HISTORICAL PERSPECTIVE: EVOLVING CONCEPTS ON THE PATHOGENESIS OF MULTIPLE ORGAN FAILURE
Our increasing ability to keep severely injured patients alive eventually created a new clinical syndrome known as post-injury multiple organ failure (MOF). As advances in prehospital and acute hospital care conquered “the golden hour”; the trimodal distribution of trauma deaths described in the 1970s and 1980s slowly flattened its first mode and MOF emerged as the leading cause of late trauma death.1,2,3
Much of the advances in the treatment of trauma and shock have been stimulated by military experience (see Chapter 13)4 and refined by research in civilian trauma centers. In World War I, soldiers’ death on the battlefield was initially attributed to toxins released by dead or dying tissue (Table 61-1).5 Cannon et al, via observations on the battlefield in 1917, expanded this concept to question the role of hypovolemia; however, it was not until the 1930s that Blalock et al established that reduced circulating blood volume was the dominant cause of shock and mortality.6 Casualties in World War II were initially resuscitated with freeze-dried plasma and later with stored whole blood. This continued in Korea where blood and plasma were administered until blood pressure returned to normal. In addition to rapid transport for definitive care in field medical units, prompt blood and plasma resuscitation improved battlefield survival but resulted in late deaths due to oliguric renal failure. In the 1960s, Shires et al proposed that extracellular fluid deficits (third space losses) compounded traumatic shock and demonstrated in animals that survival improved with the addition of balanced salt solutions.7 Consequently, crystalloid resuscitation was added to blood and plasma resuscitation in the Vietnam War and resuscitation end points focused on maintaining adequate urine output. Helicopter evacuation enabled rapid transport of casualties and the overall mortality rate decreased. Although late deaths from renal failure declined, a new entity termed “shock lung” emerged as the primary cause of late deaths.5 This new disorder became recognized in civilian trauma centers, and was named the adult respiratory distress syndrome (ARDS).8 In the 1970s, subsequent improvements in advanced organ support such as mechanical ventilation (MV), vasoactive drugs, total parenteral nutrition, and hemodialysis armed physicians with better treatment to sustain the critically ill. Death from isolated pulmonary failure became rare, and a new syndrome of “multiple, progressive, or sequential systems failure” was recognized.9
Time period | Proposed etiology | Interventions | Clinical patterns |
---|---|---|---|
World War I | Wound toxins | Undefined | Cardiac failure |
World War II | ↓ Blood volume | Resuscitate to normal SBP | Renal failure |
Vietnam War | ↓ Blood volume ↓ Extracellular fluid | Resuscitate to normal urine output Crystalloid resuscitation | Respiratory failure |
Mid-1970s | Shock Advanced age sepsis | Advanced organ support | Multiple organ failure |
Early 1880s | Uncontrolled infection | Prevent and treat septic complications | Infectious models |
Late 1980s | Systemic inflammation Bacterial translocation | Control inciting event Attenuate early inflammation | Inflammatory models |
1990s | SIRS/CARS Abdominal Compartment Syndrome | Improved resuscitation end points Avoid secondary events | Dysfunctional inflammation |
2000 to present | Cellular and subcellular signaling | Immunomodulation, Standard Operating Procedures Early sepsis intervention | Decreased MOF incidence and severity |
2011 to present | Genetic storm |
Eiseman et al coined the term “multiple organ failure” at the Denver General Hospital (now known as Denver Health Medical Center) in 1977, and provided the first clinical description of 42 patients with progressive organ dysfunction.10 During the late 1970s MOF was thought to be the “fatal expression of uncontrolled infection.”11 In addition, at this time, studies indicated that organ failure was a bimodal phenomenon with distinct patterns: rapid single-phase MOF due to massive tissue injury and shock or delayed two-phase MOF due to moderate trauma and shock followed by delayed sepsis.12,13 While infection remained a frequent cause of organ failure, by the mid-1980s it was convincingly shown that organ failure occurred in the absence of infection and the concept of “generalized auto-destructive inflammation” emerged.14,15
By the 1990s, noninfectious inflammatory models of MOF became the focus.16 In this conceptual framework, patients are resuscitated into a state of early systemic hyperinflammation, referred to as the systemic inflammation response syndrome (SIRS). A mild response is presumed to be beneficial and resolves in most patients as they recover. In the “one-event” model, a massive traumatic insult overwhelms the capacity of the patient to respond to resuscitation and precipitates organ failure. In the more common, alternative “two-event” model, patients who are initially resuscitated to a moderate response (primed) are vulnerable to a second (activating) event that can also precipitate hyperinflammation leading to early organ failure. The timing of the second hit may vary from occurring shortly after the first hit, where the two are indistinguishable, to a delayed event conspicuous 12–36 hours later. Patients who escape early MOF become susceptible to infection during the so-called compensatory anti-inflammatory response syndrome (CARS). If infected during this window, patients are at risk of developing late MOF. Other second hits include abdominal compartment syndrome (ACS), sepsis, fat embolus, MV, blood transfusions, long bone fixation, and other secondary surgical procedures.17 ACS, in particular, emerged in epidemic proportions in the early 1990s as a result of tremendous advances in trauma care in the 1980s (including ATLS, trauma systems, damage control, and surgical critical care) which decreased the number patients bleeding to death early after hospital admission. This proved to be an iatrogenic complication that has largely been eliminated by massive transfusion protocols with avoidance of excessive crystalloids, earlier hemorrhage control and abandonment of supranormal oxygen delivery resuscitation directed by pulmonary artery catheter.
