursor-B cell ALL, but those differences have largely been abrogated by the intensive chemotherapy used in modern protocols. However, an attempt is ongoing to define high-risk patients within this group, usually in those with a very high white blood cell count at diagnosis. Some reports suggest that the outcome for this subgroup may be improved by transplant in first remission, but this has not become standard of care in pediatric patients. It is not certain that the presence of a t(4;11) translocation is an adverse risk factor for children older than 1 year of age, but infants who harbor this translocation have an almost fivefold increase in the risk for an adverse event compared with infants without an MLL rearrangement. Even within this group further distinctions can be made: very young infants with MLL + ALL and a high white blood cell count at diagnosis have an EFS of less than 5%. An international study of infant leukemia found that a poor prednisone response in addition to the previously mentioned factors identified those at the highest risk. It is not definitively known if HSCT in first complete remission can improve the outcome of this group; however, single-center studies have reported an EFS of more than 60% and the current version of the international consortium trial recommends HSCT in first remission for the highest-risk group of infants.
Many studies have demonstrated the prognostic importance of the rapid clearance of leukemic cells in the peripheral blood or bone marrow during induction chemotherapy, and patients who have induction failure have a poor outcome. Historically, the presence of blasts was assessed histologically; however, more sensitive methods of flow-cytometric detection of leukemic blasts or polymerase chain reaction amplification of transcripts for clonal immunoglobulin, T-cell receptor, or oncogenic fusion genes has become standard. In a large European study, induction failure, defined as the persistence of leukemic blasts 4 to 6 weeks after induction chemotherapy, occurred in only 2.3% of newly diagnosed patients, but the overall prognosis for this group was poor, with a 10-year overall survival of 32%. In this study, patients with induction failure and either T-cell ALL, MLL rearrangement, or age greater than 10 years had a particularly poor outcome and a clear benefit of HSCT over chemotherapy was demonstrated. However, for younger patients (age <6 years) with B-cell phenotype ALL and no high-risk genetic features, no benefit of HSCT was shown.
In summary, it appears that ALL patients with some high-risk features at diagnosis have a decreased incidence of relapse and improved survival when taken to HSCT from a matched RD in first complete remission and that HSCT confers the greatest advantage in the patients at highest risk. However, it is likely that increasing numbers of targeted therapies for specific molecular subtypes of ALL will enter the early stages of therapy in the coming years, making the indications for HSCT in newly diagnosed ALL an evolving field.
Once ALL has recurred, the chance of cure drops dramatically. A landmark study in the late 1980s concluded that HSCT from a matched RD cured approximately 40% of children with relapsed ALL. In contrast, conventional chemotherapy resulted in subsequent relapse over 90% of the time, establishing HSCT as the mainstay of therapy for relapsed ALL. However, just as for newly diagnosed patients, clinical and biologic features strongly affect the likelihood of cure with HSCT versus further chemotherapy. The strongest predictors of outcome in patients with recurrent ALL are the duration of the first remission, T-cell phenotype, and the site(s) of relapse. These features are most commonly used to identify the patients with relapsed disease most likely to benefit from HSCT.
Patients who experience an early relapse (defined variably as relapse occurring on therapy or within 18 to 36 months from diagnosis) present one of the most difficult challenges in ALL therapy. It is clear that chemotherapy alone cannot cure these patients, and most studies show a survival advantage for sibling HSCT. However, the results for HSCT in patients with early relapse ALL are also poor: a report of children with a bone marrow relapse within 12 months of completing therapy found a 3-year EFS of approximately 25% regardless of treatment modality. Most of the earlier discussed studies have been with matched sibling donors; as outcomes with alternative donors have improved, equivalent results with UDs are now being reported. In summary, it has not been determined which patients with ALL in the second complete remission conclusively benefit from allogeneic HSCT. Patients with late isolated extramedullary relapses should not undergo transplantation in most circumstances. For the remaining groups there are no prospective randomized trials to guide therapy.
Some children are unable to tolerate the months of intensive therapy given in relapse protocols and then should be considered for HSCT, because most studies reveal a lower incidence of relapse with transplant that often but not always translates into an improved DFS. The risks and benefits of transplant must therefore be carefully considered in each situation, balancing type and match of available donor, medical condition of the patient, and patient and family preference.
Patients experiencing an isolated bone marrow relapse fare more poorly than those with either an isolated extramedullary relapse (usually involving the CNS or testes) or those with a combined extramedullary and bone marrow relapse. As a result, most children with an isolated extramedullary relapse occurring after chemotherapy has been completed are treated with intensive chemotherapy and irradiation to the site of relapse rather than allogeneic SCT. This strategy results in very good outcomes, with a recent consortium trial showing that approximately 75% of children in this situation can be cured using systemic and intrathecal chemotherapy and cranial irradiation.
Acute Myeloblastic Leukemia
Successive clinical trials over the past 30 years have firmly established the role of intensive postremission therapy in the treatment of AML. However, there remains debate as to whether allogeneic HSCT or intensive chemotherapy without stem cell support is the optimum postremission therapy. Allogeneic HSCT offers dose-intensity and the possibility of graft-versus-leukemia effect but is not an option for all patients and comes with heightened risks for acute and long-term toxicities. Intensive chemotherapy without stem cell support is feasible for most patients and may have less toxicity. A recent retrospective assessment of 233 children with cytogenetically defined high-risk AML did not demonstrate a survival difference between those who received chemotherapy alone and those who received allogeneic HSCT. In this study the risk for relapse was lower in the transplanted population and the transplant-related mortality rate was higher. The study included patients who underwent transplants between 1989 and 2006. It is likely that the transplant-related mortality is lower in the modern era owing to improvements in supportive care. It is possible that the reduced risk for relapse after transplant may outweigh the risk for transplant-related mortality in the modern era.
