Gene name
3′UTR
miR sites
References
NR3A1/ESR1
4307 b
Let-7
Manavathi et al. (2013)
miR-137
Zhao et al. (2008)
Zhao et al. (2008)
Zhao et al. (2008)
miR-145
miR-17-5p
miR-18a/19b/20b
Manavathi et al. (2013)
miR-181
Pandey and Picard (2010)
miR-19
Pandey and Picard (2010)
miR-193b
Klinge (2012)
miR-206
miR-221/222
miR-22
miR 26ab/1297
Pandey and Picard (2010)
miR-302
Klinge (2012)
NR3A2/ESR2
871 b
miR-92
Al-Nakhle et al. (2010)
NR3C1/GRα
3985 b
miR-101
Riester et al. (2012)
miR-130b
Tessel et al. (2011)
miR-124/506
miR-142-3p
miR-18a
miR-433
Riester et al. (2012)
Riester et al. (2012)
miR-96
NR3C2/MR
2597 b
miR-124/506
Sõber et al. (2010)
Sõber et al. (2010)
miR-135a
NR3C3/PGR
9492 b
miR-126-3p
Cui et al. (2011)
miR-181
Maillot et al. (2009)
miR-200a
Haraguchi et al. (2014)
miR-26ab/197
Maillot et al. (2009)
NR3C4/AR
6783 b
miR-135b
Östling et al. (2011)
Östling et al. (2011)
Östling et al. (2011)
Östling et al. (2011)
Östling et al. (2011)
Östling et al. (2011)
Östling et al. (2011)
Östling et al. (2011)
miR-185
miR-297
miR-299-3p
miR-34 ac
miR-371
miR-421
miR-449ab
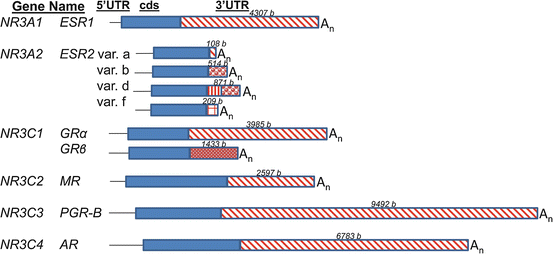
Fig. 5.1
A diagram of the human mRNAs encoding steroid hormone receptors. The mRNAs encoding estrogen receptor α (NR3A1 or ESR1), estrogen receptor β (NR3A2 or ESR2), glucocorticoid receptors α and β (NR3C1 or GR), mineralocorticoid receptor (NR3C2 or MR), progesterone receptor (NR3C3 or PGR) and androgen receptor (NR3C4 or AR) are diagrammed. The 5′ untranslated regions (“5′UTR ”) are shown as narrow black lines, the coding sequences (“cds”) are solid blue boxes, the 3′untranslated regions (“3′UTR”) are red patterned boxes, and the polyA tail is indicated by “An”. For the different 3′UTRs in the variant (“var.”) mRNAs of ESR2 and GR, the different patterns in the 3′UTR boxes indicate unique sequences. The lengths of the 3′UTRs is indicated in bases (“b”) above the 3′UTR box. The information is from the reference mRNA sequences from GenBank: NM_000125.3 (ESR1); NM_001437.2, NM_001040275.1, NM_001214902.1, NM_001271876.1 (ESR2); NM_000176.2, NM_001020825.1 (GR); NM_000901 (MR); NM_000926.4 (PGR); NM_000044.3 (AR)
The 3′UTR sequences of mRNAs are predicted to form stable structures with algorithms such as MFOLD (Zuker 2003). For example, the folded structure of the 4307 base long 3′UTR of ESR1 mRNA (depicted in Fig. 5.2) has numerous single stranded regions that are susceptible to cleavage by common ribonucleases such as RNase A. Cleavage at any position in the 3′UTR separates the 5′UTR and cds from the polyA tail (“An” in Figs. 5.1 and 5.2) and results in rapid degradation of the mRNA (Bevilacqua et al. 2003). In the example of estrogen up-regulation of vitellogenin gene expression in frog liver, estrogen treatment lowers the rate of the cleavage of vitellogenin mRNA within its 3′UTR (Dodson and Shapiro 2002). The 3′UTR sequences of an mRNA are fairly well-conserved across related species, although insertions and deletions of sequence are well tolerated (Mitchell and Ing 2003; Manjithaya and Dighe 2004). The 3′UTR sequences, structures, and binding molecules (proteins and microRNAs ) confer its function: regulating the lifespan of the mRNA.