In the late 2000s, the MOF pathogenesis model (Fig. 61-1) proposed that injury triggered simultaneous, opposite responses: the proinflammation (SIRS) and the anti-inflammation (CARS).18 The term “compensatory” inflammation is now considered a misnomer as CARS appears to occur in response to the injury (not to SIRS). In this paradigm, the development of MOF is related to the intensity and the balance between these opposing inflammatory responses to trauma. Severe SIRS, due to unbalanced early proinflammation via activation of the innate immune system, causes early MOF and can eventually result in a fulminant proinflammatory death. On the other hand, early anti-inflammation, via inhibition of the adaptive immune system and apoptosis, is directed at limiting proinflammation, creating a preconditioned state where the host is protected against second hits, and hastening the healing process. When countering unbalanced proinflammation, persistent anti-inflammation can result in a severe systemic anti-inflammatory response syndrome (SARS, a proposed, more appropriate terminology for CARS), setting the stage for immunoparalysis, impaired wound healing, recurrent nosocomial infections, and late MOF.18,19
More recently, the Inflammation and Host Response to Injury Large-Scale Collaborative Research Program (Glue Grant), sponsored by the National Institutes of Health (NIH), confirmed that alterations in the genomic expression of the classical inflammatory and anti-inflammatory responses occurred simultaneously.20
These new constructs evolved from translational research, a two-way exchange between clinical observations and bench-level experimental studies that remains the foundation of contemporary MOF research. Research during the past decade has progressed toward further explaining these inflammatory mechanisms at the cellular and subcellular levels. New strategies to reduce secondary insults and to modulate the inflammatory responses in the early and late post-injury periods are currently undergoing intense research investigation.
In the 2000s, there were two major ICU initiatives that reduced ICU deaths. The first was the Glue Grant Standard Operating Procedures, which ensured consistent implementation of evidence-based care for standard ICU interventions.21 The second was the Surviving Sepsis Campaign, which emphasized sepsis screening and rapid implementation of a sepsis bundle, including antibiotics within 1 hour and volume resuscitation.22 As a result, patients are now surviving longer in ICUs with low-level organ dysfunction and are being discharged to long-term acute care facilities. There they suffer an indolent death from a newly described MOF phenotype, the persistent inflammation-immunosuppression catabolism syndrome (PICS), described later in the chapter.20 In sum, the phenotypic expression and the epidemiology of post-injury MOF has changed considerably over the past 20 years. Yet, as we will describe in the next sections, MOF remains morbid, lethal, and resource intensive.21
There is no specific measurement that identifies MOF. Thus, defining MOF given the absence of a definitive gold standard is a challenge.23 The syndrome seems to fit well with Justice Stewart’s famous quote: “I may not be able to define it, but I know it when I see it.” As a consequence, researchers have used different definitions for the syndrome resulting in widely different epidemiological descriptions.
Since there is no gold standard, validation of current definitions must be done examining the association of different scores with objective adverse outcomes, reflecting clinical status (VFD: ventilator-free days, IFD: intensive-care-free days, and death) and resource utilization (length of ICU stay [ICU-LOS] and MV days). VFD and IFD have been recently proposed as alternative outcomes for critically ill patients that account for patients who died early and consequently had shorter MV and ICU times.24 The validation process can be greatly enhanced if conducted on a homogenous population observed for an extended period of time, as in our long-term Denver MOF dataset. Covering a long period of time (1992–2008), this database contained prospectively collected clinical data on patients at risk for post-injury MOF, for the first 28 post-injury days. To our knowledge, this is the longest, sustained prospective database on post-injury MOF using standardized data collection methods. Acutely injured patients admitted to the surgical intensive care unit (SICU) at the Denver Health Medical Center (DHMC, a state-designated level I trauma center verified by the American College of Surgeons Committee on Trauma) were studied prospectively.
