Primary and Metastatic Brain Tumors
Muhammad O. Chohan
Gregory N. Gan
Olivier Rixe
I. PRIMARY BRAIN TUMORS
A. Epidemiology, risk factors, and survival
Primary brain tumors (PBT) constitute a wide spectrum of neoplasms that have cellular origin in the central nervous system (CNS). In the United States, a nationwide collection of nonmalignant brain tumors began in 2004 following the passage of the “Benign Brain Tumor Cancer Registries Amendment Act.” These data, along with statistics on malignant PBT collected by various state and nationwide cancer registries, is tracked by the Central Brain Tumor Registry of the United States (CBTRUS). The most recent report covered a 4-year trend (2004 to 2007) in nonmalignant PBT and a 27-year trend (1980 to 2007) in malignant PBT across the nation. On the basis of age-adjusted incidence rates between 2007 and 2011, it is estimated that 68,470 new cases of primary malignant and nonmalignant brain and CNS tumors will be diagnosed in the United States in 2015. Of these, about 23,180 will be primary malignant and 45,300 nonmalignant.1
Nonmalignant tumors were about twice as common as malignant tumors among adults (aged ≥20 years). Women had an overall brain tumor incidence rate of 23.26 per 100,000 persons; men had a corresponding rate of 19.42. Incidence rates among children aged 0 to 19 years were much lower than among adults (5.42 vs. 21.42 per 100,000 adults), but the tumors were much more likely to be malignant in children: 65.2% versus 33.7% malignant in adults. It is estimated that 13,770 deaths will be attributable to PBT and CNS tumors in the year 2015.1
No underlying etiologies have been clearly identified for primary glial tumors except exposure to ionizing radiation, although findings of studies on electromagnetic fields, head trauma or other environmental factors such as pesticides are still being debated.
Optimal strategic recommendations are being made in the context of a multidisciplinary clinic and tumor board that includes neurosurgeons, radiation and medical oncologists, neuropathologists, neuroradiologists, and supportive care staff and physicians.
B. Malignant gliomas
Malignant gliomas represent a heterogeneous category of invasive tumors with a wide range of clinical outcomes. These tumors are
classified on the basis of standard morphologic evaluation by light microscopy techniques, but the latest technology using Next Generation Sequencing is being evaluated for better prognostication and therapeutic stratification.2,3
classified on the basis of standard morphologic evaluation by light microscopy techniques, but the latest technology using Next Generation Sequencing is being evaluated for better prognostication and therapeutic stratification.2,3
The World Health Organization (WHO) grading scheme classifies PBT into benign (WHO grade I), low-grade (WHO grade II), anaplastic (WHO grade III), and malignant (WHO grade IV) gliomas. Malignant gliomas (WHO grade IV, glioblastoma multiforme, GBM) can arise either de novo or as a result of progression from low-grade gliomas (LGG, i.e., secondary GBM). This classification is based on the presence or absence of nuclear atypia, mitoses, endothelial proliferation, and necrosis. In addition to the grade, astrocytic, oligodendroglial or mixed (oligoastrocytic) tumors are reported by the neuropathologist. A large range of genetic alterations have been extensively reported in gliomas and include (but are not limited to) EGFR amplification, deletion or mutations, PTEN mutations, Olig2 expression, IDH 1/2 mutations, LOH 10q and/or 19q, PI3kinase alterations, RB mutations, and VEGFR overexpression. A new molecular classification has recently been proposed for GBM, and it identifies 4 molecular subtypes (proneural, neural, classic, and mesenchymal) whose clinical relevance is determined by six molecular markers (TP53, IDH1, PDFGRA, EGFR, NF1, and CDKN2A).4
1. Grade I glioma
Pilocytic astrocytomas are WHO grade I tumors that most commonly arise in the posterior fossa. These tumors are most common in the pediatric population and have a very low rate of recurrence when a gross total resection (GTR) is achieved.5 Even in the setting of subtotal resection (STR), itis often recommended that re-resection be considered, if possible, versus observation. Often radiation is deferred if the patient is young, but can be considered in select situations such as unresectable disease (i.e., thalamic, hypothalamic).