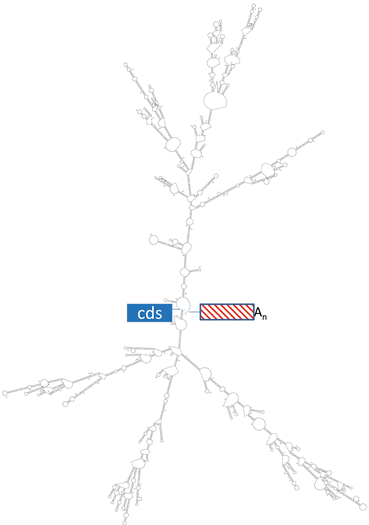
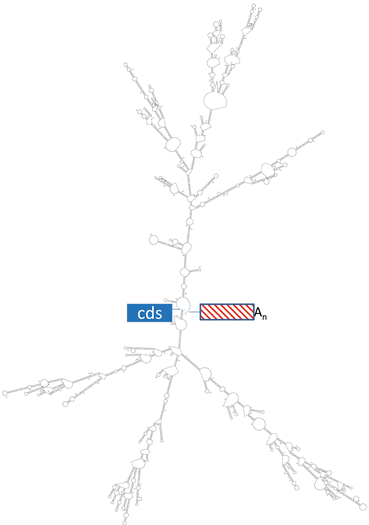
Fig. 5.2
Predicted structure in the 3′UTR of ESR1 mRNA. The MFOLD algorithm predicted the folded structure shown for the 5′ end of the 3′UTR (1330 bases) of the human ESR1 mRNA. The end at left joins the coding sequence (“cds”) while the end at right joins the rest of the 3′UTR and the polyA tail (“An”). The free energy of the predicted structure is −474 kcal/mol
As in the case of mRNA synthesis (transcription) in the nucleus, rates of mRNA degradation in the cytoplasm are regulated by finite sequence elements in the 3′UTR and the proteins that bind them (Guhaniyogi and Brewer 2001). The most well characterized mRNA elements are AU-Rich Element s (AREs). These were first identified as instability elements in the 3′UTRs of oncogene and cytokine mRNAs, e.g. those encoding c-fos (FOS) and tumor necrosis factor (TNF; Zhang et al. 2002; Bevilacqua et al. 2003). There are four distinct classes of AREs (Stellato 2004). The simplest is individual AUUUA elements scattered in 3′UTR sequences, as in follicle-stimulating hormone β-subunit (FSHB) and epidermal growth factor receptor (EGFR) mRNAs (Balmer et al. 2001; Shim and Karin 2002; Manjithaya and Dighe 2004). More complex AREs have arrays of several AUUUA elements in nearby regions of the 3′UTR, such as those in many oncogene mRNAs. Other sequence elements involved in stability have been identified, such as C-rich elements on the 3′UTR of the LHCGR mRNA encoding the luteinizing hormone receptor (Menon et al. 2004; Ing 2005).
There are multiple families of proteins that bind AREs, including AU-binding factors (e.g. HNRPD gene products, also known as AUF1 ), zinc-finger proteins (e.g. tristetraprolin, the ZFP36 gene product) and relatives of the Drosophila RNA-binding ELAV protein (e.g. ELAV1 which is also known as HuR ; Staton et al. 2000; Shim and Karin 2002). While some ARE -binding proteins (such as tristetraprolin) destabilize the ARE-bearing mRNA, others (such as HuR) have stabilizing effects. The HNRPD gene produces four AUF1 proteins AUF1p37, AUF1p40, AUF1p42 and AUF1p45 by alternative splicing of the mRNA. All of the proteins bind to AREs. However, AUF1p37 and AUF1p42 destabilize mRNAs while AUF1p40 and AUF1p45 stabilize the mRNAs they bind (Wagner et al. 1998; Loflin et al. 1999; Tolnay et al. 1999; Chen et al. 2001; Mukherjee et al. 2002; Sarkar et al. 2003; Lal et al. 2006). Most RNA binding proteins are ubiquitously expressed although there is some degree of tissue-specificity in the distribution of some of them (Brewer 2001; Hollams et al. 2002; Zhang et al. 2002; Lu and Schneider 2004). Interestingly, the expression of some genes encoding ARE-binding proteins are regulated by steroid hormones via their receptor proteins (Cuadrado et al. 2003; Arao et al. 2002, 2004; Ing 2010).