An analytic dataset of 1440 patients from 1992 to 2004 enrolled in the Denver MOF dataset, was used for a validation of two widely used MOF definitions: the Denver score25 and the Multiple Organ Dysfunction Score (MODS).26 For other scores, the reader is referred to the excellent review by Baue.27 The Denver score, first proposed by our group in 1991, originally included eight organ systems (cardiovascular, pulmonary, renal, hepatic, gastrointestinal, hematologic, central nervous system [CNS], and metabolic). The score was later revised and gastrointestinal, hematologic, CNS, and metabolic failures were excluded since their addition did not improve the characterization of MOF. In brief, the Denver score grades on a scale from 0 to 3 the dysfunction of four systems (pulmonary, renal, hepatic, and cardiovascular), evaluated daily throughout the patient’s ICU stay with the total score ranging from 0 to 12 (Table 61-2). The Marshall MODS was developed in 1994 and validated based on probability of subsequent mortality in a sample of 692 patients from a Canadian ICU.26,28 It grades the dysfunction of six organ systems (pulmonary, renal, hepatic, cardiovascular, hematologic, and neurologic) from 0 to 4 (Table 61-3). For both scores, organ dysfunction must be graded daily, and the sum of simultaneously obtained individual organ scores corresponds to the daily MOF score. The Denver MOF score ranges from 0 to 12, and MOF is declared when the daily score is greater than 3. The authors of the MODS did not propose a cutoff to declare MOF, but traditionally a daily score greater than 5 has been used.
Organ system | Grade 0 | Grade 1 | Grade 2 | Grade 3 |
---|---|---|---|---|
Pulmonary | ||||
PaO2/FIO2 ratio | >250 | 250–200 | 200–100 | ≤100 |
Renal | ||||
Creatinine (mg/dL) Creatinine (μmol/L) | ≤1.8 <159 | 1.9–2.5 160–221 | 2.51–5.0 222–442 | >5.0 >442 |
Hepatic | ||||
Bilirubin (mg/dL) Bilirubin (μmol/L) | ≤2.0 <34 | 2.0–4.0 34–68 | 4.1–8.0 69–137 | >8.0 >137 |
Cardiac | No inotropes | Only one inotrope at a small dosea | Any inotrope at moderate dose or >1 agent, all at small dosesa | Any inotrope at large dose or >2 agents at moderate dosesa |
Small | Moderate | Large | ||
Milrinone | <0.3 | 0.4–0.7 | >0.7 | |
Vasopressin | <0.03 | 0.03–0.07 | >0.07 | |
Dopamine | <6 | 6–10 | >10 | |
Dobutamine | <6 | 6–10 | >10 | |
Epinephrine | <0.06 | 0.06–0.15 | >0.15 | |
Norepinephrine | <0.11 | 0.11–0.5 | >0.5 | |
Phenylephrine | <0.6 | 0.6–3 | >3 |
Dysfunction | Grade 0 | Grade 1 | Grade 2 | Grade 3 | Grade 4 |
---|---|---|---|---|---|
Pulmonary | |||||
PaO2/FIO2 ratio | >300 | 226–300 | 151–225 | 76–150 | <75 |
Renal | |||||
Creatinine (μmol/L) | <100 | 101–200 | 201–350 | 351–500 | >500 |
Hepatic | |||||
Bilirubin (μmol/L) | <20 | 21–60 | 61–120 | 121–240 | >240 |
Cardiac | |||||
1994 version 1995 versionb | Heart rate <120 PAR <10 | Heart rate 120–140 PAR 10.1–15 | Heart rate >140 PAR 15.1–20 | Inotropesa PAR 20.1–30 | Lactate >5 mmol/L PAR >30 |
Central nervous system | |||||
Glasgow Coma Scale | 15 | 13–14 | 10–12 | 7–9 | <6 |
Hematologic | |||||
Platelet count × 103/mL | >120 | 81–120 | 51–80 | 21–50 | <20 |
Patients fitting the following criteria were entered in the Denver MOF dataset:
Moderate to severe injury (injury severity score [ISS] >15)—patients with this level of injury or worse are all likely to be admitted to the ICU, minimizing selection bias.
Survival longer than 48 hours from injury—studies at the time of this validation demonstrated that physiologic derangements prior to 48 hours were confounded by the primary injury or incomplete resuscitation.29
Admission to DHMC within 48 hours of injury—due to delay in admission, early risk factors shown to be associated with MOF were not observed or recorded.
Age greater than 15 years—it has been demonstrated that MOF presents differently among children, and that the currently used organ dysfunction measures may not be appropriate to the pediatric population; thus, children were not included in this analysis.30
No isolated traumatic brain injury (TBI)—nonneurologic organ dysfunctions in TBI patients seem to have a different pathophysiology and presentation compared to those with torso injuries.31
In addition, we excluded patients with burn and/or hanging injuries, as they were most often treated primarily at other centers and their risk factors for MOF are different from torso injuries. Daily physiologic and laboratory data were collected through day 28. There were 1389 patients, typically young, healthy males, with moderate to severe injuries, mostly due to blunt force in motor vehicle accidents. Mortality and number of VFD days were low, but health care resource utilization was high, with over one-third of these patients requiring longer than 14 admission days in the surgical ICU (LOS) and/or more than 7 days of MV. The incidence of MOF was 22% with a case-fatality rate of 30% according to the Denver MOF score, as opposed to an incidence of 50% with a case-fatality rate of 15% according to the MODS (using the traditional >5 cutoff). When comparing the patients identified by each score, we noted three major groups: (1) a severe injury group of patients classified by both scores as MOF with high rates of risk factors (high ISS, shock, and advanced age), mortality and health care utilization, (2) a moderate injury group classified as MOF by the MODS but not by the Denver MOF score with medium risk factor rates, medium health care utilization and low mortality, and (3) a mild injury group which included patients classified as no MOF by both scores for whom risk factor rates, utilization, and mortality were all low. These findings suggest that the MODS tends to be more sensitive, while the Denver MOF score tends to be more specific.
