Method
Total white blood cells
Platelets × 1011
References
Mechanical apheresis
Cobe spectra V5-LRS
<1 × 106
2.56–7.41
[15]
Fenwal amicus
<1 × 106
2.82–7.14
[15]
Cobe V7 turbo
<1 × 106
2.80–7.54
[15]
Filtration—6 pooled units
Imugard IG 500
0.5–2 × 106
3.1–4.0
PALL PL100
5–6 × 105
3.5–4.0
[26]
Sepacell PL
0.1–5 × 106
3.1–4.0
[27]
Historical centrifugation studies of 6 pooled units
5–7 × 108
3.0–4.0
[28]
Single units of platelets derived from PRP or BC can be leukodepleted upon pooling, prior to transfusion, in the blood center, or at the bedside. Leukocyte-adherent filters are utilized for this process. The initial materials utilized were cotton wool and cellulose (Imugard) [25].
Initial studies of nonwoven polyester filters were utilized at bedside or in the blood bank producing adherence of the leukocytes to the filters (Sepacel, Baxter/Fenwal; Pall, Pall Corp) [26, 29]. Table 56.1 summarizes the content of the resulting platelet products. Additional investigations of materials and different structures of materials for leukodepletion have been conducted [27].
Many blood centers are also evaluating the prefiltering of products for distribution, and in-line filters have been tested for use during red cell collection and during apheresis. One study has shown no increased risk of bacterial growth in platelet concentrates as a result of prestorage white cell reduction [30]. There has been advocacy for prestorage leukodepletion to diminish cytokine production by leukocytes during storage [31, 32]. Others have demonstrated that poststorage leukodepletion may be more useful in removing complement and reducing reactions [33]. Additional research in leukodepletion technology is attempting to design filters capable of removing prions [34]. These issues point out the complexity of the ongoing clinical investigation of leukodepleted blood products and attempts to define the role and extent of leukodepletion of blood products [32].
Platelet Storage
The increased demand for platelet transfusions as well as the delay imposed by requirements for multiple pretransfusion infectious agent product screenings has stimulated ongoing research in the technology of platelet storage and methods for evaluation of platelet survival [35]. Many physical factors influence successful platelet storage. These include optimal storage temperature, maintenance of a certain range of plasma pH, suspension in an adequate volume of plasma, proper agitation during storage, and attention to the characteristics of the storage bag [36–39].
Storage conditions for individual platelet units derived from whole blood donations as well as for the storage of apheresis platelet collections have been defined and optimized based on the stability of storage pH and durability of platelet function [36–41]. It has been determined through numerous investigations that room temperature (22–24°C) storage is optimal for platelets [39, 40, 42, 43]. Despite the increased metabolic activity that occurs in platelets stored at 22°C, significantly better poststorage platelet viability at this temperature (compared with platelet concentrates stored at 4°C) has been confirmed by studies of transfusion response after storage at the two different temperatures [39, 40, 42, 43]. Studies suggests that even a brief reduction in storage temperature to 20°C during transport over long distances may have deleterious effects on subsequent platelet in vivo viability and in vitro function [44].
The storage bag characteristics have also been shown to be important in the maintenance of platelet viability [37, 38]. The development of new plastics and plasticizers and the production of larger and thinner bags have established a more stable storage environment, making possible extended storage of platelet concentrates and single-donor apheresis platelets [37, 45–48]. Storage bag qualities help to regulate oxygen and carbon dioxide exchange across the bag, which in turn regulates pH. The maintenance of plasma pH above 6.0 during storage preserves cell viability, although a very high pH can lead to irreversible platelet clumping [37, 39, 49]. Entry of adequate oxygen permits the maintenance of oxidative metabolism in platelets stored at room temperature, thus suppressing glycolysis (with its production of lactic acid) and preventing a fall in pH level. The egress of carbon dioxide across the bag surface also helps to maintain pH. The newer plastic bags provide increased oxygen transfer compared with the first-generation bags and have allowed stable, prolonged, storage with viability demonstrated after 5 or more days [45–48].
Agitation during storage of platelets is also important, since it prevents platelet “packing” and is believed to facilitate gas exchange through the storage bag [50, 51]. With the use of the permeable storage bags, it seems that the mode of agitation is less critical to platelet viability, and a variety of platelet rotators appear to be suitable for maintaining function [50, 51].
Additional studies have demonstrated, however, that even with the many improvements in technology, the collection and storage of platelets lead to platelet activation with a subsequent “storage lesion” [36, 52, 53]. Platelets appear to be activated throughout the process of collection, processing, and storage, demonstrating metabolic and functional signs of activation [36, 52]. Thus, stored platelets show less sensitivity to aggregating agents [54] and release increased amounts of platelet-specific proteins such as β-thromboglobulin [55, 56] with membrane expression of activation proteins such as P-selectin (CD62P) and glycoprotein IIb/IIIA [56–58]. Measurement of CD62P is being used in assessment of platelet activation in clinical trials [58]. Stored platelets have reduced platelet survival corresponding to the duration of storage [52, 53]. Snyder et al. have delineated multiple factors that impact platelet storage viability (Table 56.2) [8]. Future research in storage technology includes the possibility of inactivation of platelets during storage [59, 60] and the use of non-plasma-buffered storage solutions [61].
Table 56.2
Factors contributing to platelet storage lesion
During collection of platelets |
Type of anticoagulant |
Sheer effects from blood drawing—activation by catheter |
Time between whole blood collection and separation |
Temperature |
During storage of platelets |
Storage duration |
Temperature of storage |
Mode of agitation |
Storage container (gas exchange) |
Irradiation |
Leukodepletion filter (activation) |
Cryopreservation |
Cryopreservation
The technology for long-term frozen platelet storage has proved clinically helpful in the management of alloimmunized patients at the University of Maryland and Albert Einstein Cancer Centers [62, 63], and in Europe [64]. During recovery from chemotherapy-induced aplasia and achievement of remission, such patients often have rapidly rising platelet counts, and plateletpheresis during this period often provides a high yield of platelets for cryopreservation. Both autologous and HLA-typed platelets have been frozen for subsequent use in alloimmunized patients. Cryopreservation techniques using either dimethyl sulfoxide (DMSO) [65, 66] or glycerol [67, 68] have been described, but to date only the DMSO methodology has been reproducible enough for general usage. This technology calls for a slow infusion of DMSO into PRP to a concentration of 5 %. This is similar to the methodology used for marrow and stem cell cryopreservation. Recoveries are 50–70 % of fresh platelets, and satisfactory hemostatic results are documented; occasional alloimmunized patients are supported solely by cryopreserved autologous platelets [62, 63, 69].