While some post-transcriptional regulation of mRNA stability is the result of RNA binding proteins (either stabilizing or destabilizing), other post-transcriptional regulation is performed by non-coding regulatory RNAs such as microRNAs . MicroRNAs are short (about 22 bases) single-stranded, non-coding RNAs that regulate expression of protein encoding genes, usually by destabilizing the mRNA or repressing translation. The microRNAs that effect the changes in gene expression of the greatest magnitude usually do both (Baek et al. 2008). MicroRNAs exist in large complexes with proteins, called RNA-Induced Silencing Complexes or RISC . Within the RISC, microRNAs are very stable even in bodily fluids, such as serum, that contain high levels of RNAses. MicroRNAs act by binding their 5′ seed sequence to a complementary sequence within the 3′UTR of the mRNA, which directs the RISC complex. Subsequently, the mRNA is degraded in the exosome. While there are only approximately a thousand microRNA genes in the human genome, each individual microRNA can regulate the expression of hundreds of protein-encoding genes. It is estimated that microRNAs regulate more than a third of human genes (Ing et al. 2009). Therefore, it is not surprising to note that microRNAs regulate the expression of genes encoding the steroid hormone receptors. For discussion of microRNAs regulating steroid hormone action by their effects on nuclear receptor cofactors and kinase signaling cascades, as well as regulation of the expression of microRNA genes by the steroid hormones via the steroid hormone receptor proteins, see current reviews (Ing 2009; Pandey and Picard 2010; Klinge 2012; Manavathi et al. 2013).
3 Autoregulation of the Stabilities of Messenger RNAs Encoding Steroid Hormone Receptors
Estrogen is a steroid hormone that autoregulates the stability of ESR1 mRNA, which encodes its dominant, active receptor, estrogen receptor α (ERα), in most tissues of the body. Estrogens stabilize ESR1 mRNA in fish liver during the initiation of oogenesis and in mammalian endometrium during the preovulatory surge of estrogen (Flouriot et al. 1996; Mitchell and Ing 2003; Boyce-Derricott et al. 2010). In both cases, the stabilization and resultant up-regulation of ESR1 mRNA are dependent upon ERα protein, because ERα antagonists block the effect. The conservation of this estrogen action across these diverse species implies that it is an important mechanism for augmenting further estrogen responses. In sheep endometrium, a single physiological dose of estradiol up-regulates ESR1 mRNA concentrations fivefold in 24 h, during which time there is no increase in the transcription rate of the ESR1 gene (Ing et al. 1996). The primary mechanism of ESR1 mRNA up-regulation, by increasing ESR1 mRNA stability , was directly demonstrated in vivo with pulse-chase labeling and ex vivo using explants cultured with a transcription inhibitor (Ing and Ott 1999).