Allogeneic HSCT in first complete remission was first addressed in a series of prospective trials in the mid-1980s. In 111 consecutive patients, the Seattle group undertook matched sibling allogeneic HSCT for patients who had a donor whereas the remaining patients received conventional intensification. The probability of 5-year DFS was 49% for HSCT compared with 20% for chemotherapy alone. However, since that time, more intensive postremission chemotherapeutic regimens have been associated with improved disease control in children and adults with AML. This has again raised the question of whether allogeneic HSCT is more effective than intensive chemotherapy in the postremission setting. To address this issue the Children’s Cancer Study Group conducted a large, randomized trial of allogeneic HSCT versus chemotherapy or autologous HSCT in 652 children and adolescents with AML who achieved remission. At the end of induction, patients with five or six HLA-antigen matched relatives were allocated to allogeneic HSCT. All others were randomized between autologous HSCT with 4-hydroperoxycyclophosphamide (4-HC) purging or four courses of chemotherapy. The results of this trial showed that the outcome with allogeneic HSCT was superior to that of either chemotherapy or autologous HSCT. For patients treated with an intensive induction regimen, the DFS with allogeneic HSCT was 66%, compared with 53% with chemotherapy 48% with autologous HSCT. These data underscore the benefit both of intensive induction chemotherapy and of first-remission matched sibling donor HSCT in the management of newly diagnosed AML for many patients.
Studies have attempted to identify groups of patients that are at increased risk for poor outcomes with chemotherapy alone in first complete remission for which HSCT would be beneficial. Investigators have shown that allogeneic transplant in first complete remission in patients can improve the outcome for children with high-risk prognostic features ( Fig. 8-3 ). In the pediatric population, consistent prognostic indicators are the response to initial induction therapy and cytogenetic markers. Additionally, the presence of MRD before transplant has gained prognostic importance. The Medical Research Council (MRC) AML-10 trial found 5-year survival rates of 67%, 65%, and 23% in patients who achieved complete remission, partial remission (5% to 15% blasts), and had residual disease, respectively. The relapse rates in these groups were 34%, 38%, and 87%. This and other studies stratified patients based on favorable, intermediate, and adverse karyotypic abnormalities. Favorable karyotypic abnormalities include t(8;21), inv(16), t(15;17), and, in some studies, t(9;11) and t(16;16). Adverse abnormalities include −5, −7, del(5q), abn(3q), and complex karyotypes. Patients who have neither favorable nor high risk are considered of intermediate risk. In the MRC AML-10 trial, patients with favorable and intermediate karyotypic abnormalities had survival rates of 76% and 52% with relapse rates of 34% and 44%. This was compared with survival and relapse rates of 40% and 61% in patients with adverse karyotypes. The MRC further designated risk groups of good, standard, and poor risk in the MRC AML-10 trial. The good-risk group included patients with favorable karyotypes or FAB type M3, irrespective of response status after one course of chemotherapy or the presence of other karyotypic abnormalities. The standard-risk group included patients in neither the good- nor poor-risk groups. The poor-risk group included patients with adverse karyotypic abnormalities or with resistant disease in the absence of favorable karyotypic abnormalities and not FAB type M3. There was no significant difference in the percentage of deaths during first complete remission between any of the groups. There were significant differences between the good, standard, and poor groups in regard to relapse-free survival (65%, 62%, and 36%), DFS (59%, 54%, and 32%), survival from relapse (61%, 17%, and 0%), and 7-year survival from complete remission (78%, 60%, and 33%). The 61% salvage rate after relapse in the good prognosis group suggested that HSCT in the first complete remission may be inappropriate for this group. Although not assessed in the MRC trial, patients with an activating FLT3 mutation have been shown to have a poor prognosis and benefit from HSCT in the first complete remission.
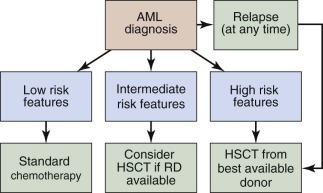
Several studies have suggested that the presence of high MRD at any point before HSCT leads to increased relapse rate. Researchers at the Fred Hutchinson Cancer Research Center studied 253 patients and found that patients with even small levels of MRD before allogeneic myeloablative HSCT has a negative impact on overall survival in patients transplanted in both first and second complete remissions. Rosenberg and associates investigated the impact of MRD on survival in 89 pediatric patients after myeloablative allogeneic transplant. The 3-year overall survival for patients with MRD positivity before transplant was 15%, compared with 67% in children without MRD. A prospective multicenter study is currently investigating the impact of MRD assessed before and at two time points after transplantation.
Autologous HSCT has been studied for patients who have no allogeneic donor. In many trials, autologous marrow was purged of residual leukemic cells with 4-HC, although no direct benefit of this technique has been proven. Multiple trials in adults and adolescents with AML suggest that autologous HSCT might offer additional therapeutic efficacy over chemotherapy. Pediatric trials and a recent meta-analysis have not demonstrated any advantage to this modality in patients in first complete remission, and there are insufficient data to assess the influence of AML subclass on outcome.