Successful transfusion of platelets that have been stored at liquid nitrogen temperatures (−120°C) for 3 years or more has been documented [69]. This freezing methodology is also potentially applicable to collection and storage of platelets with specific HLA types or for the maintenance of emergency supplies. One report suggests that the addition of a small percentage of epinephrine to the DMSO cryopreservation solution may enhance the quality of the cryopreserved platelets, by increasing post-thawing function [70]. Finally, recent studies to attempt to improve on the frozen platelet methodology have confirmed prior data that despite the freezing injury, the platelets retain function and are able to aggregate, and that there is an in vivo improvement in function once the previously frozen platelets circulate [71].
Figure 56.1 shows the consistently good response to transfusion of autologous frozen platelets in a patient who was alloimmunized and refractory to transfusion from random donors. The patient received multiple courses of intensive postremission chemotherapy, each requiring transfusion support. As shown, the patient was an excellent and readily available source of platelets for herself.
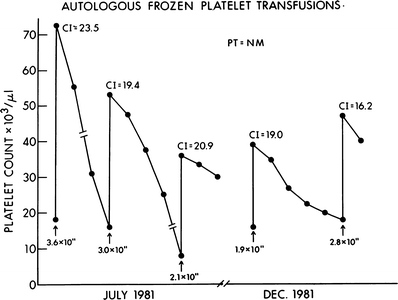
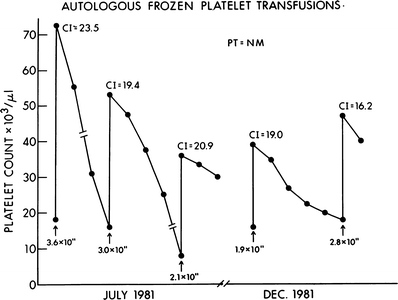
Fig. 56.1
Clinical effectiveness of autologous frozen platelets in an alloimmunized patient. This patient had episodes of mild bleeding, which responded to transfusions of autologous frozen platelet transfusions. The increments following transfusion were consistently good. CI corrected increment
Granulocyte Collection and Storage
Granulocyte collection technology has followed steps similar to those of platelet collection and utilizes the same principles of differential centrifugation to obtain the selected blood component. Modern techniques utilize the apheresis technology described in Chap. 54, with the best yields obtained with continuous-flow leukapheresis. Granulocytes are difficult to separate from whole blood because they are essentially the same density as RBCs. Therefore, whether apheresis or gravity leukapheresis is utilized, additional techniques are necessary to induce the efficient separation of granulocytes from RBCs [6, 72, 73].
This involves the use of sedimenting agents that induce the formation of erythrocyte rouleaux so that “clumps” of RBCs become heavier than granulocytes and the two cell types can be centrifuged apart. Hydroxyethyl starch (HES) and low molecular weight dextran have been used for this purpose [74]. Both agents coat the red cell surface, induce the formation of rouleaux, and allow efficient differential centrifugation, with sedimentation of the red cell clumps. Since granulocyte transfusions are collected from normal donors, the effect of starch exposure in donors has been evaluated. At 24 h, 50 % of the HES remains in the blood of the donor, and traces of the substance have been found as long as 8–9 months after a leukapheresis procedure [75]. No long-term effects have ever been reported in either donors or recipients in more than 25 years of leukapheresis utilizing this substance. HES is also widely used as a volume expander in surgical and intensive care patients with no long-term ill effects reported.
Granulocytes, particularly for adult recipients, are almost exclusively collected using apheresis machines (see Chap. 54 for details) [6, 73]. Techniques of granulocyte collection of historical interest include a manual technique called gravity leukapheresis, in which 1 U of whole blood was removed and sedimented with HES; the granulocytes were then separated, and the red cells were reinfused. This cycle was repeated until an adequate number of granulocytes were collected, similar to the gravity method for platelet collection [5, 72]. Reports from Japan have utilized a “simple bag method” to obtain granulocytes from growth factor-stimulated donors without apheresis for pediatric recipients [76]. Fortunately, modern apheresis and donor preparation techniques (see discussion to come) provide increased granulocyte yields for transfusions for adults with minimal granulocyte activation [77, 78].
The major technical problem limiting granulocyte transfusion efficacy has been the inability to obtain a sufficient dose of granulocytes to be effective in combating sepsis in neutropenic patients. Initial attempts to increase granulocyte yield utilized premedication with corticosteroids (dexamethasone 8 mg orally 12 h prior to the procedure, and 10 mg as a slow intravenous infusion immediately preceding the procedure) to induce marginalized granulocytes to enter the blood stream [79]. Even with this approach and modern apheresis techniques, the yield of granulocytes ranges from 2.5 to 5 × 1010 granulocytes for each donation [77–79]. This is still 1/100th of the estimated intrinsic response to sepsis. Functional studies have demonstrated normal antibacterial function of granulocytes collected using steroid premedication and HES sedimentation [80].
However, a resurgence of interest in studying the efficacy of granulocyte transfusions for treatment of systemic fungal infections has fostered a new approach to enhancing granulocyte yield from normal donors [81, 82]. Several reports from cancer centers have demonstrated that premedicating normal donors with granulocyte colony-stimulating factor (G-CSF) is effective in increasing the yield of granulocytes [83, 84]. Donors receive single or multiple doses of G-CSF and donor WBC counts reach as high as 50,000/μl, with mean granulocyte collection yields of 4 × 1010 (range, 1–14 × 1010) [83].