The molecular mechanism by which estrogen stabilizes ESR1 mRNA was discovered using the sheep uterus as a model system. Human and sheep ESR1 mRNAs carry 14 and 10 ARE elements in their respective 3′UTRs . (There are no ARE elements in the 5′UTRs or cds regions of human or sheep ESR1 mRNAs.) The AREs are probably responsible for the inherent instability of the ESR1 mRNA and of the heterologous mRNAs that have the ESR1 3′UTR sequence transferred to them (Mitchell and Ing 2003; Kenealy et al. 2000). Using an in vitro stability assay developed with cytoplasmic extracts from endometrial samples from control (ovariectomized) and ovariectomized, estradiol-treated ewes, two discrete (82 bases long) Minimal Estradiol-Modulated Stability Sequences (MEMSSs) were identified in the vast (4366 bases long) 3′UTR of the sheep ESR1 mRNA (Mitchell and Ing 2003). These MEMSSs conferred estradiol-enhanced stability to heterologous mRNAs when they were transferred to them. UV-crosslinking identified several endometrial proteins that were induced to bind the MEMSS by estradiol treatment of ewes. One predominant MEMMS binding protein is AUF1p45, one of four protein products of the HRNPD gene (Ing et al. 2008). The three other estradiol-induced MEMSS binding proteins of 27, 34 and 70 kDa sizes remain to be identified. When recombinant AUF1p45 fusion protein was produced and purified from bacteria, it stabilized ESR1 mRNA in vitro in the presence of endometrial extracts from ovariectomized ewes. Similar analyses with recombinant HuR , a 34 kDa RNA stabilizing protein that binds AREs, did not stabilize ESR1 mRNA in vitro (Ing et al. 2008). Intriguingly, estradiol treatment of ewes increased concentrations of AUF1p45 mRNA and protein apparently by stabilizing the AUF1p45 mRNA (Ing 2010). These data lead to the model of the molecular mechanism of estrogen up-regulation of ESR1 gene expression in Fig. 5.3.
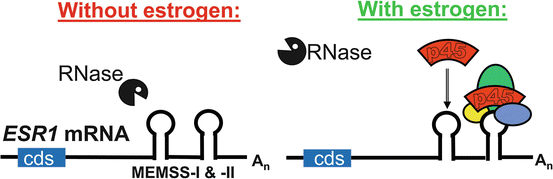
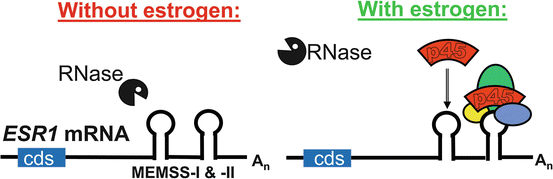
Fig. 5.3
A molecular model for estrogen induction of a stabilizing ribonucleoprotein complex on the 3′UTR of ESR1 mRNA in the sheep uterus. The cartoon of sheep ESR1 mRNA shows the cds as a blue box that is 5′ to the extensive 3′UTR (4366 bases long). Within the 3′UTR are two 82 base long Minimal Estrogen Modulated Stability Sequences (“MEMSSs”), shown as the hairpin structures predicted by MFOLD. Each carries a common 10 base long U-Rich Element (“URE”) on the loop of the hairpin. At left, in the absence of estrogen, the long 3′UTR is susceptible to cleavage by ribonuclease (“RNase”). However, estrogen treatment induces the formation of a stabilizing protein complex that includes AUF1p45 (“p45”) on the MEMSS, which protects the 3′UTR of ESR1 mRNA from cleavage by RNase (right panel)
In contrast, estradiol treatment has also been reported to destabilize ESR1 mRNA in the MCF7 breast cancer cell line (Saceda et al. 1998). The explanation of these contrasting effects of estrogens may become clear when the molecular mechanism(s) that regulate ESR1 mRNA stability are further investigated.
Estrogens also regulate gene expression by binding estrogen receptor β, which is encoded by the distinct ESR2 gene. There are numerous variant ESR2 mRNAs. These result from usage of three promoters on the ESR2 gene, alternative splicing in 5′ and 3′ UTRs and alternative polyA signal usage. Three protein isoforms (estrogen receptor β-1, -2, and -5) are produced. They vary mainly at a small region of the C-terminus. Representative ESR2 mRNAs with 3′ UTR differences are shown in Fig. 5.1 (from human reference sequences in GenBank). In sharp contrast to the ESR1 mRNA, the 3′ UTRs of ESR2 mRNAs are very short. It is interesting to note that splicing within the 3′UTR near the stop codon can trigger nonsense mediated mRNA decay (Ni et al. 2007; McGlincy and Smith 2008; Saltzman et al. 2008). Studies of ESR2 gene expression in normal breast and prostate and cancers derived from those tissues have focused on different 5′ UTR sequences and their effects on translational efficiencies (Smith et al. 2010; Lee et al. 2013). However, the dissimilar 3′UTRs interact with 5′UTR sequences to alter translational efficiencies. Estradiol treatment appears to inhibit translational efficiencies of two of 15 human ESR2 mRNA variants (Smith et al. 2010). However, estradiol treatment of rainbow trout does not alter the stabilities of ESR2 mRNAs in liver (Boyce-Derricott et al. 2010).