We used the area under the receiver-operating characteristics (AUROC) curve to compare the association of the two scores (Denver MOF score and MODS) with the objective adverse outcomes. (death, VFD <21 days, ICU-LOS >14 days, and MV >7 days). Figure 61-2 shows the two scores’ AUROCs for mortality. Overall, both scores were associated with areas greater than or equal to 75 for all outcomes, and greater than or equal to 80 for mortality (ideal value = 100). The AUROC for the Denver score was slightly greater than that for the Marshall score regarding prediction of death, VFD, and MV. The differences, however, were not significant, suggesting similar, good performances for both scores.
Since its initial description, MOF’s incidence has changed dramatically, although some controversy remains. Indeed, large US and Australian studies have shown a steady decline in the incidence of MOF over the past decade, likely due to new therapeutic approaches.32,33,34,35 Conversely, a large German study reported an increase in the MOF incidence.36 Yet, the different reports unanimously recognized that the mortality and morbidity associated with MOF remain high.32,33,34,36,37,38,39,40,41
The controversies on MOF incidence are related to a number of factors, including disparate definitions, as highlighted above, as well as differences in populations and resuscitation practices. We recently conducted a large, comprehensive examination of the recent trends in post-injury MOF using the aforementioned Glue Grant dataset.33 This contemporary, longitudinal, prospective study includes several US trauma centers who shared standard operating procedures (SOPs) minimizing intervention heterogeneity.42 SOPs included: early resuscitation, mechanical ventilation (including guidelines for ventilator discontinuation), ventilator-associated pneumonia, insulin infusion, nutrition and venous thromboembolism prevention.42 Our hypotheses were (1) the incidence of post-injury MOF has decreased and (2) rates of MOF-related morbidity and death have not changed significantly. Adult patients with severe blunt torso injuries and hemorrhagic shock were enrolled from 2003 to 2010 and followed through day 28 or discharge.
We studied 1643 patients enrolled from 2003 to 2010 by four centers that enrolled more than 20 eligible patients per biennial. Of these, 252 (15%) did not survive. MOF developed in 223 (13.6%) patients of whom 81 (36%) died. Table 61-4 details the distribution of admission risk factors, fluids, transfusions, complications, outcomes as well as MOF incidence and MOF-related outcomes across the biennial periods. Anatomic injury severity remained relatively stable while measures of shock upon admission had a slight improvement over time. Blood transfusions remained stable while 12 hours crystalloids’ volume decreased. MOF crude incidence decreased over time (p = 0.0033), while MOF-related mortality, VFD and IFD remained stable at high levels. The Kaplan-Meyer analysis confirmed these findings (Fig. 61-3). Applying the MODS definition produced similar results, that is, a significant decline in MOF incidence and no change in MOF mortality over time.
2003–2004 | 2005–2006 | 2007–2008 | 2009–2010 | p-valuea | |||||||||
---|---|---|---|---|---|---|---|---|---|---|---|---|---|
N = 335 | N = 506 | N = 546 | N = 256 | ||||||||||
Variable | Median or % | LQ | UQ | Median or % | LQ | UQ | Median or % | LQ | UQ | Median or % | LQ | UQ | |
Demographic | |||||||||||||
Age (years) | 40 | 26 | 54 | 41 | 26 | 54 | 43 | 28 | 57 | 45.5 | 25.5 | 58 | 0.0246 |
Body Mass Index (kg/m2) | 25.7 | 23.1 | 29.9 | 26.7 | 23.7 | 31.4 | 27.1 | 23.8 | 31.3 | 27.3 | 24.1 | 32.0 | 0.0017 |
Male sex (%) | 64.5 | 66.6 | 67.2 | 67.6 | 0.3940 | ||||||||
Comorbidity Index ≥2 (%) | 8.4 | 12.9 | 8.4 | 10.2 | 0.8276 | ||||||||
