Part 1 / Diabetic Neuropathy

Why would patients who for so long were using only one to two injections of insulin per day who had no access to intensive glucose monitoring, blood pressure (BP) or lipid management have exceeded all expectations by minimizing their prevalence of complications? Other patients with T1DM develop proliferative retinopathy and chronic kidney disease despite remaining vigilant in maintaining their prescribed A1C levels. Clearly, factors other than simple glycemic control factor into long-term outcomes for all patients with diabetes.
more AGEs become receptor bound, the greater the likelihood of developing a complication over time. However, patients, such as the Joslin Gold Medalists, appear to have been provided with a truly unique gift from their parents known as a “soluble” AGE. As the AGE binds to the RAGE, a small protein ligand breaks free of the receptor effectively “blocking” the complication pathway from progressing forward. Patients who can produce the “soluble RAGE” are likely to be symptom free, whereas those patients who lack the soluble component are prone to develop the complications.
declined from 21.7% in 1994 to 20% in 2007. All other risk factor trends have been increasing during this same reporting period.15 Duration of diabetes and exposure to glycemic burden also impact the risk of complications.16

circulation in response to tissue ischemia through the release of growth factors and cytokines. The EPCs hone into the ischemic or damaged tissue and stimulate endothelial repair. In addition to traditional cardiovascular risk factors, oxidative stress has been associated with reduction in the number and function of circulating EPCs, whereas an expanded EPC pool decreases cardiovascular mortality.25
are fortunate to experience no diabetes-related complications, whereas those less fortunate with prediabetes may develop retinopathy or painful diabetic neuropathy (Fig. 5-1).
TABLE 5-1. Practical Approaches to Reducing Oxidative Stress | |||||||
---|---|---|---|---|---|---|---|
|
derangements related to hypertension and hyperlipidemia. DPN is statistically associated with other microvascular complications such as DR and diabetic neuropathy.46
TABLE 5-2. Risk Factors for Diabetic Neuropathy | |||||||||||||||
---|---|---|---|---|---|---|---|---|---|---|---|---|---|---|---|
|
TABLE 5-3. Classification of Diabetic Neuropathy | ||||||||||||||||||||||||||
---|---|---|---|---|---|---|---|---|---|---|---|---|---|---|---|---|---|---|---|---|---|---|---|---|---|---|
|
Focal and multifocal neuropathies are confined to the distribution of a single peripheral nerve (mononeuropathy) or multiple peripheral nerves (mononeuropathy multiplex). Mononeuropathies are caused by vasculitis, ischemia of the capillaries supplying the neurons, or nerve infarcts.47 Cranial neuropathy in diabetic patients is rare, typically affecting older persons with a long history of diabetes.48 Cranial nerves III, IV, or VI may be involved (Fig. 5-12). The classic presentation of a cranial neuropathy is acute-onset diplopia with ptosis and papillary sparing associated with ipsilateral headache. Neurologic deficits resolve on average within 21/2 months. Recurrence rates are 25% in patients with diabetes. Advise patients with a cranial neuropathy to wear a patch over the affected eye and to adhere to strategies that improve glycemic control.