In most studies of patients with relapsed AML, the duration of first remission is strongly predictive of outcome, with the DFS of patients experiencing relapse less than 1 year after diagnosis 10% to 20% regardless of treatment modality used. Early studies reported DFS of patients transplanted in untreated first (“early”) relapse to be as high as 25% to 30%. In contrast, a more recent report of 379 adults with relapsed or refractory AML found the DFS of patients transplanted in untreated first relapse to be no better than 13%. Patients with treatment-refractory disease had an equivalently poor prognosis. However, the DFS for patients who were are able to go into a second remission was significantly better at 32%. The outcome of children with relapsed AML was studied by the MRC Childhood Leukemia Working Party. Of the 81 patients in whom curative therapy was attempted, 69% went into a second remission. Approximately two thirds of these patients were consolidated with allogeneic HSCT, and the overall survival of the group was 44% at 3 years. Although this was not a randomized study, patients treated with HSCT had a longer duration of second remission than those treated with chemotherapy alone. These data support the use of allogeneic HSCT in the treatment of relapsed AML, especially for those patients who can achieve a second remission.
Chronic Myelogenous Leukemia
CML is a proliferative stem cell disorder arising from a translocation between chromosomes 9 and 22 (t(9;22) ) known as the Philadelphia chromosome. The only curative therapy for this condition is allogeneic HSCT. However, with the advent of targeted molecular therapies such as imatinib, a TKI, daily administration of an oral agent can control the disease for years, if not longer, with minimal side effects. As a result, the role of HSCT with the attendant short- and long-term toxicities is being redefined. The majority of the literature on both HSCT and TKI therapy reflects studies in adults in which current practice is to use TKI as initial therapy in virtually all patients presenting with chronic-phase CML. A French prospective trial has shown that imatinib has similar efficacy in the pediatric population. However, the risk-benefit profile remains different in the pediatric versus adult population; the results of transplant are better than in adults owing to both decreased transplant-related mortality and GVHD and the implications for both compliance and toxicity of decades of daily therapy are not yet well understood. In the largest pediatric transplant series to date patients receiving either matched sibling donor (n = 182) or UD (n = 132), HSCT had a 63% and 56% DFS, respectively. The best outcome was observed in those going to transplant in the first chronic phase and within 6 months of diagnosis; DFS with a sibling donor for that cohort was 73%. Given these data and newer studies showing outcomes to be virtually identical with sibling donors or UDs for pediatric leukemia patients, it still may be reasonable to offer HSCT as the first therapeutic intervention for select pediatric patients with either a sibling donor or fully matched UD. However, the most common approach is to begin TKI therapy at the time of diagnosis with careful monitoring of disease response. Robust data now allow response to imatinib at specific time points to be categorized as “failures,” thus identifying patients who need alternative therapies. In young patients, whether the next intervention should be allogeneic HSCT or a trial of second-generation TKIs depends on donor availability and physician and family and patient assessment of the risks and benefits attendant on both strategies. However, given the paucity of efficacy and safety data on second-generation TKIs in the pediatric population, proceeding to allogeneic HSCT is currently the most conservative approach. The rare patients who present in accelerated phase or myeloid or lymphoid blast crisis of CML have a much worse prognosis and require lymphoid- or myeloid-directed chemotherapy to try to attain a chronic phase followed by HSCT. Allogeneic HSCT historically offered curative therapy for approximately 40% of such patients. The addition of TKI therapy both before and after HSCT has favorably impacted relapse in early studies.
For those patients undergoing HSCT, careful monitoring of disease status remains an essential component of posttransplant care. Relapse detected when disease is confined to cytogenetic or molecular positivity responds extraordinarily well to infusions of donor lymphocytes (DLI). Currently, most patients receive escalating doses of CD3+ T cells in an attempt to achieve disease control without inducing GVHD. TKI administration for post-HSCT recurrences—either alone or in combination with infusions of donor lymphocytes—is still being evaluated but seems to be generally well tolerated and may favorably impact outcome.
Hodgkin and Non-Hodgkin Lymphoma
Autologous HSCT has been utilized in children with Hodgkin disease (HD) or aggressive non-Hodgkin lymphoma (NHL) in the setting of refractory or recurrent disease after conventional therapy. Both TBI-based and chemotherapy-only approaches have been used with equal success. Autologous PBSCs as compared with bone marrow are the preferred stem cell source based on earlier engraftment for both neutrophils and platelets. The ability of high-dose therapy with autologous rescue to salvage pediatric patients with Burkitt lymphoma was established in 1986, when a 5-year French experience demonstrated approximately 50% DFS. Similar results in children with Burkitt and large cell lymphoma as well as HD have been reported more recently from other European centers and in larger combined pediatric and adult series. Paralleling the findings in adult patients with lymphoma, the DFS is most consistently affected by the presence of chemotherapy-sensitive disease before HSCT; long-term survival is unlikely in those with resistant bulky disease at the time of HSCT. Length of first remission (< or >12 months) also appears to impact survival. The addition of rituximab before and/or after HSCT for B-cell lymphomas may further improve outcome in this group of patients, although a more recent study of almost 500 adult patients failed to show a decrease in relapse with the addition of rituximab maintenance therapy after autologous HSCT. Refractory or relapsed T-cell lymphoblastic lymphoma is treated identically to T-cell ALL, and allogeneic HSCT from the best available donor is the standard approach; achieving remission with salvage therapy is essential to have the potential for cure.