2. Grade II glioma
WHO grade II gliomas, or LGG, are most commonly observed in the third and fourth decades of life. This tumor typically appears as a nonenhancing, diffuse, hypointense mass on T1-weighted magnetic resonance imaging (MRI) and a diffuse, hyperintense mass on T2-weighted FLAIR sequence. A biopsy is necessary in these cases to identify the histologic subtype and rule out foci of high-grade malignancy because up to 30% of nonenhancing tumors are shown to be anaplastic (WHO grade III) at the time of surgery. The clinical course of these tumors is tied to the histologic subtype of tumor cells and associated genetic abnormalities. The 5-year overall survival (OS) ranges from 58% to 72%, and the progression-free survival (5-PFS) at 37% to 55%, with oligodendrogliomas having the best 5-OS, at 70%, followed by mixed gliomas, at 56%, and astrocytomas, at 37%.
Conducting two prospective radiation-based clinical trials, EORTC 22844 (construction set) and EORTC 22845 (validation set), Pignatti et al.6 demonstrated that unfavorable, “high-risk” prognostic factors included age exceeding 40 years, predominant astrocytoma histology, largest tumor size exceeding 6 cm, tumors crossing midline, and the presence of a preexisting neurologic deficit. Several other studies have similarly characterized relevant prognostic factors for LGGs.
Conducting two prospective radiation-based clinical trials, EORTC 22844 (construction set) and EORTC 22845 (validation set), Pignatti et al.6 demonstrated that unfavorable, “high-risk” prognostic factors included age exceeding 40 years, predominant astrocytoma histology, largest tumor size exceeding 6 cm, tumors crossing midline, and the presence of a preexisting neurologic deficit. Several other studies have similarly characterized relevant prognostic factors for LGGs.
The first course of treatment is to offer a maximal safe surgical resection of tumor. Overall, surgery provides a change in mean OS of 5.1 years with STR to 7.5 years with GTR. Despite inconsistencies in defining the extent of resection (volumetric vs. nonvolumetric methods), it is becoming clear that delaying surgery places the patient at an increased risk of malignant degeneration (decreasing malignant progression-free survival, MPFS), neurologic deficits becoming permanent, emergence of a more resistant seizure disorder, and the eventual need for surgical resection in an older patient. In a recent meta-analysis of the role of extent of resection (EOR) in LGG involving 11 large studies with at least 75 patients per study, multivariate analysis showed >98% 5-year OS in patients with 100% EOR based on volumetric analysis. Seven out of 8 studies with nonvolumetric assessment of EOR also confirmed a similar advantage of GTR on 5-OS. The 10-year OS in this series was 76% after GTR and 49% after STR, while MPFS was 12.5 and 7 years, respectively. Another series of 216 patients with a mean follow-up of 4.4 years showed that predicted OS was negatively influenced by a minimal residual tumor volume (as small as 10 cm3). The obvious caveat is that OS is not a valid tool in assessing outcomes in LGG because survival is often long. Therefore, achieving maximal survival with good quality of life (QoL) depends on the balance between decreasing oncologic burden (EOR) and respecting functional surgical boundaries.
Data from three prospective randomized clinical trials in adults with LGG provide guidelines for the timing of radiation as well as the appropriate postoperative dose to use. The EORTC 22845 study demonstrated that the use of immediate versus delayed postoperative radiotherapy in patients improved median PFS (5.3 vs. 3.4 years), but did not affect median OS (7.4 vs. 7.2 years). Furthermore, there was no difference in the rate of malignant transformation between groups. However, this study observed that the use of immediate radiotherapy improved 1-year seizure control rates. Therefore, radiotherapy should be used for symptomatic patients as it can improve patient overall QoL.7,8 EORTC 22844 and INT/NCCTG both examined the use of high-versus lower-dose radiation regimens, and found that radiation doses of 45 to 54 Gy resulted in similar outcomes compared with
higher doses (59 to 65 Gy) and were associated with improved tolerance without a decrement in OS when using the lower dose.9,10 Acceptable postoperative radiation doses for LGG range between 50.4 and 54 Gy, although a retrospective analysis by Shaw et al.11 suggested that postoperative radiation doses >53 compared with those <53 Gy were associated with a superior 5- and 10-year OS (67% and 40% vs. 50% and 20%, respectively).
higher doses (59 to 65 Gy) and were associated with improved tolerance without a decrement in OS when using the lower dose.9,10 Acceptable postoperative radiation doses for LGG range between 50.4 and 54 Gy, although a retrospective analysis by Shaw et al.11 suggested that postoperative radiation doses >53 compared with those <53 Gy were associated with a superior 5- and 10-year OS (67% and 40% vs. 50% and 20%, respectively).