Nerve entrapment syndromes begin gradually and may become disabling over time without intervention. Most often, the median, ulnar, peroneal, lateral femoral cutaneous, or tibial nerve within the tarsal tunnel is involved. Entrapment syndromes affect up to 30% of patients with diabetes and should be evaluated carefully in all those with signs and symptoms of neuropathy.49
Carpal tunnel syndrome (median neuropathy) is a clinically relevant problem in 6% of patients with diabetes.50 Painful paresthesias of the fingers may progress to a deep-seated ache, which radiates proximally through the forearm. Symptoms are worse at night. Motor weakness can become progressive, and thenar wasting occurs over time.
Ulnar neuropathy occurs in 2% of diabetic patients as a result of nerve compression immediately distal to the ulnar groove beneath the edge of the flexor carpi ulnaris aponeurosis in the cubital tunnel. Alcoholism is a risk factor. Typical symptoms include painful paresthesias in the fourth and fifth digits associated with hypothenar and interosseous muscle wasting. Treatment is conservative. Patients with motor loss and muscle wasting may require surgical intervention.
Compression of the lateral femoral cutaneous nerve (meralgia paresthetica), although uncommon in diabetes, can result in pain, paresthesias, and sensory loss over the lateral aspect of the thigh. Most cases resolve spontaneously. In cases associated with severe pain, allodynia, and disability, corticosteroid injections using focal nerve blocks at the inguinal ligament or surgical decompression may be required.
Tarsal tunnel syndrome is a painful lower limb entrapment syndrome that involves the tibial nerve, as it traverses the tarsal tunnel. The tibial nerve innervates only the muscles of the soles. Walking or standing triggers severe burning pain over the plantar aspect of the foot. A positive Tinel sign on the underside of the medial malleolus with atrophy of the sole muscles are typical
clinical observations. Sensation over the dorsum of the foot is normal. Ankle reflexes are maintained. Nerve conduction studies demonstrate asymmetry compared with the normal leg.
Diabetic truncal radiculoneuropathy affects middle-aged and elderly men. The primary feature is pain of acute onset that resolves spontaneously within 4 to 6 months. The pain— which is worse at night—is described as an aching or burning sensation with superimposed lancinating stabs. Patients describe the location of pain as being in a girdle-like distribution along the lower thoracic or abdominal wall. The pain may be unilateral or bilateral. Patients may experience profound weight loss associated with the onset of their symptoms. Clinical findings range from no abnormalities to sensory loss and painful hyperesthesia in a complete dermatomal pattern.
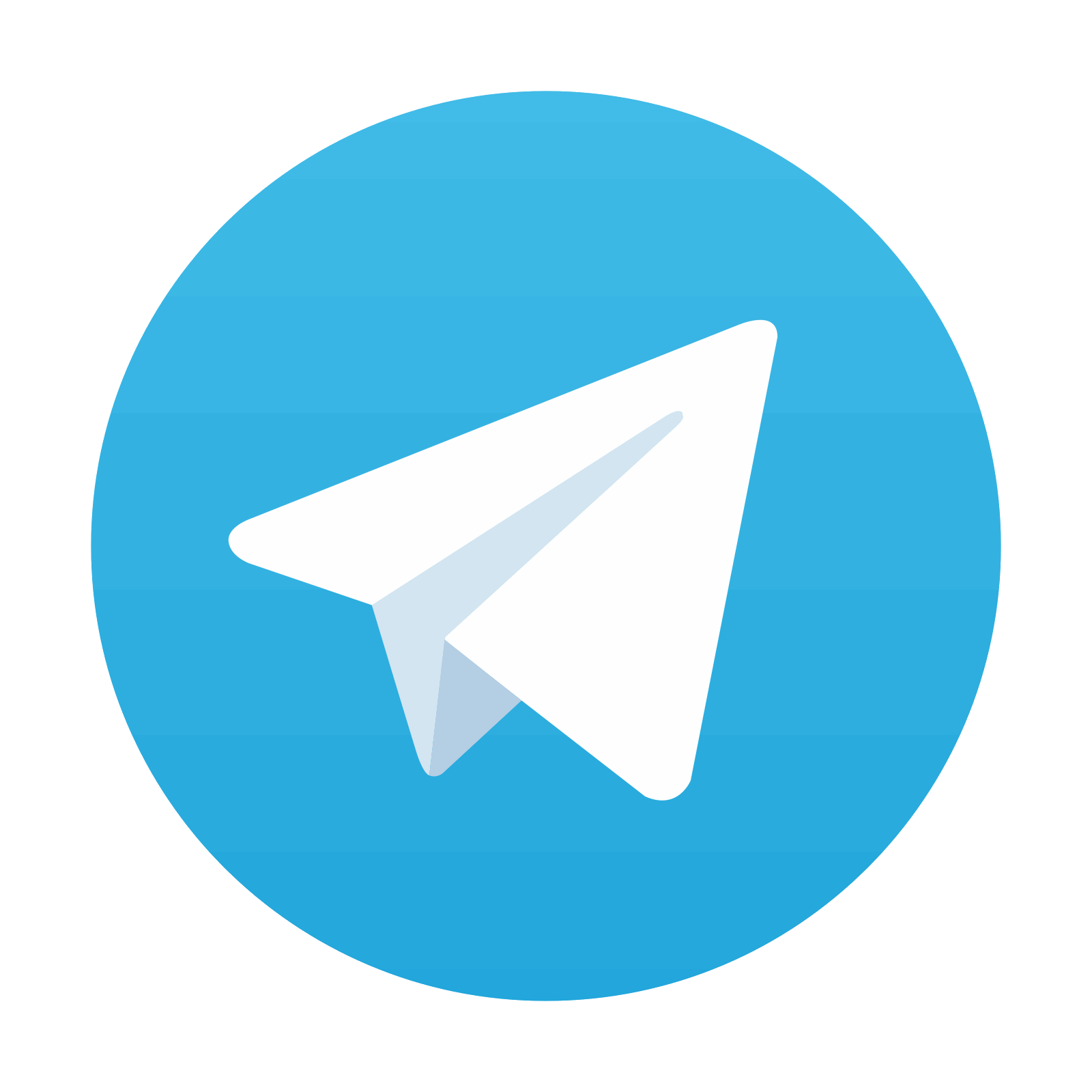
Stay updated, free articles. Join our Telegram channel

Full access? Get Clinical Tree
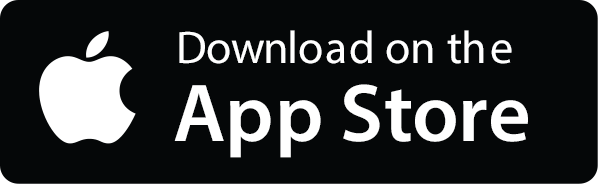
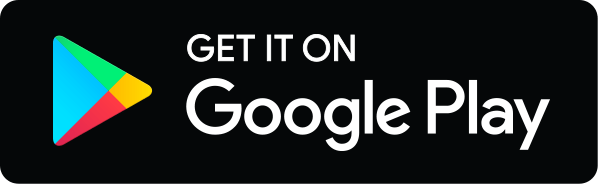