There has been long-standing interest in the role of allogeneic BMT in the treatment of relapsed/refractory NHL and HD, although the balance of risk and benefit is still frustratingly unclear because most series show a decreased rate of relapse but increased transplant-related mortality. Retrospective studies in adult/pediatric series suggest that ultimately the results are comparable. A prospective trial confirmed lower relapse rates in allogeneic BMT but no overall survival advantage, owing to significant transplant-related mortality. As seen in patients undergoing autologous HSCT, chemosensitivity before the transplant procedure was the major prognostic factor affecting outcome. The advantage conferred by an allogeneic stem cell source may be dependent on the type of lymphoma; a recent study of pediatric patients with anaplastic large cell lymphoma reported a remarkable 75% DFS, with successes even in patients experiencing relapse after autologous HSCT. Whether the decrease in relapse rate reported in these studies reflects a graft-versus-lymphoma effect or merely the infusion of uncontaminated HSCs is not clear. The introduction of reduced-intensity approaches can decrease transplant-related mortality, but this is often at the expense of an increased incidence of relapse. Characterization of patients at particularly high risk for failure should allow better delineation of which patients could benefit from an allogeneic transplant—either alone or as a tandem approach after autologous transplant —despite the increase in transplant-related mortality and the risk for cGVHD.
Neuroblastoma and Other Solid Tumors
The biology and treatment of pediatric solid tumors are discussed elsewhere in this text (see Section XV). The most extensive HSCT experience in pediatric solid tumors is in patients with advanced-stage neuroblastoma. Historically, patients receiving conventional doses of chemotherapy almost always succumbed to disease. In 1999, a landmark trial showed a significant improvement in DFS (34% vs. 22%) for patients randomized to consolidation with autologous BMT compared with continued intensive nonmyeloablative chemotherapy. Regimen-related toxicity was acceptable, and dose intensification appeared to impact DFS; thus sequential or “tandem” transplants were investigated utilizing two or three cycles of non–cross-resistant agents over a short period of time with autologous PBSC support after each cycle. It appears that such approaches can further improve outcome, with reported 3-year DFS of 58% and an estimated DFS of 45% at 7 years from diagnosis. Relapse remains a significant problem primarily at diffuse osseous sites. Neuroblastoma cells marked in the stem cell product have been identified at sites of relapse, and a variety of positive and negative selection techniques have been employed to purge the stem cells before reinfusion, although no technique has been definitively shown to impact outcome; profound depletion of T cells from the graft has, however, resulted in an increase in life-threatening viral infections. Current efforts have focused on selecting a conditioning regimen that provides the greatest additive effect for disease control. Early results from a European randomized trial suggested that the incidence of relapse was lower in children receiving a BU/melphalan approach as compared with the carboplatin-based approach used in the United States. The ongoing national Children’s Oncology Group trial is evaluating this regimen. There are insufficient data to address efficacy; toxicity has been acceptable, although the incidence of severe VOD appears to be increased. There has been long-standing interest in the role of allogeneic transplant for high-risk neuroblastoma. A retrospective study from the international registry did not demonstrate any impact on relapse rate or survival.
Therapy for MRD after HSCT is essential. The use of cis -retinoic acid as a differentiating agent adds minimal toxicity and favorably impacts relapse. A recent randomized trial unequivocally demonstrated the role of anti-GD2 antibody therapy after HSCT with significant improvement in both overall survival and DFS. The trial met criteria for early stopping owing to demonstrated efficacy, and the ethical issues surrounding this relatively rare event are nicely reviewed by Unguru and colleagues.
Most children undergoing autologous HSCT for neuroblastoma are very young, and there have been concerns about their quality of life after this intensive therapy. However, it appears that most survivors of this previously almost uniformly fatal disease are neuropsychologically intact. Survivors are at risk for a variety of medical complications, the most common being short stature, gonadal failure, and hearing loss (seen in three fourths of patients) and must be closely followed by appropriate specialists to optimize outcome.
The role of high-dose therapy with autologous PBSC rescue for brain tumors is still being evaluated. The literature is most extensive for those tumors with a clear chemotherapy dose-response curve such as medulloblastoma/primitive neuroectodermal tumor and germ cell tumors. HSCT is most often employed in the setting of recurrent disease but is occasionally used at the time of first remission in patients with adverse prognostic features or in very young children in an attempt to avoid radiation therapy. The EFS ranges from 25% to 60%; and, as is the case for most conditions treated with autologous HSCT, those with no detectable disease at the time of transplant fare best. Ongoing consortium trials hope to standardize therapy and better delineate which patients can benefit most from this therapeutic approach.
Small single-institutional series have been published on the use of either allogeneic or autologous HSCT for other nonhematologic malignancies. Some promise in terms of response rate has been observed for patients with sarcomas who have both MRD and chemosensitive disease, but the generalizability and durability of these responses will need to be established before widespread application can be supported.
Immunodeficiency Disorders
Since the late 1960s, allogeneic BMT has been successfully employed to cure children with a number of lethal immunodeficiency diseases, including severe combined immunodeficiency disease (SCID) and Wiskott-Aldrich syndrome.
Severe Combined Immunodeficiency Syndromes.
SCID is a clinical syndrome arising from a variety of genetic abnormalities that all result in profound deficiencies in T-cell function. At least 18 types of SCID have been identified that vary in the affected gene but share as a common feature a profound effect in T-cell development or function. Differences in the genetic basis of SCID results in a spectrum of abnormalities that may also affect B cell and NK cell number and function. In classic X-linked SCID there is profound T-cell lymphopenia and a variable number of nonfunctioning B cells whereas patients with adenosine deaminase deficiency lack B cells, T cells, and NK cells. Autosomal recessive SCID phenotypes have different combinations of B-cell and NK-cell presence.