On the basis of these data, postoperative radiation therapy is often recommended for patients after a STR or biopsy, particularly if there are additional “high-risk” features. There is, however, no definitive effect of radiotherapy on OS. Chemotherapy (temodar or PCV) is similarly reserved for patients with poor prognostic factors. A recent report of RTOG 0424, a phase II trial examining the use of postoperative radiation (54 Gy) combined with concurrent and adjuvant temozolamide in patients with ≥3 high-risk features showed a 3-year OS of 73.1%.12 The study was not randomized, but when compared with historical control OS, there was a significant improvement in OS, and so further study is warranted. There is at least one report on the use of neoadjuvant temozolomide in diffuse LGG to improve EOR.13
WHO grade II oligodendrogliomas and anaplastic oligodendrogliomas (grade III) deserve special therapeutic recommendations as allelic loss of the short arm of chromosome 1p and the long arm of chromosome 19q occurs in 50% to 70% in both and predicts better response to chemotherapy and longer survival. PCV has been shown to prolong disease-free survival (DFS) but not OS in two randomized phase III trials in patients with anaplastic oligodendrogliomas. However, in a subset of high-risk LGG patients from RTOG 9802, the use of postoperative radiotherapy with PCV over radiotherapy alone demonstrated a significant improvement in median OS (13.3 vs. 7.8 years). Similar results have been shown with RTOG 9402 and EORTC 26951. The emergence of temozolomide, which is far more tolerable than PCV, with demonstrated benefit in patients with WHO grade IV glioblastoma multiforme in addition to the concern for delayed radiotherapy-induced neurocognitive toxicity in patients predicted to have potentially longer survival, and the discovery of molecular-chromosomal markers makes the findings of these phase III studies difficult to apply. For example, temozolomide has shown a 31% objective response rate as initial therapy for low-grade oligodendrogliomas in patients with clinical and/or radiographic progression and no prior therapy other than surgery.14 However, the question of whether temozolamide alone can be used to manage LGG patients and whether it can truly replace PCV chemotherapy when combined with radiation is highly controversial and will need to be addressed in future prospective clinical studies.
At the time of recurrence, surgery is still considered the first line of treatment aiming to offer the maximum EOR and also to update histopathologic grading so that postoperative therapy (based on prior medical treatment(s)) can be added when there is evidence of malignant transformation. If surgical resection to GTR is not an option because of extensive areas of brain involvement, upfront radiation can be used in patients who have not received prior radiotherapy. In the setting of prior radiotherapy, salvage chemotherapy is routinely used. Another option, in recurrent disease, is the use of stereotactic radiosurgery or hypofractionated radiotherapy, which, retrospectively, has been shown to be effective (median OS and PFS from reirradiation was 23 and 12 months, respectively) and tolerable.15
3. Grades III and IV glioma (high-grade gliomas)
High-grade gliomas (HGG) represent over 70% of all newly diagnosed PBT every year, making them the most common type. Anaplastic astrocytoma (AA, WHO grade III) occurs most commonly in the fourth and fifth decades, whereas glioblastoma (GBM, WHO grade IV) occurs most commonly in the fifth and sixth decades. Median OS is 24 to 36 months for AA and 12 to 16 months for GBM. These two types of tumors may appear quite similar on MRI because both often appear as diffuse hypointense lesions on T1-weighted images and both readily enhance after administration of intravenous contrast. These tumors are most commonly observed in the cerebral hemispheres and can have cystic, necrotic, or hemorrhagic components. While the etiology of most HGG is unclear, ionizing radiation, tuberous sclerosis, and NF1/NF2 mutations are known risk factors. Without treatment, median survival is <3 months, whereas with best treatment, median survival is 12 to 15 months for GBM, with only <25% of patients surviving up to 2 years. In the light of this poor outcome, patients must be encouraged to participate in prospective therapeutic clinical trials.16
a. Role of surgical resection in newly diagnosed malignant gliomas
The Glioma Outcomes Project studied patterns of care for adults with newly diagnosed malignant gliomas (grade III and IV). A total of 788 patients were followed across 52 sites and 154 physicians in the United States. Perioperative corticosteroid and antiepileptic use was common for both types of lesions, while grade IV GBMs were more likely to have a GTR and adjuvant RT compared with AA. Data from the Glioma Outcomes Project similarly provided class II evidence to support tumor grade, patients’ age, and performance status as prognostic factors for survival in patients with newly diagnosed malignant gliomas. It also showed that surgical resection was better than biopsy alone.