The glucocorticoid receptor proteins GRα and GRβ are encoded by the same NR3C1 gene. The mRNAs that encode GRα and GRβ differ by alternative splicing of the last exon, exon 9 (Fig. 5.1). The GRα protein has 50 amino acids encoded by exon 9a prior to the stop codon, while GRβ has only 15 amino acids encoded in exon 9b (Schaaf and Cidlowski 2002). The shorter C-terminus of GRβ does not allow glucocorticoid binding, but GRβ regulates transcription of distinct genes from GRα. GRβ also dimerizes with GRα to act as a dominant negative factor (Vandevyver et al. 2014). The transcripts that encode GRα and GRβ have unique 3′UTRs that carry 10 and 4 type I AREs (AUUUA sequence elements), respectively (Schaaf and Cidlowski 2002; Stellato 2004). Mutation of all ten AUUUA elements to GUUUA in the mRNA encoding GRα stabilizes the mRNA and increases GRα protein levels in transfected COS-1 cells (Schaaf and Cidlowski 2002). Similar effects occur with mutation of just one AUUUA of the four in the 3′UTR of the mRNA encoding GRβ. It is fascinating to note that the mutation occurs naturally in humans, is associated with rheumatoid arthritis, and likely contributes to glucocorticoid resistance (Derijk et al. 2001). While glucocorticoids transcriptionally repress the NR3C1 gene, they also rapidly destabilize NR3C1 mRNA in many cell types (Schaaf and Cidlowski 2003; Ramamoorthy and Cidlowski 2013). Ligand activated GRs are known to act via AREs and kinase pathways to down-regulate a number of genes involved in inflammation (Stellato 2004). There is at least one report that implies that dexamethasone treatment of rats decreases GR mRNA in muscle concurrent with increasing AUF1 mRNA (Sato et al. 2011). However, in that study, GRα and GRβ and the four AUF1 transcripts (two encode proteins that destabilize mRNAs while the other two encode mRNA stabilizing proteins) were not distinguished from each other.
The NR3C2 gene encodes the mineralocorticoid receptor (MR). Although the MR mRNA has a long 3′UTR (2597 bases long, Fig. 5.1) that carries eight AREs (AUUUA elements determined using the AREsite 1.0 algorithm; Gruber et al. 2011), very little has been published about its regulated stability. There is an indication that restraint stress of rats down-regulates MR heteronuclear RNA in nuclei of the hippocampus within 2 h (Herman and Watson 1995). In that study, decreased levels of MR mRNA in the cytoplasm were not detected. However, the technique used was in situ hybridization, which is considered to be semiquantitative. In addition, the in situ hybridization probes could bind partially degraded MR mRNA as well as the full length mRNA, so perhaps degradation was not complete within the 2 h time frame.
The NR3C3 gene encodes progesterone receptors A and B (PGR-A and PGR-B). Their mRNAs are similar to ESR1 mRNA in that they carry a very long 3′UTR (9492 bases, Fig. 5.1). The 3′UTR of PGR mRNA bears numerous AREs: 28 AUUUA elements, with all but three conserved across mammalian species (AREsite 1.0 algorithm). The mRNAs that encode PGR-A and PGR-B differ in 5′ sequence because the transcription of PGR–B mRNA initiates at a site upstream of the PGR–A mRNA transcription initiation site. The PGR-B protein is longer and has a more efficient transcriptional activation domain than PGR-A. In contrast to the bidirectional autoregulation of ESR1 mRNA by estrogen, progestins have only been reported to stabilize PGR mRNA (Tseng and Zhu 1997). The progestin medroxyprogesterone acetate increased the PGR mRNA half-life from 6 to 12 h in primary cultures of stromal cells from human endometrium (Saceda et al. 1998).