Without effective therapy, the outcome of SCID is invariably fatal and HSCT has become the mainstay of therapy for most types of SCID, with long-term survival as high as 90% of patients. Unlike most transplant settings, owing to the underlying immunologic defect, SCID patients with a matched RD do not necessarily require conditioning. After infusion of genotypically matched donor HSCs without cytoreduction, immune reconstitution will take place within several weeks and evidence of good lymphocyte function will be evident by 2 to 3 months. Significant aGVHD or cGVHD is uncommon. Dramatically improved long-term survivorship from the range of approximately 50% in the 1970s to over 90% currently appears attributable to more rapid and precise diagnosis, followed by transplant of patients at a younger age and in better condition, and to improved supportive care for the associated infectious complications.
The optimal approach for children lacking a matched sibling donor is less clear, and two different strategies have been employed: T-cell–depleted haploidentical transplant usually using a parent as the donor or transplant from a matched UD or UCB unit. Early experience with haploidentical HSCT was hampered by graft rejection, frequent severe GVHD, and opportunistic infection, resulting in poor long-term survival. The advantage of this approach is that neither cytoreductive therapy nor GVHD prophylaxis is needed and thus peritransplant mortality is low. However T-cell function usually takes several months to appear, patients generally do not have B-cell function mandating lifelong intravenous immune globulin replacement, and graft rejection necessitating a second or even third transplant occurs in approximately 20% of patients. T-cell function normalized and was most robust in children diagnosed early and undergoing transplant within the first 28 days of life, thus increasing the importance of screening initiatives to diagnose SCID in the neonatal period.
Given the concerns about haploidentical transplantation, there is increasing interest in using of matched UDs as a stem cell source for those patients lacking a matched sibling donor. Although cytoreductive conditioning is required with this approach, it may allow the more rapid reconstitution of a fully-functional donor immune and hematopoietic system. These advantages must be weighed against the time taken to identify a UD and the risk for GVHD, the increased risk for acute infectious morbidity and mortality associated with myeloablative therapy, and the long-term sequelae, including the likelihood of infertility. Recent reports indicate that use of a UD confers a survival advantage compared with mismatched RDs (80% vs. 52%) as well as improved reconstitution of a full T-cell repertoire. The role of nonmyeloablative HSCT and the ability of this approach to decrease acute toxicity and mitigate long-term sequelae is being investigated but may be a particularly attractive option in children with severe organ dysfunction at the time of HSCT.
Wiskott-Aldrich Syndrome.
Wiskott-Aldrich syndrome is an X-linked disorder in which progressive T-cell immunodeficiency is associated with eczema, small platelets, and absolute thrombocytopenia. The pathogenesis of this disorder is associated with aberrant expression of the WAS protein, which is involved in cytoskeletal organization as well as T- and NK-cell activation. (See Chapter 24 .) Both TBI-containing and BU-based regimens have successfully been used in conditioning patients with Wiskott-Aldrich syndrome, and the reported survival rates of almost 80% to 90% in patients are among the best of any patient cohort undergoing allogeneic SCT. A recent collaborative study demonstrated 84% survival in 194 patients with Wiskott-Aldrich syndrome treated with HSCT, although almost a fourth of patients did not have full donor chimerism at a year, suggesting that additional improvements in outcome may accrue from optimized conditioning regimens. Thus most children afflicted with Wiskott-Aldrich syndrome are now expeditiously referred for transplantation using the best available donor, with a palliative approach including splenectomy and chronic intravenous administration of immune globulin being utilized only in rare cases.
In summary, outcomes for patients with primary immunodeficiencies treated with HSCT are improving, as a result of better donor selection, optimal supportive care, and early diagnosis, but the field is still an evolving one, and the relative roles for HSCT from different donor sources—and the use of gene therapy for some diseases—are likely to change with time.
Hemoglobinopathies
Sickle Cell Disease.
Allogeneic HSCT offers a curative approach for patients with sickle cell disease (SCD). Because the clinical course of SCD is highly variable and, particularly with the advent of pneumococcal prophylaxis, childhood morbidity and mortality is low, HSCT as a treatment modality has been relatively slow to gain widespread acceptance. Initial studies offered HSCT to the subgroup of patients with the most significant symptoms: stroke, recurrent acute chest syndrome, or frequent painful crises. In the seminal report by Walters, DFS after BU-based myeloablative conditioning was 73% and overall survival was 91% at 4 years; end organ damage was stabilized in patients with sustained donor engraftment. Improved management of transplant complications in general and increased attention to SCD specific toxicities (e.g., additional immunosuppression to decrease graft rejection, antiseizure prophylaxis, strict management of hypertension) have resulted in consistent gains in cure rates, with EFS of more than 95% in patients undergoing a transplant after January 2000. Meticulous long-term assessment of these patients has demonstrated that successful engraftment will protect against progressive pulmonary and CNS disease but significant gonadal toxicity is sustained. Newer approaches such as the use of hydroxyurea to decrease the risk for graft rejection by impacting pretransplant bone marrow cellularity or the use of a nonmyeloablative approach to decrease transplant-related morbidity and mortality or even preserve fertility require both larger numbers of patients and longer follow-up before being considered as a standard approach. Early results suggest that reduced-intensity conditioning results in mixed chimerism for most patients but is associated with sufficient erythroid engraftment to reverse the sickle phenotype. HSCT has historically been considered only for patients who have an available matched sibling donor. A multiinstitutional survey of factors impacting the use of HSCT in this population found that less than 15% of affected children had an acceptable sibling donor. The role of UD HSCT in patients with the most severe manifestations of disease or in those unable to receive long-term transfusion therapy is unknown, but multiinstitutional trials are currently ongoing with a reduced-intensity conditioning platform. The use of unrelated UCB is a theoretically attractive option given the decreased incidence of GVHD and the ability to identify acceptable donors for patients with less common HLA typing by tolerating greater HLA disparity. Small numbers of transplants have been reported with successful outcomes, but graft rejection remains an obstacle to widespread use and resulted in early closure of the UCB arm of the ongoing national trial.