Like LGG, surgical resection is the mainstay of treatment in HGG.17 Although there is no level I study comparing the effect of EOR on clinical outcomes in HGG, several prospective and retrospective studies have shown that (a) surgical resection is better than biopsy alone, (b) GTR is better than near total resection (NTR), and (c) NTR is better than STR. A meta-analysis of 14 large HGG studies (>200 patients each) showed survival benefit with greater EOR for both grade III and IV malignant gliomas on multivariate analysis. Chaichana et al.18 showed a greater than 70% volumetric resection and <5 cm3 of residual tumor volume as independent surgical thresholds affecting survival and recurrence in newly diagnosed GBM.
Multifocal and multicentric GBMs account for 0.5% to 20% of all GBMs and carry a less favorable prognosis. Multiple craniotomies in a single session to achieve GTR for these lesions, in highly selected cases, have similarly shown to be feasible and provide survival advantage.
Several recent technological advances, such as use and availability of intraoperative MRI and fluorescence-guided surgery, along with pre- and intraoperative mapping (functional MRI, awake procedures) have significantly improved EOR in modern surgical series.
b. Postoperative management and prognostic factors for newly diagnosed WHO grade III gliomas
Despite advances in surgical resection, treatment of malignant gliomas has resulted only in a modest increase in survival. Postoperative management of AA remains controversial in 2015 because of a lack of randomized prospective data. However, postoperative radiotherapy (59.4 to 60 Gy) with concurrent temozolomide is the most common treatment approach. The potential benefit of combined modality therapy and adjuvant therapy is currently being evaluated in a prospective trial (CATNON) through NRG Oncology.19
Positive prognostic factors include high Karnofsky Performance Score (KPS), GTR, and younger age (<60). In addition, multiple studies have now shown that methylation of the O6-methylguanine-DNA methyltransferase (MGMT) promoter is prognostic of improved survival, and may be predictive of increased responsiveness to temozolomide chemotherapy in GBM.
c. Postoperative therapies in newly diagnosed WHO grade IV gliomas
1) Chemotherapy and radiation
Postoperative radiation treatment has played an important role following GTR, NTR, or biopsy. Clinical studies have helped to refine the method, technology, and the dose for how radiation is now delivered for malignant gliomas. Early
studies demonstrated a benefit with whole brain radiotherapy (WBRT) followed by a boost to the operative site compared with observation or BCNU therapy alone. Modern radiation has moved away from WBRT to the use of MRI imaging to help with delivering directed radiotherapy, and this has improved QoL. In 2005, a study conducted by the European Organisation for Research and Treatment of Cancer (EORTC) and the National Cancer Institute of Canada (NCIC) demonstrated that the addition of temozolamide could improve OS and PFS.20 In this large randomized phase III study, postoperative radiation (60 Gy in 30 fractions over 6 weeks) has been compared with the combination of radiation plus the oral alkylating agent temozolomide (75 mg/m2/day during radiation followed by dosing for 5 days in a 28-day cycle [for 6 cycles] given at 150 to 200 mg/m2/day). The combination arm showed an acceptable toxicity profile and provided a survival advantage (14.6 vs. 12.1 months, p < 0.001).20 This combination, also known as the “Stupp regimen,” is now considered the standard of care therapy and is used as the control arm in current prospective clinical trials.
studies demonstrated a benefit with whole brain radiotherapy (WBRT) followed by a boost to the operative site compared with observation or BCNU therapy alone. Modern radiation has moved away from WBRT to the use of MRI imaging to help with delivering directed radiotherapy, and this has improved QoL. In 2005, a study conducted by the European Organisation for Research and Treatment of Cancer (EORTC) and the National Cancer Institute of Canada (NCIC) demonstrated that the addition of temozolamide could improve OS and PFS.20 In this large randomized phase III study, postoperative radiation (60 Gy in 30 fractions over 6 weeks) has been compared with the combination of radiation plus the oral alkylating agent temozolomide (75 mg/m2/day during radiation followed by dosing for 5 days in a 28-day cycle [for 6 cycles] given at 150 to 200 mg/m2/day). The combination arm showed an acceptable toxicity profile and provided a survival advantage (14.6 vs. 12.1 months, p < 0.001).20 This combination, also known as the “Stupp regimen,” is now considered the standard of care therapy and is used as the control arm in current prospective clinical trials.