Antiplatelet therapy (%)b | 6.6 | 7.1 | 10.1 | 8.2 | 0.1414 | ||||||||
Injury | |||||||||||||
Moderate TBI (%)c | 30.2 | 18.0 | 21.6 | 18.0 | 0.0037 | ||||||||
Injury severity score | 29 | 22 | 41 | 32 | 22 | 41 | 34 | 24 | 41 | 34 | 22 | 43 | 0.0622 |
Prehospital GCS | 13 | 4 | 15 | 14 | 10 | 15 | 14 | 9 | 15 | 14 | 9 | 15 | <0.0001 |
Prehospital SBP (lowest) | 89.0 | 72.5 | 104.5 | 86.0 | 71.0 | 102.0 | 88.0 | 73.0 | 108.0 | 85.0 | 74.0 | 100.0 | 0.8425 |
Prehospital HR (highest) | 116 | 95 | 131 | 118 | 100 | 130 | 115 | 97 | 130 | 118 | 102 | 132 | 0.4810 |
Admission GCS | 6 | 3 | 15 | 10 | 3 | 15 | 11 | 3 | 15 | 3 | 3 | 15 | 0.1534 |
Admission SBP (mm Hg) | 110 | 93 | 135 | 111 | 90 | 132 | 110 | 90 | 131 | 109.5 | 89 | 128 | 0.0429 |
Admission SBP ≤90 mm Hg | 22.1 | 25.9 | 26.7 | 29.9 | 0.0350 | ||||||||
Admission HR (beats/min) | 108 | 86 | 127 | 110 | 90 | 126 | 109 | 91 | 127 | 110 | 92 | 127.5 | 0.2080 |
Fluids/blood | |||||||||||||
RBC units/12 h | 5 | 3 | 11 | 6 | 3 | 12 | 5 | 2 | 9 | 5 | 2 | 9 | 0.0739 |
FFP units/12 h | 3 | 0 | 8 | 3 | 0 | 8 | 2 | 0 | 6 | 3 | 0 | 7 | 0.0798 |
0–6 h RBC:FFP ratio | 0.6 | 0 | 1.5 | 0.5 | 0 | 1.6 | 0 | 0 | 1.5 | 0.6 | 0 | 1.4 | 0.1224 |
Platelet units/12 h | 0 | 0 | 1 | 0 | 0 | 1 | 0 | 0 | 1 | 0 | 0 | 1 | 0.4160 |
Prehospital crystalloids (mL) | 1.6 | 0.6 | 3.0 | 1.8 | 0.7 | 3.3 | 1.4 | 0.5 | 2.9 | 1.8 | 0.7 | 3.1 | 0.3504 |
Crystalloids (mL)/12 h | 10.3 | 7.2 | 15.7 | 10.0 | 7.6 | 13.6 | 8.7 | 5.9 | 12.3 | 9.0 | 6.3 | 12.0 | <0.0001 |
LABORATORIAL TESTS | |||||||||||||
ED Base Excess (mEq/L) | –8.9 | –11.4 | –6 | –8.4 | –11.2 | –6 | –7.6 | –0.8 | –5 | –8 | –11.1 | –5.35 | 0.0012 |
ED Lactate (mg/dL) | 4.3 | 3 | 5.9 | 3.9 | 2.7 | 5.6 | 3.6 | 2.4 | 5.2 | 4 | 2.4 | 6 | 0.0016 |
Day 1 Platelet 1000/mcL | 100 | 79 | 129 | 95 | 76 | 123 | 107 | 87 | 133 | 101 | 84 | 128.5 | 0.0028 |
PaO2/FIO2 ratio/12 h | 119 | 66 | 213 | 161 | 86.5 | 256 | 158 | 89 | 269 | 163 | 79 | 285 | 0.0003 |
ED Hemoglobin g/dL | 10.9 | 9.33 | 13 | 11.3 | 9.5 | 13.1 | 11.9 | 10 | 13.3 | 11.5 | 9.6 | 12.9 | 0.0217 |
ED INR | 1.2 | 1.1 | 1.5 | 1.3 | 1.1 | 1.5 | 1.21 | 1.1 | 1.5 | 1.3 | 1.1 | 1.5 | 0.3152 |
Complications | |||||||||||||
Nonseptic complication (%) | 44.5 | 47.2 | 44.1 | 40.4 | 0.2365 | ||||||||
Surgical site infection (%) | 13.1 | 16.4 | 14.8 | 8.2 | 0.1180 | ||||||||
VAP (%) | 26.6 | 26.5 | 24.4 | 23.1 | 0.2335 | ||||||||
Outcomes | |||||||||||||
Multiple organ failure (%) | 17.0 | 15.0 | 11.9 | 9.8 | 0.0033 | ||||||||
Lung failure (%) | 57.6 | 56.5 | 55.3 | 50.8 | 0.1073 | ||||||||
Cardiac failure (%) | 20.9 | 17.6 | 16.1 | 12.5 | 0.0064 | ||||||||
Liver failure (%) | 15.2 | 16.2 | 13.4 | 14.1 | 0.3762 | ||||||||
Renal failure (%) | 10.1 | 10.7 | 11.9 | 12.5 | 0.2804 | ||||||||
ICU days | 8 | 4 | 19 | 9 | 4 | 17 | 10 | 5 | 18 | 9 | 5 | 17 | 0.1070 |
ICU-free days | 11 | 0 | 21 | 15 | 4 | 23 | 15 | 3 | 22 | 17 | 8 | 22 | 0.0002 |
Ventilator days | 6 | 2 | 14 | 5 | 2 | 13 | 7 | 2 | 13 | 6 | 2 | 12 | 0.8414 |
Ventilator-free days | 16 | 0 | 24 | 19 | 7 | 25 | 20 | 8 | 25 | 21 | 12 | 25 | <0.0001 |
Mortality (%) | 23.9 | 15.4 | 12.3 | 10.5 | <0.0001 | ||||||||
MOF related outcomes | |||||||||||||
Case fatality (%) | 33.3 | 38.2 | 36.9 | 36.0 | 0.7800 | ||||||||
ICU days | 22 | 9 | 34 | 17 | 8.5 | 8.0 | 15 | 9 | 27 | 19 | 10 | 24 | 0.1889 |
ICU-free days | 0 | 0 | 4 | 0 | 0 | 8 | 0 | 0 | 10 | 4 | 0 | 7 | 0.2289 |
Ventilator days | 20 | 9 | 26 | 15 | 6.5 | 27 | 12 | 7 | 21 | 13 | 6 | 19 | 0.0642 |
Ventilator-free days | 0 | 0 | 8 | 0 | 0 | 11.5 | 0 | 0 | 14 | 4 | 0 | 14 | 0.1969 |
MOF related complications | |||||||||||||
Nonseptic complication (%) | 77.2 | 75.0 | 83.1 | 80.0 | 0.4481 | ||||||||
Surgical site infection (%) | 22.8 | 27.6 | 16.9 | 20.0 | 0.4042 | ||||||||