The NR3C4 gene has a single transcript that encodes androgen receptor (AR). Androgens autoregulate the stability of AR mRNA in both positive and negative directions (Yeap et al. 1999, 2004). The direction (up or down) of regulation depends upon dose of androgen and the tissue or cell line examined. For instance, androgens stabilize AR mRNA in a prostate cancer cell line but destabilize it in the MDA-453 breast cancer cell line. The extensive 3′UTR of AR mRNA (6783 bases long, Fig. 5.1) does not carry many AREs (only five AUUUAs, AREsite1.0 algorithm). However, the 3′UTR of AR mRNA bears a UC-rich element that is conserved across species. This AR mRNA element binds a poly(C)-binding protein as well as HuR (Yeap et al. 1999, 2004). These two proteins cooperate in a cell-type-specific manner to determine whether androgen stimulation stabilizes or destabilizes the AR mRNA (Yeap et al. 2004). In prostate cancer cell lines, the ErbB3 binding protein 1 (EBP1) destabilized AR mRNA by binding the same UC-rich element in the 3′UTR (Zhou et al. 2010).
4 microRNA Regulation of Messenger RNAs Encoding Steroid Hormone Receptors
The best studied gene encoding a steroid hormone receptor that is regulated by microRNAs is the ESR1 gene (Ing 2009; Pandey and Picard 2010). One reason for this is that ERα is critical to PGR gene expression: so microRNAs that down-regulate the expression of the ESR1 gene will also down-regulate the PGR gene. Other reasons are that ESR1 gene expression is critical to many aspects of mammalian health and may be dysregulated in disease (Klinge 2012). And finally, the 3′UTR of ESR1 mRNA is long and is replete with 65 putative binding sites for microRNAs predicted by algorithms such as TargetScan (Pandey and Picard 2010). There are 13 different microRNA binding sites on the 3′UTR of ESR1 mRNA that have been validated in various model systems (Table 5.1; Pandey and Picard 2010; Manavathi et al. 2013; Klinge 2012; and references therein).
Several studies demonstrated that microRNAs regulate expression of the ESR1 gene in breast cancer. One study began with interest in microRNAs that had increased concentrations in ERα-negative breast tumors compared to ERα-positive breast tumors (Iorio et al. 2005). Among those microRNAs, the investigators focused on the few microRNAs that also had predicted binding sites within the 3′UTR of ESR1 mRNA (Adams et al. 2007). This lead to the discovery that experimentally decreasing the concentrations of miR-206 increased ESR1 gene expression in MCF7 cells. The mechanism by which miR-206 decreased ESR1 gene expression was by destabilization of ESR1 mRNA. Further studies demonstrated that estradiol treatment of breast cancer cells decreased concentrations of miR-206, leading to increased ESR1 mRNA stability and ERα protein concentrations. This describes a second mechanism by which estrogens post-transcriptionally up-regulate ESR1 gene expression, in addition to that described in the sheep uterus (Fig. 5.3). Another group searching for microRNAs that regulate ESR1 gene expression identified 12 microRNAs that were more highly expressed in ERα-negative breast cancer cell lines compared to ERα-positive lines (Zhao et al. 2008). These investigators predicted that miR-221/222 would target the 3′UTR of ESR1 mRNA. By altering levels of the microRNA in the MCF7 and T47D breast cancer cell lines, they demonstrated that miR-221/222 decreased ERα protein concentrations but not ESR1 mRNA concentrations, indicating that this microRNA inhibits translation of ESR1 mRNA. In breast cancer patients who have received chemotherapy, high levels of miR-221 in plasma is predictive of chemoresistance (Zhao et al. 2008). Some have suggested that down-regulation of miR-221 with antagomiR oligonucleotide drugs may be a useful approach to treating breast cancer (Piva et al. 2013).