Attempts at modifying the classic Stupp regimen with additional biologics and hypofractionated radiotherapy (higher dose per fraction, fewer fractions) to date have been unsuccessful in improving survival. Two recent prospective randomized phase III trials conducted in the US and in Europe have failed to demonstrate a survival advantage when bevacizumab (Avastin), a monoclonal antibody against vascular endothelial growth factor (VEGF), is combined with radiation and temozolomide in the adjuvant setting.21 An identical result has been observed with cediranib, a VEGFR tyrosine kinase inhibitor, in a prospective trial.22 Therefore, until a patient subgroup has been defined, these results do not support the use of antiangiogenics for newly diagnosed glioblastoma. A phase II trial at the University of Colorado combining postoperative hypofractionated radiotherapy (60 Gy delivered over 10 fractions) with concurrent temozolamide and avastin followed by adjuvant temozolamide and avastin demonstrated a median PFS and OS of 12.8 and 16.3 months, respectively.23 In comparison, a similar phase II trial from Memorial Sloan Kettering Cancer Center (MSKCC) utilized a similar chemotherapy regimen, but a lower hypofractionated dose of radiotherapy (36 Gy delivered over 6 fractions) showed a median PFS and OS of 10 and 19 months, respectively, which was favorable to historic controls.24 Both these aggressive radiotherapy regimens demonstrated safety in addition to improved convenience for patients over
traditional radiotherapy regimens, and further study of these regimens is warranted. Adjuvant chemotherapies for malignant gliomas are outlined in Table 16.1.
traditional radiotherapy regimens, and further study of these regimens is warranted. Adjuvant chemotherapies for malignant gliomas are outlined in Table 16.1.
d. Electrical field therapy
A recent phase III randomized trial has successfully tested the impact of NovoTTF-100A in the adjuvant setting.25 Tumor treating fields (“TTFields”) stop the growth of tumor cells, resulting in cell death of the rapidly dividing cancer cells in vitro and in vivo. The NovoTTF-100A System is a portable battery or power-supply operated device that produces changing electrical fields, and is applied to the head of the patient by electrically insulated surface electrodes. The frequency of the TTFields used for a particular treatment is specific to the size of the cell type being treated. The percentage of patients alive at two years when treated with TTFields together with temozolomide was 43% compared with 29% when treated with temozolomide alone. Patients treated with TTFields together with temozolomide demonstrated a significant increase in OS compared with temozolomide alone (median OS from randomization of 19.4 months compared with 16.6 months, hazard ratio = 0.75, p = 0.022). QoL surveys demonstrated an improvement with the NovoTTF-100A System in recurrent GBM subjects compared with best standard of care options alone.
e. Recurrent GBM
GBM recurrence can occur at 34 to 36 weeks after primary resection. There is mounting evidence in support of multiple reoperations to improve survival in carefully selected patients, and that a PFS of >3 months significantly predicts survival after repeat surgery. Recurrent glioblastoma is treated by surgical debulking for selected cases with or without carmustine
(BCNU) wafers, bevacizumab, stereotactic radiosurgery (SRS), stereotactic radiotherapy (SRT), nitrosoureas (lomustine), or other single-agent therapies such as carboplatin (see Table 16.2).
(BCNU) wafers, bevacizumab, stereotactic radiosurgery (SRS), stereotactic radiotherapy (SRT), nitrosoureas (lomustine), or other single-agent therapies such as carboplatin (see Table 16.2).