VAP (%) | 47.3 | 43.4 | 50.8 | 44.0 | 0.8946 |
A Cox proportional hazards (Cox PH) model demonstrated that the MOF significant downward trend from 2003 to 2010 was independent from included admission risk factors (p < 0.0001). The adjusted MOF risk decreased by 16% (hazard ratio = 0.86) by each period of 2 years for an estimated 50% decrease over the entire study period. Conversely, adjusted MOF-related death did not change over time (p = 0.6907). Inclusion of crystalloids and blood component transfusions did not significantly alter these results.
The risk factors for developing MOF included demographic characteristics (advanced age, male sex, obesity), injury severity (higher ISS), and physiologic derangement (admission acidosis, number of transfused units of red blood cells [RBC]/12 hours). MOF-related death was positively associated with female sex, ISS and number of RBC units transfused in the first 12 hours post-injury.
Lung failure incidence decreased significantly over time, but remained the most common organ failure over the study time period affecting over half of these patients (see Table 61-4). Cardiovascular dysfunction, as measured by inotrope requirement, also became significantly less frequent, while renal and liver failures persisted at similar levels. Adjustment for admission risk factors confirmed these results.
The mortality associated with individual organ failures (with or without involvement of other organs) was highest for cardiovascular dysfunction (39%), followed by failure of the kidneys (38%), liver (19%), and lungs (12%). Only 1% of the patients had isolated dysfunction of the kidneys, liver, or heart. Mortality for isolated cardiovascular dysfunction was 58%, while for renal failure was 15%; none of the patients with isolated liver dysfunction died. In contrast, 32% of the patients in this series had isolated lung dysfunction, and this rate remained relatively stable over the study period (from 2003 to 2010). This suggests that there was a decrease in the progression from lung dysfunction to MOF over time.43 On the other hand, it also suggests that we have advanced little in preventing lung dysfunction. Mortality of isolated lung failure remained stable over time (3–6%). MOF without lung dysfunction is rare: only 8% of the MOF patients did not have lung involvement.
MOF onset retained the traditional multimodal distribution (Fig. 61-4A), with the highest peak within the first 3 days post-injury and a smaller peak afterward. Approximately half of MOF cases occurred within 3 days (Fig. 61-4B). The onset of individual organ failure varied little over the biennials. Lung, cardiac, and renal failures were early events occurring on days 2 or 3, while liver failure is usually detected later, around day 6.
The time interval between MOF onset and death is usually short, with death ensuing in 2 days in 58% of the cases and within a week in 80% of the cases. Early MOF (within 3 days post-injury) carried a higher mortality than later MOF (Fig. 61-4B). In the biennial 2009–2010, 50% of the early MOF patients died, compared to 40% of those with MOF onset between 4 and 7 days and none of those with late MOF.
The Burden of MOF: MOF patients in this multicenter series endured longer ICU stays (median 18 days; IQR 9–29) compared to all non-MOF patients (median 8 days; IQR 4–16). Similarly, MOF victims required much longer ventilation (median 14 days; IQR 7–24) compared to non-MOF patients (median 5 days; IQR 2–12). Stratification by MOF and survival status showed that MOF survivors were the group who demanded more ICU and ventilation resources. MOF survivors were responsible for 20% of the total ICU and mechanical ventilation days invested in the critical care of this population even though they represented only 9% of the total population.