Recipients in these initial studies demonstrated sustained circulation of transfused granulocytes and no significant ill effects were noted in the donors [83, 84]. One report, evaluating the kinetics of the cells being administered prophylactically in bone marrow transplant patients, could not correlate the transfusion dose with the absolute neutrophil count achieved in the recipient, but noted significant and sustained increments in the recipients of G-CSF-stimulated granulocytes administered every other day in these patients [85]. In addition, several laboratories have evaluated granulocyte function in these collections, and have demonstrated that these cells have normal chemotaxis and phagocytosis, and may have enhanced enzymatic activity, demonstrated by chemiluminescence and superoxide formation [84, 86–88].
Reports in pediatric oncology, where there is a high granulocyte dose to body surface area (BSA) ratio, suggest clinical benefit [76, 89–91]. However, in the adult setting, reports are limited to cases and small series. Many investigators are calling for randomized studies to demonstrate clinical benefit from the use of these larger doses of granulocytes, obtained at substantial additional cost to the donor center and to donors. Current studies of granulocyte collection techniques are evaluating the relative efficacy in terms of collections by use of premedication with G-CSF alone, dexamethasone alone, or the combination, with reductions in doses of G-CSF [92–96] and donor tolerability of various premedication regimens [89, 94, 97].
The ability to premedicate donors and provide daily or every other day granulocytes is most efficiently achieved within the setting of a cancer center with apheresis capability and where donors are often highly motivated to provide products for a specific patient. Nevertheless, Price et al. have demonstrated the ability to provide maximally stimulated granulocytes in high yields utilizing community donors who are also highly motivated [98].
The ability to collect larger and potentially more therapeutically useful doses of granulocytes has stimulated increased interest in revisiting the issue of granulocyte storage. Historically, even short-term liquid storage of granulocytes has been extremely difficult due to the high metabolic activity of these cells. The number of morphologically normal granulocytes in standard storage conditions declines by 10–15 % per day during the first 2 days of storage. Studies have shown rapid exhaustion of glucose in the storage media [99], with measurable decreases in functional parameters such as microbial killing, chemotaxis, and the ability to circulate within the first 24 h of storage [100, 101]. Alterations in expression of adhesion molecules have also been described, which may contribute to these functional deficiencies [102]. One study evaluated the storage potential of granulocytes mobilized with G-CSF [103]. Although the hypothesis was that these cells might be less metabolically active, in fact the pH was less than 7.4 at the time of collection, and was less than 6.0 after 24 h of storage. To maintain storage pH, mobilized granulocyte concentrates required dilutions by 1 to 8 or 1 to 16, neither of which is practical [103].
New investigations of enhancing granulocyte storage are evaluating the addition of G-CSF to the storage medium [88, 91, 104], and some investigators have reported successful 24-h storage with this approach in conjunction with reduced temperature [104]. Further evaluation is needed, and no standard long-term storage practice currently exists.
Thus, current practice dictates that granulocytes be transfused as soon after collection as possible. Donors must be pretested so that the product can be transfused within hours of collection. Even for short-term storage (meaning hours), granulocytes should be placed in large-volume bags (1,000–2,000 ml) with a large volume of plasma to avoid loss of function. Storage should, however, be avoided if at all possible.
Therapeutic and Prophylactic Platelet Transfusions
A direct relationship exists between the platelet count and the bleeding time, which is used to assess platelet function in vivo [105].
The bleeding time will begin to lengthen as the platelet count falls to less than 100,000/μl, although clinically apparent bleeding problems may not develop until the platelet count is substantially lower (less than 20,000/μl). Early studies of patients with acute leukemia described the quantitative relationship between low platelet counts, duration of thrombocytopenia, and episodes of serious bleeding [2, 106] (see Chaps. 23 and 48). It was subsequently demonstrated that platelet transfusions could diminish the severity of bleeding in thrombocytopenic patients with ongoing hemorrhage. The effectiveness of therapeutic platelets transfusions is well documented [2, 106]. This observation led to the suggestion that platelets given prophylactically for low platelet counts might therefore help prevent bleeding. Since life-threatening hemorrhage can develop rapidly in the absence of prior signs of minor bleeding, particularly in patients with concurrent clinical problems, prophylactic platelet transfusion given for a platelet count of 10,000 to 20,000/μl is often the practice, rather than waiting for bleeding to occur [17]. This practice comes, however, with several caveats described later in this chapter.
It has become apparent that thrombocytopenic patients vary greatly in their likelihood to develop serious bleeding as well as in their ability to respond to random donor platelet transfusions. Therefore, assessment of the clinical setting should determine whether prophylactic platelet transfusions are indicated [17, 107–109].
Many patients with chronic hematologic disorders who maintain platelet counts of less than 20,000/μl or even less than 10,000/μl have no or minimal bleeding. In such patients, a low but stable platelet count is tolerated without transfusion unless other clinical factors are also present, such as infection or a bleeding site [17, 108, 109].
The other example is in chemotherapy-treated patients with solid tumors who are not treated at doses designed to achieve marrow aplasia and who have functional marrow reserve. A review of more than 1,200 such patients who experienced transient thrombocytopenia (less than 20,000/μl for a median of 2 days) showed no bleeding as a result of thrombocytopenia alone [110]. Those who did experience hemorrhage demonstrated the other clinical complications that have been shown to predispose to bleeding during thrombocytopenia, such as mucositis, a bleeding necrotic tumor, fever, and/or sepsis; bleeding frequently occurred at platelet counts above 20,000/μl [110].
Elting and a group from MD Anderson Cancer Center have also evaluated the risk of bleeding in patients with lymphoma and solid tumors who are being treated [111]. They have derived a bleeding risk index, with clinical factors similar to those in leukemia, which predicts for bleeding in this patient group, more than does a specific platelet count threshold [111].
By contrast, patients with acute leukemia and those undergoing highly intensive chemotherapy for other hematologic malignancies, whose treatment usually leads to marrow aplasia and in whom marrow regeneration may not be expected for a prolonged time period, even weeks, are usually treated prophylactically with platelet transfusions for expected prolonged severe thrombocytopenia. These patients frequently develop concurrent clinical conditions that add to the risk of thrombocytopenic bleeding: mucositis, fever, infection, and many of the other clinical complications listed in Table 56.3 [107, 112, 113]. Prophylactic platelet transfusions given to those patients who respond to transfusions with a posttransfusion increment are indicated in this setting [17, 107, 109, 113].