The choice to proceed to matched-sibling HSCT remains complicated, particularly if complications of the disease are moderate or intermittent. There are no prospective randomized trials to inform the decision and therapy with hydroxyurea, chronic transfusions and HSCT each have associated risks and benefits. HSCT clearly has the highest upfront risk but also offers the only curative modality. The Belgian experience illustrates that children undergoing transplants at a young age before significant complications occur do best, with a DFS of 93% and overall survival of 100%, and serious manifestations of SCD impacting on long-term survival such as pulmonary hypertension continue to be identified. Unfortunately, patients undergoing HSCT and who are cured of SCD remain at risk for complications from the transplant process: infertility is likely, and the development of extensive cGVHD is a rare but potentially devastating possibility. Patients (when of an appropriate age) and parents must be aware of the possible therapeutic options, thus allowing them to be educated and active participants in the decision-making process.
Thalassemia.
Thalassemia major (or Cooley anemia) is the most common transfusion-dependent anemia in the world. Chronic transfusion therapy and chelation to prevent the associated iron overload have modified the natural history of thalassemia and transformed a diagnosis that was uniformly fatal in early childhood into a chronic disease that is usually manageable, although progressive end organ dysfunction still develops. Over time, even medically compliant patients are at risk for complications, including endocrine dysfunction, hepatic cirrhosis, cardiac disease, and transfusion-associated viral infections. In the developed world with sophisticated medical management, life expectancy is 25 to 55 years; compliance with chelation therapy is the most important factor impacting survival. Although advances have been made in supportive care over the past decade with resulting increases in life expectancy, in the developing world the majority of patients still die before the third decade of life.
The successful application of HSCT as a curative approach for thalassemia major was first reported in 1982. Subsequently, a very large experience reported by Lucarelli and coworkers unquestionably established the feasibility of HSCT for thalassemia, and more than 3000 patients worldwide have now received transplants, with the overwhelming majority using a matched sibling donor and a BU-based conditioning regimen. The Pesaro classification system, based on the presence of hepatic fibrosis as assessed by liver biopsy, degree of hepatomegaly, and a history of inadequate chelation, allows risk stratification of potential transplant patients younger than 17 years of age: class I patients have no risk factors, class 2 patients have one or two risk factors, and class 3 patients have all risk factors. The risk factors used by Lucarelli and coworkers may be difficult to reproduce. The probabilities of overall survival, thalassemia-free survival (TFS), and graft rejection for patients undergoing HSCT from a matched family donor were 94%, 94%, and 0%, respectively for class I patients and 80%, 77%, and 9% for class 2 patients. Class 3 patients had only a 53% TFS owing to both increased graft rejection and transplant-related mortality. Newer approaches based on increased immunosuppression using hydroxyurea, azathioprine, and/or fludarabine have yielded improved results for class III patients, with a TFS of 80% in patients younger than age 17 years. Post-HSCT management of preexisting hepatic and cardiac iron overload and viral infections is essential for optimal outcome. Positive effects on bone mineral density in children may be seen after successful engraftment, and even reversal of cirrhosis has been reported. Impressively, a large study reporting on the quality of life of patients older than age 20 years after HSCT demonstrated that overall quality of life was identical to that of the general population for the majority of patients. Older age at HSCT and the presence of GVHD predicted a poorer quality of life. Interestingly, a matched cohort of thalassemic patients treated with transfusion/chelation reported a significantly worse quality of life when compared with transplanted patients.
Although matched sibling HSCT has been shown to provide curative therapy for more than 90% of patients, the decision to accept the short- and long-term risks associated with HSCT remains complex and should occur on an individual basis after consultation with both hematologists and transplant physicians. Historically, patients lacking a matched sibling donor were not offered the option of HSCT because transplants using alternative donors conferred a dismal prognosis in this disease. However, improvements in HLA typing, approaches to pre-HSCT preparative regimens, and supportive care have globally impacted transplant outcomes; thus alternative donor transplantation is now a reasonable option for selected patients. After mirroring results using sibling donors, it was shown that the best results occur in those with less-advanced disease, and a TFS of 80% to 100% for class I or II patients has been reported in small series. Progress has occurred in the use of alternative donors in patients with advanced-stage disease as well. A recent small study using mismatched RDs and a preparative regimen emphasizing immunosuppression achieved a TFS of 94%. A larger Italian study of 60 patients, two thirds of whom received transplants from UDs, employed a treosulfan-based preparative regimen and had a 5-year TFS of 84%, likely owing at least in part to the decreased hepatotoxicity of treosulfan compared with BU. There was no effect of Pesaro class or donor type (sibling vs. unrelated) on outcome.
Thalassemia is a major global health problem because it is now most prevalent in resource-poor countries, with more than 100,000 affected children born annually in the Middle East and Southeast Asia. These countries lack the infrastructure to provide safe transfusions and/or chelation therapy, and the majority of children die before reaching adulthood. The development of safe and affordable transplant strategies that can provide a cure for these patients is one approach to this important problem.