Based on national estimates of critical care costs,44 the total cost of the critical care delivered to MOF patients in this dataset amounted to $19,990,420 (ICU with mechanical ventilation = $16,479,606 + ICU without mechanical ventilation = $3,510,814). This corresponded to 22% of the total ICU cost for this population (ICU with mechanical ventilation = $69,238,641 + ICU without mechanical ventilation $20,580,321 = Total ICU cost $89,818,962). The estimated median cost per MOF patient was $77,202, compared to $38,442, which was the presumed cost of caring for non-MOF patients.
Initial responses to trauma involve several systems: hemostatic, inflammatory, endocrine and neurological. The two-hit model of post-injury MOF, described in a previous section and illustrated in Fig. 61-1, was derived from a two-way translational model in which information was exchanged between epidemiological studies, predictive models, and basic investigations using in vivo and in vitro models. As mentioned above, severe trauma patients are resuscitated into an early state of systemic inflammation or SIRS. The American College of Chest Physicians and the Society of Critical Care Medicine defined SIRS as two or more of the following criteria: (1) temperature less than 36.8°C or greater than 38.8°C, (2) heart rate more than 90/min, (3) respiratory rate more than 20 breaths/min or pco2 less than 32 mm Hg, and (4) WBC less than 4000/mL or greater than 12,000 mL or greater than 10% immature forms.
The current framework for the immunoinflammatory response to trauma is illustrated in Fig. 61-5. SIRS is the manifestation of the immunoinflammatory activation that occurs in response to ischemia–reperfusion (I/R) injury and factors released from disrupted tissue. The injured cell releases endogenous damage-associated molecular patterns (DAMPs, alarmins), which are analogous to the microbial pathogen-associated molecular patterns (PAMPs), released in sepsis.45,46,47 This analogy is not surprising as the human mitochondria derived from bacterial endosymbionts during the evolutionary process. Both PAMPS and DAMPS/alarmins activate innate immunity.
Liu et al48 showed that up to 70% of unique proteins measured in the plasma of blunt trauma victims were intracellular molecules that could function as DAMPS/alarmins and trigger pattern recognition receptors. Our laboratory was the first to describe the proteome description of human mesenteric lymph collected from critically ill or injured patients using a label-free semiquantitative mass spectrometry based approach.49 A total of 477 proteins were identified, including markers of hemolysis, extracellular matrix components, and general tissue damage in addition to the classical serum proteins. Hemolysis, a common event post-trauma, releases hemoglobin to the extracellular environment, where it becomes a redox-reactive DAMP molecule.50 Extracellular hemoglobin can also bind to PAMPs and triggers toll-like receptor-mediated signal transduction. High levels of extracellular hemoglobin can generate reactive oxygen species, which affects the innate immunity.
Among the proteins we identified in the mesenteric lymph, there were several markers of tissue damage including the cytoskeletal proteins actin, tubulin, cofilin-1, spectrin, gelsolin, and profilin-1.49 We also detected 16 mitochondrial proteins suggesting the presence of lysed mitochondria, presumably from cell death. There have been reports showing that circulating mitochondrial DNA and formyl peptides can mediate organ injury through PMN activation.45
We recently performed a mass spectrometric analysis of the plasma metabolome of severely injured patients, including a subgroup that underwent resuscitative thoracotomy in the Emergency Department of the Denver Health Medical Center, compared to healthy controls.51 Metabolomics describes the metabolites present in a biologic matrix and is reflective of the host’s pathologic state or response to stimuli. We observed a trauma-dependent metabolic state of hypercatabolism that provides carbon and nitrogen sources to compensate for trauma-induced energy consumption and negative nitrogen balance. This state is characterized by sugars consumption, lipolysis and fatty acid use, accumulation of ketone bodies, proteolysis and nucleoside breakdown. Unexpectedly, metabolites of bacterial origin (including tricarballylate and citramalate) were detected in the plasma of these trauma patients (see the section “Role of the Gut” later in the chapter).
Plasma from trauma patients showed accumulation of mannitol, heme, and oxidative stress markers.51 It should be noted that mannitol’s increase could reflect also therapy (as it is present in blood transfusions), although our data suggested limited transfusion effect, as we detected no difference in metabolite levels between resuscitative thoracotomy patients (who received limited transfusions) and other trauma patients, whose blood samples preceded intravenous fluid or blood products.
This metabolomic analysis confirmed the presence of an altered lipidomic profile, a hallmark of trauma-induced metabolic adaptation.51 Fatty acid mobilization (accumulation of acylcarnitines) and lipid breakdown (buildup of ketone bodies and breakdown products of fatty acids—eg, choline, glycerol, ethanolamine, glycerol phosphate, and glycerophosphocholine moieties) were observed. In addition, elevations in post-injury proinflammatory arachidonate metabolites (prostaglandin E2 and leukotriene B4) supported the proposed immunomodulatory effect of diets balancing the ratio of omega-3 and omega-6 fatty acids in reducing post-injury MOF.52 Moreover, the hyperactivation of lipid metabolism in response to trauma results in the accumulation of anionic compounds such as ketone bodies, further promoting acidosis.