Table 56.3
Clinical conditions affecting platelet transfusion outcome
Clinical factora | Shorten 1-h count | Shorten 24-h count |
---|---|---|
Fever | No | ± |
Infection, controlled | No | ± |
Pre-platelet count <10,000 | No | + |
Bleeding: ooze | No | ± |
Daily RBC transfusion | ± | + |
Coagulopathy: DIC | ± | + |
Palpable splenomegalyb | ± | + |
Sepsisb | ± | + |
Hypersplenism | + | + |
Anti-HLA antibodies | + | + |
Amphotericin therapyb | + | + |
Bone marrow transplantationb | + | + |
As noted previously, the platelet count trigger to initiate prophylactic platelet transfusion has been a matter of debate, and the count plus the clinical setting seems the best approach. Three randomized trials have evaluated using a platelet count trigger of 10,000/μl vs. 20,000/μl in patients with acute leukemia [114–116]. In all three studies, there was no difference in major bleeding [114–116]; however, one found no difference in red blood cell transfusion requirements [114], and only one found that fewer platelet transfusions were administered [116].
A statistical analysis of the risk of bleeding in the Rebulla study determined that the risk factors for mild bleeding were increased body temperature and decreased platelet count [117]. Risk factors for clinically significant bleeding were grade 1 bleeding the previous day, low platelet count, and fever. Thus, as noted in many prior studies, clinical factors in the setting of profound thrombocytopenia markedly enhance the risk of bleeding (Table 56.3) [17, 113, 117]. Additional studies have demonstrated maintenance of endothelial integrity with platelet counts of ≥5,000/μl [118].
In addition to the conditions discussed previously, patients who demonstrate a rapidly falling platelet count appear to be predisposed to bleeding. Patients with hyperleukocytosis, particularly consisting of blasts, are also at greater risk and should receive platelet transfusions if they are thrombocytopenic. Finally, patients with coagulopathy, as is common in patients with acute promyelocytic leukemia (discussed in detail in Chap. 23), are at much greater risk of bleeding from their ongoing coagulopathy. They have been managed with heparin therapy, although this need may diminish in the future, and they often require frequent platelet transfusions, and coagulation factors [119–121]. Patients with liver dysfunction and decreased production of clotting factors are also at greater risk for bleeding, from the combined effect of both coagulopathy and thrombocytopenia. More frequent platelet transfusion may be necessary in such patients since they are very likely to have ongoing bleeding. Patients who are refractory to platelet transfusion in these acute settings pose a difficult dilemma, and this will be discussed subsequently. In general, if there is no platelet product that yields a posttransfusion increment, careful observation without transfusion (unless active bleeding is present) is the recommended course of action. This is stressful for staff and patient but is unusually manageable and is the safest course in the long run.
The increased availability of platelet transfusions has also permitted the safe performance of diagnostic and therapeutic procedures in thrombocytopenic patients. Platelets can be transfused just before or during invasive diagnostic procedures, such as bronchoscopy or endoscopy, and may permit the safe performance of brushings or biopsy. Therapeutic procedures such as the placement of permanent central intravenous lines (Hickman catheters), the drainage of infected paranasal sinuses, tooth extractions, or even emergency major surgery can be safely accomplished in thrombocytopenic patients with the use of platelet transfusions [122, 123]. Although no count threshold has been proven, we and others have observed that platelet counts of more than 50,000/μl provide a margin of safety for invasive procedures [122, 123]. It is very important to document a satisfactory posttransfusion count increment before initiating the procedure, to be confident that hemorrhage can be controlled during the procedure. It is equally important to maintain the platelet count at 50,000/μl or more for several days immediately following an invasive procedure, particularly after a biopsy or surgery.
To some degree, the debate regarding platelet count trigger for prophylactic platelet transfusion has been resolved with the randomized trials and the definitions of at risk clinical situations. However, the other debate among transfusion support providers is the dose of platelets necessary for a successful prophylactic transfusion. The goal is to provide sufficient patient support with the least exposure to risks of transfusion [infections, transfusion-related acute lung injury (TRALI)], and with the managed costs and preservation of platelet availability.
Recently two randomized, multicenter trials evaluating dose of platelets have been reported [124, 125]. The SToP trial was conducted at centers in Canada, the USA, and Europe, and compared standard-dose platelet transfusions to transfusions of 50 % less platelets [124]. Transfusions were a fixed dose per product transfused. In this study, there was more bleeding in the low dose arm, including more grade 4 bleeding, which led to the trial being stopped by the Data Safety and Monitoring Committee [124]. It may be that the fixed dose was part of the issue, although there was an upper weight limit, since in the other study described next, platelets were dosed based on BSA. In the PLADO study in the USA, there were three dose levels of platelets: 1.1 × 1011/m2, 2.2 × 1011/m2, and 4.4 × 1011/m2 [125]. The transfusion trigger was a platelet count of 10,000/μl and this was adhered to in 90–94 % of patient days and was similar in all three transfusion groups. The percentage of patients having grade 2 or higher bleeding was not significantly different, 71 %, 69 %, and 70 % in low, medium, and high groups, respectively, and the only death from bleeding was in the high-dose group (pulmonary hemorrhage). In this study, the percentage of patients with grade 2 or higher bleeding was significantly greater among those undergoing chemotherapy (73 %) or allogeneic stem cell transplant (SCT) (79 %) compared to those undergoing autologous or syngeneic SCT (57 %). However, platelet dose did not demonstrate an effect on bleeding in any of these groups. There was no difference in grade 4 bleeding among these different treatment groups [125]. Among these three dose groups, the low dose significantly reduced the quantity of platelets transfused, although with more transfusions given. Equivalent numbers of transfusions were given in the medium- and high-dose groups. This study suggests that a strategy of low-medium dose per BSA of platelets could reduce the number of platelets transfused, and preserve the resource that is increasingly being utilized, and maintain safe management of patients undergoing treatment for hematologic malignancies and SCT [125].