Osteopetrosis
Infantile malignant osteopetrosis is a rare disorder of osteoclast function that affects 1 : 200,000 to 1 : 300,000 children. Osteoblast function is preserved, and the osteoclast dysfunction leads to an inability to resorb and remodel bone. The resulting osteoblast-osteoclast imbalance results in the formation of excessive mineralized bone and cartilage. Infantile malignant osteopetrosis is inherited in an autosomal recessive or autosomal dominant manner and causes severe phenotypes. There is significant phenotypic variability dependent on the patient’s genetic mutation. Commonalities in all phenotypes are poor growth and bony encroachment on optic and other cranial nerve foramina causing vision and hearing impairment. Eradication of the marrow space ensues, with progressive pancytopenia, failure to thrive, and hepatosplenomegaly. In addition, patients with CLCN7 autosomal recessive mutations suffer from progressive neurodegeneration and do not benefit from HSCT. Murine studies revealed that osteoclasts arise from hematopoietic precursors of the monocytic lineage, and disease reversal was accomplished by engraftment of bone marrow from unaffected animals. Successful HSCT in humans was first reported in 1977, and its applicability has subsequently been shown in many reports mostly using myeloablative BU-based conditioning regimens. The European Group for Blood and Marrow Transplantation (EBMT) reported the long-term outcome of 122 children undergoing allogeneic HSCT between 1980 and 2001. The 5-year DFS rate was 73% in those receiving transplants from an HLA-identical sibling versus approximately 40% in those receiving transplants from mismatched family donors or matched UDs. The major causes of failure were lack of engraftment, attributed in part to disruption of the underlying marrow microenvironment, and pulmonary complications, particularly pulmonary hypertension. Conservation of vision was most likely in those who underwent transplantation before 3 months of age. Patients, especially those older than 2 years at transplantation, are at risk for hypercalcemia as engraftment occurs, and therefore meticulous monitoring of calcium levels is essential in the peritransplant period. At present, HSCT is the only curative option, although calcitriol, interferon-γ, and prednisone have been used to slow progression, ameliorate symptoms, or both. Donor identification and subsequent HSCT should occur expeditiously as soon as the diagnosis is confirmed. Unless an unaffected, matched sibling is readily available a UCB unit may be preferred because it can be obtained in a more expeditious manner compared with a UD search because neurologic impairment can occur rapidly and may be irreversible, even with successful hematopoietic engraftment.
Transplantation for Lysosomal and Perioxisomal Diseases
Allogeneic transplantation is a therapeutic option for children with select inherited lysosomal and perioxisomal disorders. These are rare diseases, and there are fewer than 100 transplants performed for these disorders annually in the United States. Two key principles for transplanting children with lysosomal and perioxisomal diseases are that in most cases neurologic function lost before HSCT cannot be restored and because of this children with advanced disease do not benefit from transplant.
The mucopolysaccharidoses (MPSs) are inherited diseases caused by gene defects that result in the deficiency of lysosomal enzymes needed to degrade glycosaminoglycans (GAGs). This causes cellular accumulation of GAGs and end organ tissue damage. Treatment of MPS can include therapy of organ-specific defects, enzyme replacement therapy when available, and HSCT. Enzyme replacement therapy is currently available for MPS types I, II, and VI, is typically given as monthly infusions, and can be complicated by infusional reactions. Enzyme replacement can improve the nonneurologic performance status and organ function but does not cross the blood-brain barrier and is ineffective for CNS disease.
The success of transplantation for the MPSs is based on correction of the enzyme deficiency, enzyme cross-correction, and the ability of hematopoietic cells to engraft and distribute as macrophage-derived tissue cells (e.g., microglial cells in the brain and Kupffer cells in the liver). The correction of enzyme deficiency is mediated by donor leukocytes that engraft in the host tissue and secrete the missing enzyme. The leukocytes may enter the tissue directly, or secreted enzyme may enter the circulation. CNS therapy relies on donor cells crossing the blood-brain barrier. Enzymatic cross-correction is based on the principle that healthy cells secrete enzyme and can correct the biochemical consequences of enzymatic deficiency in neighboring cells.
Clinically, the MPSs are characterized by CNS compromise and potential involvement of the skeletal system, heart and heart valves, connective tissue, facial structure, cornea, retina, upper airway, and liver. Adverse effects on growth and development are common. The pattern of organ damage is specific to each disorder, as is the response to transplant; and transplant is not effective for all diseases in this class.
Allogeneic transplantation is best established and most successful for MPS I (Hurler syndrome) and is considered investigational for the other diseases. Transplantation does not correct all manifestations of MPS I. It can correct upper airway obstruction, hepatosplenomegaly, and corneal clouding and may improve hydrocephalus, hearing dysfunction, and abnormalities of growth and development. It is least effective for skeletal disease. The majority of published reports of transplantation for MPS I use non–radiation-containing myeloablative regimens. There is debate about the most appropriate stem cell source to use for transplants in children with MPS I. A recent investigation of the transplant outcomes of 258 children with MPS I found that chimerism and enzyme levels were superior when an HLA 6/6 UCB unit was used compared with all other donor sources combined, including matched sibling donors. However, siblings who were disease carriers were included in the analysis and may contribute to lower enzyme levels in the matched sibling group.