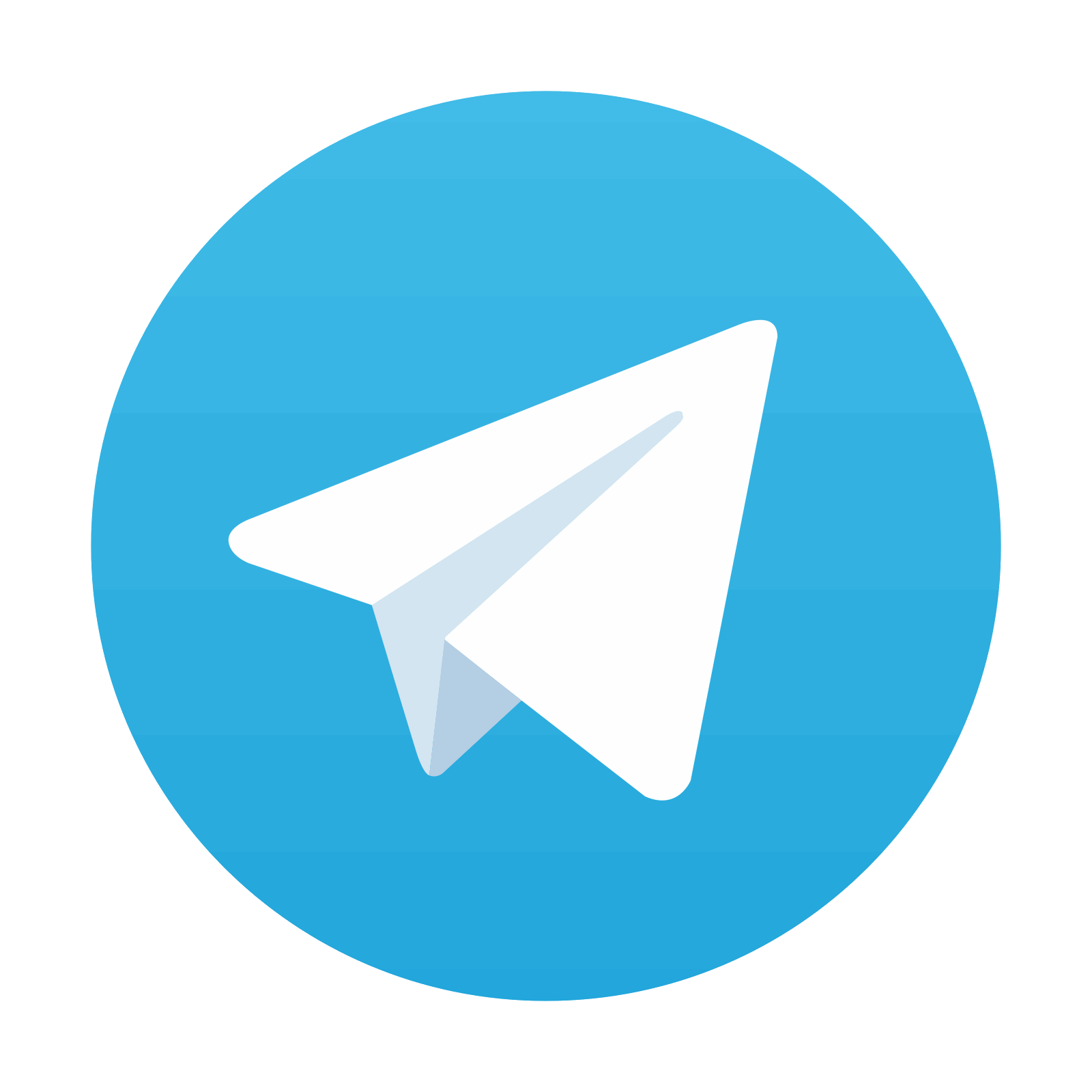
Stay updated, free articles. Join our Telegram channel

Full access? Get Clinical Tree
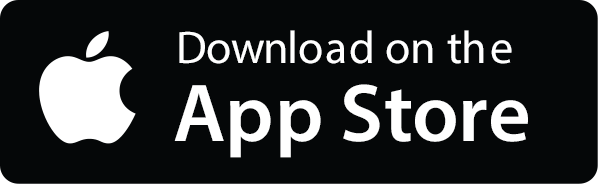
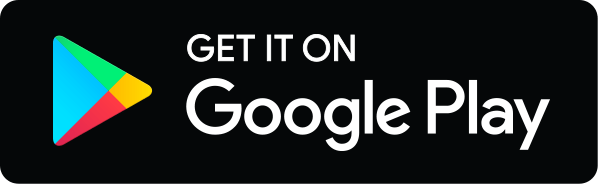