Assessment of Transfusion Response
The goal of therapeutic platelet transfusions is to stop active bleeding, which is usually easily assessed clinically. The response to prophylactic platelet transfusions is more difficult to evaluate. Bleeding times, although accurately reflecting platelet function in vivo, are not usually done in granulocytopenic patients because of the risk of infection and because achievement of a platelet count of 100,000/μl is required to normalize the bleeding time [105]. Therefore, response to transfusion is monitored by observing the increase in platelet count shortly after transfusion (i.e., platelet recovery). The increment achieved depends on the size of the recipient and on the number of platelets given. The dose of platelets usually given is approximately 1 U/10 kg body weight for a child and 6–10 U/transfusion for an adult.
The survival (duration of time circulating) of transfused platelets can be followed by obtaining daily platelet counts following a platelet transfusion. In a patient who is truly aplastic and not producing platelets, the half-life of the transfused platelets can be calculated. Platelet survival will be shortened by a number of clinical factors, which, in turn, increase the requirement for platelets. These factors include active bleeding, fever, infection, coagulopathy, and hepatosplenomegaly. Platelet survival is also shortened by the development of alloimmunization to histocompatibility antigens, producing immunologic destruction of the transfused platelets. To differentiate alloimmunization from other forms of platelet destruction, platelet recovery after a transfusion (which is diminished by alloimmunization) may be helpful in stable patients. The posttransfusion recovery is assessed by calculating the corrected count increment:


For example, an improvement in count from 20,000 to 60,000/μl after 4 × 1011 platelets when given to a 2.0 m2 individual produces a corrected count increment of 20,000/μl.
After the transfusion of fresh or properly stored random donor platelets to a clinically stable adult patient of average size, the absolute count increment at 1 h should be 6,000–8,000/μl/U of platelet transfused, with a CCI of 10,000 to 20,000/μl. The hallmark of the clinical diagnosis of alloimmunization is a failure to achieve an adequate platelet count increment at 1 h posttransfusion [112] (for practical purposes, a 10-min posttransfusion count may be equally helpful) [126]. Other clinical factors may contribute to the rapid utilization of platelets, however, and may reduce the posttransfusion count (Table 56.3).
Bishop et al. [113, 127] performed a multivariate analysis of clinical factors influencing the efficacy of pooled platelet transfusion as determined by the 1-h posttransfusion platelet CCI. This study identified six major clinical factors that affected 1-h posttransfusion platelet counts. Whereas splenectomy resulted in improved increments, several factors had a negative effect on transfusion outcome: palpable splenomegaly, bone marrow transplantation, disseminated intravascular coagulation, amphotericin B therapy (which may reflect the degree of illness of a given patient, who may have fever and infection), and anti-HLA antigen-directed antibodies [113, 127]. This group performed a similar evaluation of the effect of clinical factors on the 20-h platelet count (platelet survival) and again found that the clinical situation had a significant impact [127]. The combination of all these factors makes confirmation of the diagnosis of alloimmunization difficult in rather complex clinical cases, and refractoriness to transfusion without development of antibodies appears to reflect the multitude of clinical factors impinging on posttransfusion platelet counts [113, 127–129].
In addition, Hanson and Slichter [130] demonstrated a reduced platelet life span in severely thrombocytopenic patients, presumably due to utilization of platelets for support of vascular integrity. In this study using autologous platelets, platelet life span was only modestly shortened when patients with platelet counts in the 50,000/μl to 100,000/μl range (7.0 ± 1.5 days, 9.6 ± 0.6 days, respectively) were transfused, but was markedly shortened in severely thrombocytopenic patients (less than 50,000/μl) (5.1 ± 1.9 days) [130]. Recovery of autologous platelets was also diminished in patients with platelet counts less than 50,000/μl (50 ± 22 %) compared with that in patients who had counts more than 50,000/μl (74 ± 15 %).
The controversy regarding platelet transfusion mismatched for ABO continues to be discussed. Early studies have suggested decreased platelet survival with ABO-mismatched platelet transfusion [131]. A paired clinical study comparing ABO-matched and ABO-mismatched random donor platelet transfusions has demonstrated a detrimental effect of ABO mismatch on platelet transfusion outcome [131]. Although the initial platelet transfusion may not be affected by ABH incompatibility, results of subsequent mismatched transfusions deteriorated, perhaps because of an increase in ABH antibody titers [131].
Nevertheless, platelets can successfully be transfused across ABH groups. Clinical complications or transfusion reactions are only rarely associated with ABH-mismatched platelet transfusions. A recent systematic review of the literature on transfusion of ABO-identical vs. non-identical platelets evaluated both transfusion and patient outcome [132]. There was a consistently higher platelet increment with the use of ABO-identical platelet transfusions. However, in this retrospective review of the literature, there was no consistent clinical impact on survival, bleeding, or transfusion reaction reported, although these outcomes were not consistently reported in all studies [132].
Classic teaching states that ABH antigens are not intrinsic to platelet membranes, but may be absorbed from plasma [133]. However, more sensitive techniques have demonstrated the presence of intrinsic H substance on platelet membranes with expression of ABH antigens corresponding to the RBC phenotype, suggesting the presence of intrinsic and extrinsic antigenic substance residing on platelets [134, 135].
Thus, in patients who become refractory and who have received ABH-mismatched platelets, better posttransfusion increments may be obtained with a trial of ABH-compatible platelet transfusions.
Alloimmunization
Alloimmunization, the development of antibodies (primarily to histocompatibility [HLA] antigens), often leads to immune-mediated refractoriness to platelet transfusions. Prior to the routine use of leukodepleted blood products, alloimmunization was a major factor in the development of refractoriness to platelet transfusions and can lead to hemorrhagic deaths. Once antibodies develop, they can persist throughout the course of treatment cycles, making transfusion support very difficult.
Studies conducted in clinically stable patients have been able to correlate the presence of lymphocytotoxic antibodies directed against HLA antigens with posttransfusion CCI [112, 136, 137] (Table 56.4). The development of lymphocytotoxic antibodies frequently correlates with the loss of response to random donor platelets and indicates the necessity of providing HLA-matched platelet transfusions from previously HLA-typed donors [112, 137].