Sphingolipidoses are a group of lipid storage disorders related to sphingolipid metabolism and include Tay-Sachs, Niemann-Pick, Fabry, Gaucher, and Krabbe diseases and metachromatic leukodystrophy (MLD). Enzyme replacement therapy is available for Fabry and Gaucher diseases, and with this therapy patients with these diseases can live into adulthood. Despite having similar pathophysiology to the other sphingolipidoses, enzyme replacement therapy is not available for Krabbe disease or MLD and HSCT is the only potentially curative option for these patients. Both Krabbe disease and MLD have early onset/infantile forms that can be successfully treated if children undergo a transplant when asymptomatic or with few symptoms. Late onset forms of the diseases can be stabilized if symptoms are present, but not in the setting of advanced disease.
X-linked adrenoleukodystrophy (ALD) is a perioxisomal disorder characterized by adrenal gland dysfunction, progressive inflammatory demyelination of the brain, and chronic axonal degeneration of the spinal cord. The gene responsible for the clinical manifestations of ALD is mapped to Xq28 and encodes for the perioxisomal half-transporter ABCD1. The transporter plays a key role in the metabolism of very long chain fatty acids (VLCFA). The dysfunction of ABCD1 prevents the degradation of VLCFA and results in their tissue accumulation.
Cerebral ALD is the most severe phenotype of the disease. Boys with ALD have a 60% lifetime risk for developing the phenotype, which is asymptomatic in the early stages. Progression is characterized by MRI changes, emotional lability, impaired visuospatial function, cognitive deterioration, hemiplegia or quadriparesis, and a vegetative state followed by death within 2 to 5 years after the onset of symptoms. MRI change is evaluated by the Loes severity system, with lower scores having less disease burden.
Therapeutic strategies in cerebral ALD include metabolic correction of the VLCFA accumulation with non-VLCFA (e.g., Lorenzo’s oil and walnut oil), adrenal replacement, antioxidant supplementation aimed at reducing CNS inflammation that accompanies the disease, and allogeneic HSCT. Allogeneic HSCT is currently the only nonexperimental, potentially curative option for boys with progressive cerebral ALD. The precise mechanism of effect is not clear. Proposed mechanisms include correction of the metabolic defect in the CNS, the presence of donor microglial cells in the brain, and decreased inflammatory and immunologic reactions that contribute to disease progression. Similar to MPS disorders, the neurologic deterioration present before transplant cannot be recovered and the disease can progress for months after successful HSCT. Overall survival is best when transplant is performed in boys with few or no neurologic deficits, low Loes score, and use of an RD. Because of this it is essential that transplant occurs as soon as disease progression is detected by MRI screening or symptom development.
Hemophagocytic Lymphohistiocytosis
Hemophagocytic lymphohistiocytosis (HLH) is a potentially life-threatening condition of severe inflammation caused by uncontrolled proliferation of lymphocytes and macrophages. HLH can be classified as primary or secondary based on the presence of a genetic mutation in primary disease. Secondary disease develops in response to an infectious, rheumatologic, malignant, or other stimulus. HLH typically presents as fever, hepatosplenomegaly, and cytopenias. Other features include hypofibrinogenemia, hypertriglyceridemia, hepatic dysfunction, hyperferritinemia, neurologic symptoms, low NK cell activity, and elevated soluble IL-2 receptor levels. Allogeneic HSCT is the only curative option for children with primary HLH. The estimated overall 3-year probability of survival after HSCT for patients treated on protocol HLH-94 was 64%; 71% ± 18% with matched RDs, 70% ± 16% with matched UDs, 50% ± 24% with family haploidentical donors, and 54% ± 27% with mismatched UDs. Transplant conditioning has historically been with BU-based myeloablative regimens. Unfortunately, fully myeloablative regimens are associated with transplant-related mortality rates of approximately 25%. Reduced-intensity regimens are being used by some centers in an attempt to decrease acute toxicity and currently are being investigated in the multiinstitutional setting. Reduced-intensity regimens are associated with an increased risk for mixed chimerism and frank graft failure and thus may not be appropriate when UCB is the only potential stem cell source.
Treatment of Bone Marrow Failure—HSCT in Congenital and Acquired Bone Marrow Failure Syndromes
Acquired Aplastic Anemia.
Severe aplastic anemia (SAA) is a syndrome characterized by peripheral pancytopenia and marrow hypoplasia (see Chapter 6 ). Mortality associated with this disorder in children was historically as high as 50% in the first 6 months. Although a number of immunosuppressive and marrow stimulatory therapies have shown reasonable activity, the only curative treatment remains SCT. The potential efficacy of allogeneic SCT for patients with SAA was established with the initial publication in 1972 of results achieved in four patients ( Fig. 8-4 ). Randomized studies have since established the superiority of matched allogeneic SCT over supportive care (androgens in the 1970s, immunosuppressive therapy with antithymocyte globulin in the 1980s and 1990s) in both children and, more recently, adults. In the setting of a matched sibling donor, the role of pre-HSCT conditioning is mainly to provide immunosuppression. CY in combination with antithymocyte globulin is the standard regimen, with cyclosporine and short-course methotrexate for GVHD prophylaxis. This approach cures almost 90% of patients with none of the sequelae associated with radiation-containing regimens. Stem cell source has traditionally been bone marrow, but many centers have switched to peripheral blood progenitor cell grafts for ease of collection and faster engraftment kinetics. In a retrospective study of European and American registries, patients younger than 20 years of age had increased risk for cGVHD and higher overall mortality with peripheral blood cell versus bone marrow grafts. Immunosuppressive therapy before matched sibling HSCT leads to an inferior outcome, mostly owing to increased rates of graft rejection.