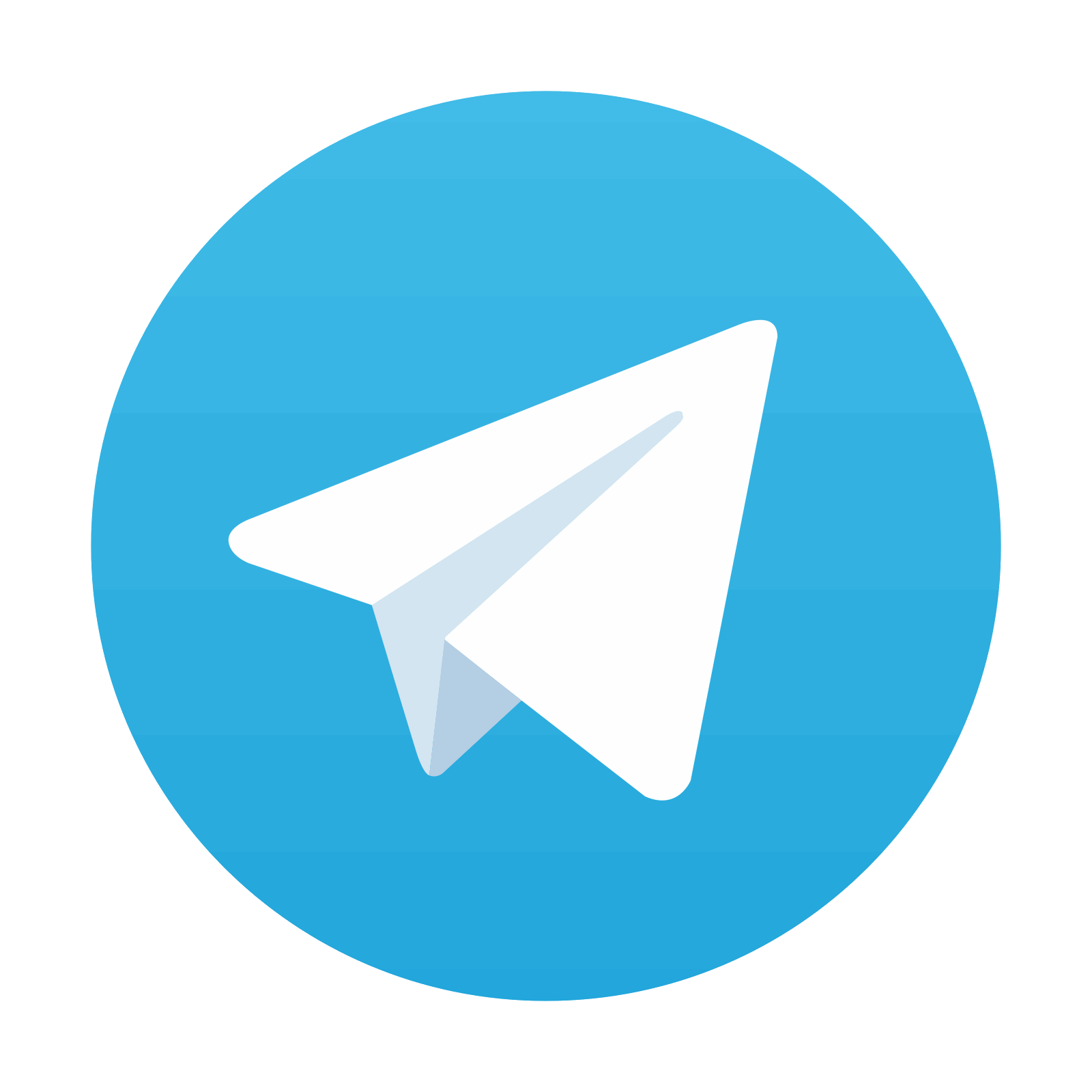
Stay updated, free articles. Join our Telegram channel

Full access? Get Clinical Tree
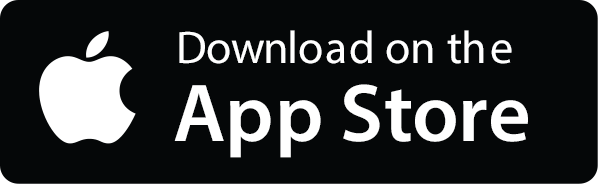
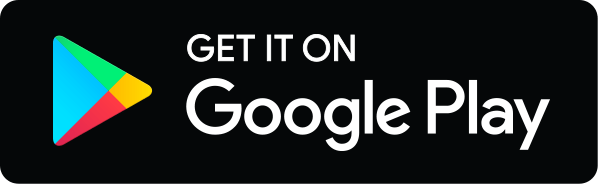