Table 56.4
Lymphocytotoxic antibody (LCTAb) levels and response to random donor platelet transfusionsa (corrected count increment dependent on level of LCTAb)
CCI at time after transfusionb (median, range) | LCTAb negative (0–10 %) | LCTAb intermediate (11–20 %) | LCTAb positive (21–100 %) |
---|---|---|---|
1 h | 16.1 (2.1–34) | 13.3 (3.5–28.9) | 5.6 (0–27.8) |
24 h | 12.0 (1.3–31) | 7.4 (1.5–19.8) | 2.6 (0–17.8) |
Chow et al. have confirmed this correlation between development of lymphocytotoxic antibodies and loss of response to random donor platelets [138], and then showed that providing HLA-matched transfusions, particularly in a more homogeneous population, such as the Chinese, led to a successful transfusion outcome [138].
As noted previously, refractoriness to platelet transfusion is multifactorial, involving both immune and nonimmune clinical features. Further analysis of the Trial to Reduce Alloimmunization to Platelets (TRAP) study demonstrated both platelet and patient factors leading to refractoriness (Table 56.5) [129]. In utilizing platelet transfusion increment rather than CCI, the rate of refractoriness was 27 %. However, in this section we will discuss the management of poor transfusion outcome due specifically to alloimmune factors and demonstrate the process of providing matched platelet transfusions.
Table 56.5
Factors affecting posttransfusion platelet increments and refractoriness
Patient factors |
Reduced response and/or refractory to transfusions |
More than two prior pregnancies |
Presence of lymphocytotoxic antibody (LCTAb) |
Male gender |
Bleeding |
Fever, infection |
Increased body surface area/weight |
Increasing number of platelet transfusions |
Heparin therapy |
Reduced platelet increment, additional factors |
Splenomegaly |
Disseminated intravascular coagulopathy |
Amphotericin B therapy |
Improved platelet increment response |
Splenectomy |
Increased patient age |
Improved response to refractoriness |
Increased platelet dose |
Filtered apheresis platelets |
Platelet factors |
Improved response |
ABO compatible |
Storage <48 h |
Large doses of platelets |
Decreased response |
UVB or Gamma irradiationa |
In most cases of alloimmune platelet refractoriness, successful transfusions can be accomplished with the use of platelets matched at the HLA-A and -B loci, using family members as well as nonrelated matched donors [139]. Care must be used in selecting family transfusion donors if an allogeneic transplant may be a later consideration, but in nontransplant candidates, family donors are often highly motivated and available and may provide an excellent source of matched platelets. In general, donors who are either HLA-identical with the recipient or who do not possess any HLA-mismatched antigens are preferred, because the recipient usually has multispecific lymphocytotoxic antibodies directed against multiple additional HLA antigenic epitopes.
Fortunately, within the HLA system, several groups of HLA antigens share antigenic characteristics and are considered serologically cross-reactive. Thus, the number of available donors can be increased by selectively mismatching for certain antigens that are cross-reactive with those of the recipient, and thus are functionally compatible [140]. In addition, certain HLA antigens, such as HLA-B12, HLA-B44, and HLA-B45, are sometimes expressed less strongly on platelets than on lymphocytes [141].
Since HLA typing is performed with lymphocytes, a mismatch at HLA-B12, HLA-B44, or HLA-B45 may still yield a good platelet transfusion response [142]. This technique of selective mismatching makes transfusion support of some alloimmunized patients considerably more feasible.
Figure 56.2 graphically demonstrates the results of this transfusion approach for an alloimmunized patient unresponsive to random donor platelets (transfusion # 1), using donors with varying degrees of HLA matching. The patient responded well to a perfectly matched transfusion (#3) and to two transfusion mismatched at a cross-reactive (HLA-B7) antigen (#2, #4), with good CCIs at 1 and 24 h after transfusion. By contrast, the lack of response to a transfusion mismatched at a non-cross-reactive antigen (#5) is also shown. Two additional transfusions (#6, #7) that were mismatched at HLA-B44 and HLA-B12 (with variable expression on platelets) also produced good CCIs. These cross-reactive antigen groups reflect differences in public and private epitopes and the use of this strategy is described as utilizing cross-reactive groups (CREGs) [143].
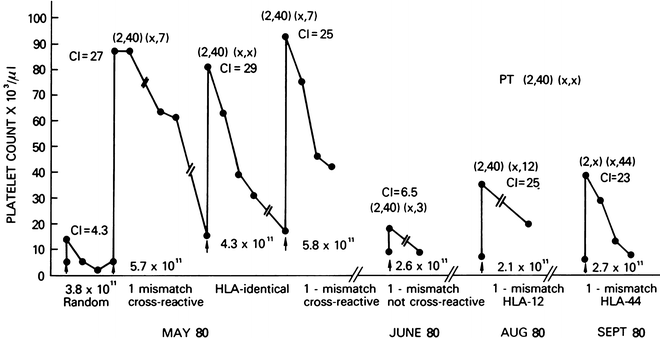
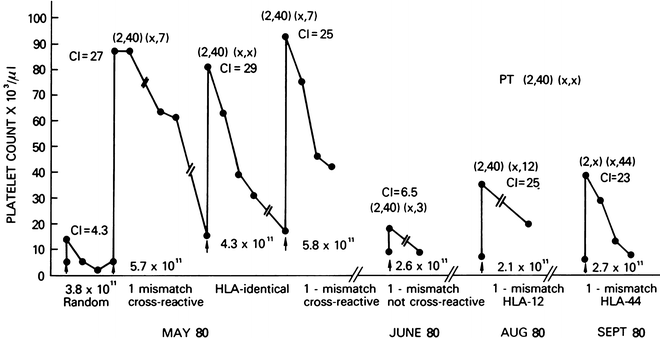
Fig. 56.2
Clinical course of this alloimmunized patient demonstrates the value of selectively mismatched platelets when the antigens are cross-reactive with recipient antigens (transfusions #2 and 4). Platelets mismatched at HLA-44 were also clinically effective (transfusion #7). Parentheses, HLA phenotypes; CI corrected increment
If appropriately matched donors cannot be located for refractory alloimmunized patients, it is inadvisable to continue to administer random donor platelets prophylactically because the risks and discomforts of such transfusions outweigh the benefits. However, significant bleeding in these patients can sometimes be managed by infusions of massive amounts of unmatched platelets [129], although heavily sensitized patients even then do not obtain count increments and continue to bleed. The possible mechanisms leading to response with this approach may include the accidental inclusion of partially matched platelets in the random pooled platelets or a temporary reduction in antibody titer by the large amount of infused antigen, enabling some of the transfused platelets to survive.