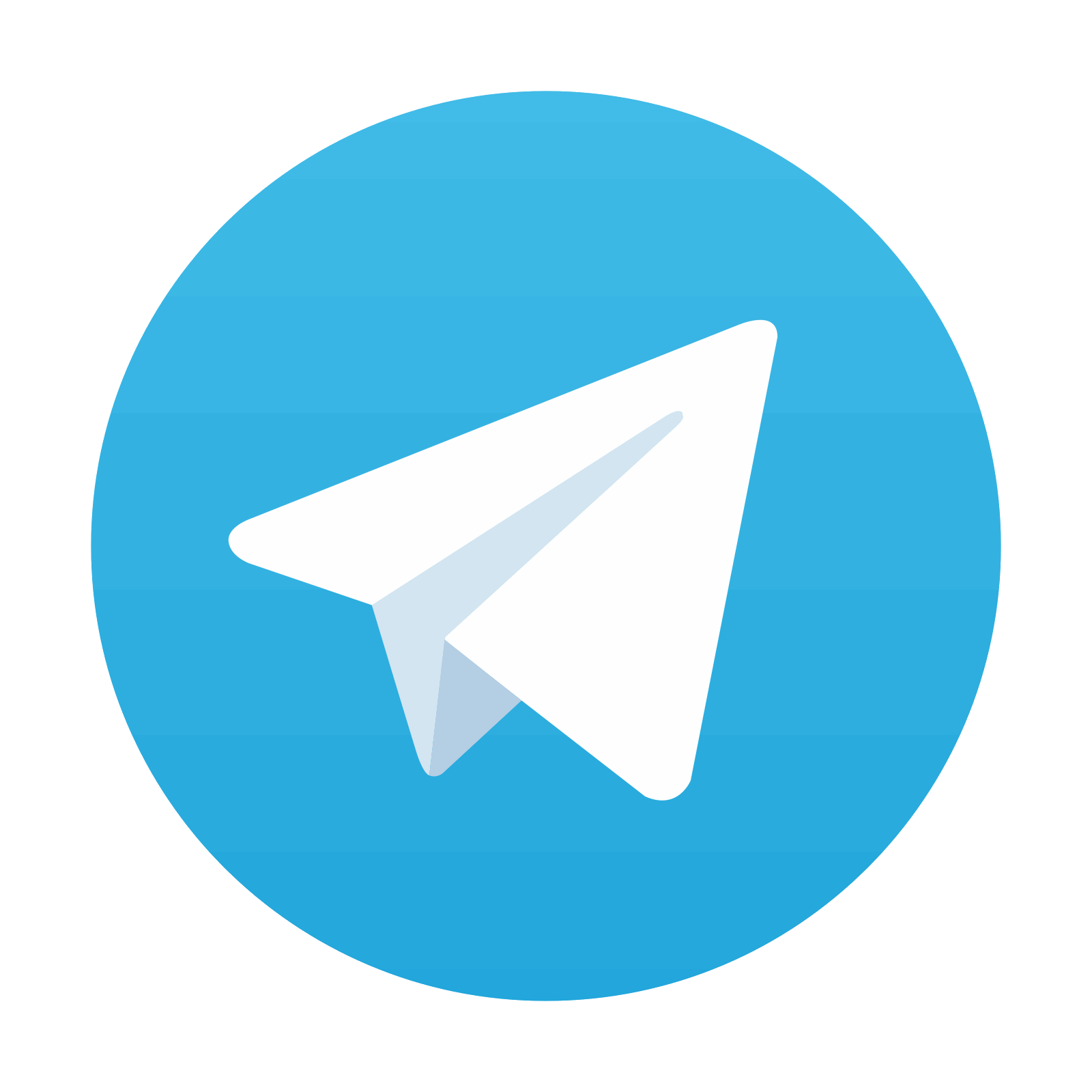
Stay updated, free articles. Join our Telegram channel

Full access? Get Clinical Tree
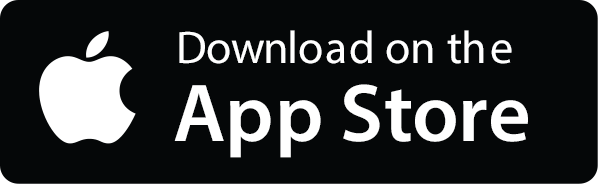
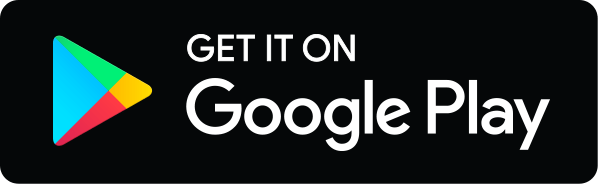