Finally, in parallel with studies in autoimmune thrombocytopenia, attempts have been made to abrogate alloimmunization by blocking the reticuloendothelial system of the spleen and liver with high doses of intravenous gamma globulin. Although this approach has demonstrated benefit in autoimmune thrombocytopenia [144], it has not proved beneficial in carefully controlled studies in patients with alloimmunization affecting transfusion outcome [145, 146]. Zeigler et al. [147] used gamma globulin with partially HLA-matched platelets in patients who had become refractory; they reported some improvement in transfusion outcome, although the response was not consistent. Kickler et al. [148] evaluated the outcome of transfusion from matched or partially matched donors, to whom patients no longer responded. Half of the patients received intravenous gamma globulin in addition to platelets from this donor, and had a significantly improved 1-h posttransfusion count compared with those patients who did not receive gamma globulin. However, the 24-h posttransfusion platelet survival was not improved [148]. Thus, the potential value of intravenous gamma globulin in the setting of alloimmunization appears to be quite limited and is not recommended in general, particularly in view of its high cost and limited benefit.
It also appears that some patients lose their allo-antibodies over time [149, 150]. In certain cases, no recent antigenic challenge has occurred, which would explain the decline in lymphocytotoxic antibody levels, and a rapid, anamnestic response to rechallenge is often seen. However, in a study of 234 patients who had developed lymphocytotoxic antibodies at some time in their clinical course, 70 (30 %) had significant declines in antibody level [150]. Among 35 evaluable patients, previously alloimmunized, who then lost their antibodies and were rechallenged with random donor platelets, 34 patients when rechallenged responded with good transfusion outcomes to random platelets for 2 weeks to 36 months [150]. Twenty-one patients failed to regenerate lymphocytotoxic antibodies despite repeated transfusions [150].
It is apparent that the transfusion support of alloimmunized patients is complex and time consuming, and can require substantial resources. This includes access to laboratory facilities for HLA typing of both the patient and numerous donors, computer facilities to process the donor list, and substantial donor recruitment efforts. In addition, not all patients who appear to be alloimmunized respond to transfusions chosen by lymphocytotoxicity-based HLA matching, suggesting the presence of complex immunologic factors [137].
Spurred by increased organ transplantation, newer technology has been developed to enhance and refine detection of anti-HLA antibodies, including the addition of antihuman globulin [151] and commercially available enzyme-linked immunosorbent assays (ELISAs) or mini-flow platform binding (Luminex Corp) [151–154].
There is also increased interest in developing crossmatching tests using the platelet as the target cell. The most practical approach is to use stored donor platelets from potential donors, to be cross-matched with recipient serum prior to transfusion [155, 156]. Moroff et al. [155] evaluated comparative transfusion data in patients known to be refractory to random donor platelets, choosing donors by HLA matching or by platelet cross-matching using three different methods. They found comparable percentages of successful transfusions using either HLA-selected or cross-matched-compatible donor selection. They also found that platelet antibody tests did not always coincide with the outcome of a specific transfusion. However, transfusion outcomes in those selected by HLA type that restricted transfusions to A and BU matches were superior to selection based on cross-matching alone and to donor selection based on any lower grade of HLA mismatching, including cross-reactivity [155].
In another early study, both HLA-compatible and cross-match-compatible methods were effective in providing single-donor platelets; these were independently important selection methodologies [156]. New technology for HLA matching is being re-evaluated, and includes DNA techniques for defining HLA types. Additionally, a computerized algorithm, called HLAmatchmaker, is being utilized to predict HLA compatibility by identifying amino acid triplets in antibody accessible regions of HLA proteins [28]. This technique began in the organ transplantation field, and is now being evaluated in platelet transfusion therapy [157, 158].
New technology in cross-matching is now frequently utilizing an assay known as Capture P, which is a commercially available SPRACA assay [159]. While detecting both anti-HLA and antiplatelet antibodies, this assay also detects ABO isoantibodies [160].
In another methodology, Petz et al. utilized an antihuman microlymphocytotoxicity test to determine the specificity of the recipient’s HLA antibodies. In their study, the outcome of transfusion of platelets chosen by the antibody specificity prediction method (ASP) [161] was compared to the outcome of platelet transfusions chosen by HLA matching and/or by cross-matching. The results were comparable. The potential advantage of this method is that more donors might be identified than through HLA typing, and the frequency of testing would be less than with cross-matching. Further evaluation of this method is ongoing.
All methods of donor selection have their proponents in the blood bank community, often based on technology available, donor availability, and cost in time and expense [153, 162].
Fortunately, the occurrence of alloimmunization is sporatic and studies have shown that the development of lymphocytotoxic antibodies is less than 50 % of patients with hematologic or solid malignancies [149, 150, 163, 164]. These data are derived from an era prior to significant leukodepletion of blood products so that the incidence is now likely to be much less, as will be discussed subsequently. Two separate studies have evaluated the incidence of alloimmunization in large groups of patients with leukemia [163] or other malignant diseases [164]. In both studies, large groups of patients were followed over a long period during treatment (2–6 months or more); no relationship was found between the number of platelet transfusions administered during the early part of the treatment phase and subsequent alloimmunization, suggesting that a minimum number of platelets is sufficient to immunize; thus, reduction of transfusion numbers will not prevent alloimmunization.
Specifically, in the study of patients with acute myeloid leukemia (AML) by Dutcher et al. [163], nonalloimmunized patients were evaluated during remission induction therapy (the first 8 weeks of treatment) for the development of alloimunization, assessed by response to random donor platelet transfusion and the development of lymphocytotoxic antibodies. All patients received random donor platelet transfusion (mean, seven transfusions; range, two to more than ten), given prophylactically and therapeutically. Of the patients not previously alloimmunized, 40 % became alloimmunized, some after as few as two transfusions (Fig. 56.3). By contrast, 60 % of the patients did not become alloimmunized, even after multiple platelet transfusions. Except for previous pregnancies and blood transfusions, no predisposing factors were predictive of the development of alloimmunization. Since a minimum of two platelet transfusions (and usually more) is needed to make it through the remission induction phase of AML treatment, no ratio exists for restricting the number of platelet transfusions specifically to prevent alloimmunization [163]. No dose–response phenomenon occurs at a clinically manageable level, and the development of alloimmunization appears to depend on the immunologic responsiveness of the recipient. Therefore, newer measures, specifically leukodepletion, have been investigated as a means of preventing alloimmunization, and this will be discussed in detail subsequently.
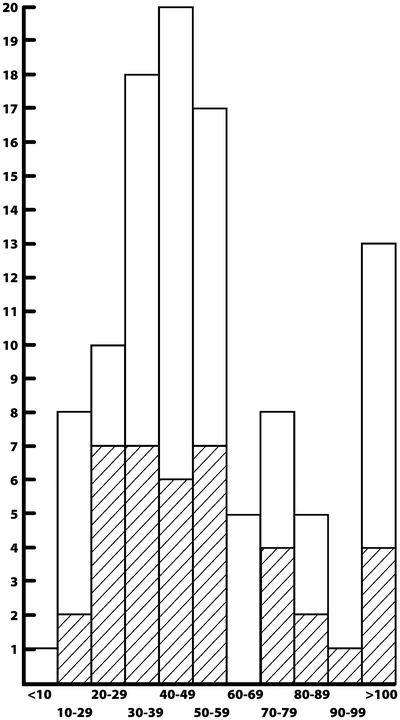
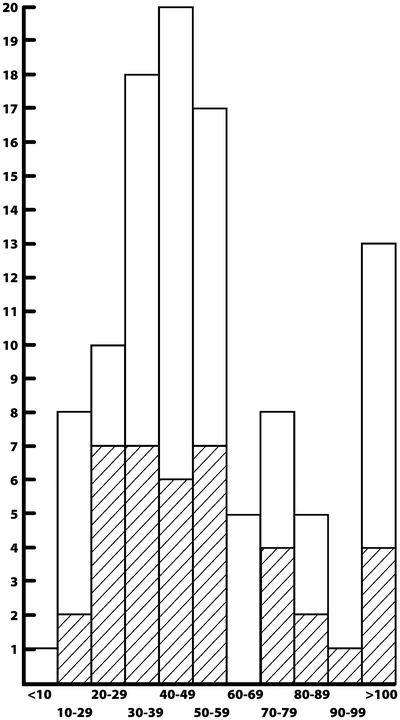
Fig. 56.3
Number of units of platelets administered per patient during induction therapy in patients with acute leukemia. There is no relationship between the number of units given and the rate of alloimmunization. Cross-matching: patients who became alloimmunized (Reproduced with permission from Dutcher et al. [163])
The long-term follow-up evaluation of those patients who survived induction therapy for their leukemia was also of interest. Patients who became alloimmunized after induction therapy remained alloimmunized during subsequent periods of treatment and transfusion, whereas almost all patients who did not become immunized after induction therapy never became immunized, and continued to respond to random donor platelets [149]. The alloimmunization status of patients during subsequent treatment courses could be predicted in more than 90 % of patients on the basis of their alloimmunization status after the induction treatment period. Patients who remain alloimmunized are identified as a group requiring continued specialized platelet transfusion support during subsequent antileukemic treatment. However, patients who never become alloimmunized are more easily given transfusion support and may therefore be better able to receive more intensive postremission therapy. Table 56.6 provides an algorithm for managing platelet transfusion support throughout the course of a patient’s treatment for leukemia.
Table 56.6
Algorithm of platelet transfusion management
I.Preparation of a new leukemia or other patient requiring multiple transfusions |
A.Obtain HLA typing from patient and family and maintain record |
B.Transfusion history: prior pregnancies, transfusions, viral infections |
II.Transfusion products |
A.RBCs: filtered or leukodepleted at blood center |
B.Platelets: Leukodepleted, random donor |
1.Pooled single units |
2.Apheresis |
C.Irradiated if potential transplant candidate or severely immunosuppressed |
III.Reactions to platelets or poor 24-h increment |
A.Obtain 1 h or 10 min posttransfusion count |
B.Assess other clinical factors that may contribute to refractoriness |
C.Assess storage duration of platelets prior to transfusion |
D.Evaluate ABH compatibility |
E.Assess for presence of lymphocytotoxic antibodies |
F.Reaction, but adequate posttransfusion increment |
1.Initiate leuko-poor or filtered platelets if not done previously |
2.Continue to monitor early posttransfusion platelet counts |
G.Poor immediate posttransfusion increment |
1.Nonmatched but fresh single-donor product—get early posttransfusion count |
2.Family donor—possibly more compatible—get early posttransfusion count |
3.Begin HLA-matched platelet transfusion—get early posttransfusion count |
4.Consider cross-matched, ASP-selected platelets |
IV.Subsequent courses of treatment |
A.Reevaluate transfusion history to determine previous response to platelet transfusions |
B.Obtain lymphocytotoxic antibody (LCTAb) screening level |
C.If alloimmunized, refractory, or with a history of reactions or with high-titer LCTAb |
1.Initiate autologous platelet cryopreservation |
2.Be prepared to provide HLA-compatible or cross-matched platelet products |
3.Monitor all transfusions with early posttransfusion counts |
D.If no history of alloimmunization or refractoriness |
1.Begin with pooled single units or random single-donor apheresis platelets |
2.Monitor early post-transfusion counts |
a.May develop anamnestic antibody response |
b.Likely to continue to respond to random donor platelets |
Prevention of Alloimmunization
The contaminating lymphocytes in earlier preparations of platelet transfusions served as the major antigenic stimulus or antigen-presenting component leading to alloimmunization to HLA antigens [165]. Animal studies document this, in that in murine studies, repeated administration of pure preparations of platelet suspensions was incapable of inducing an antibody response, but when the preparation also contained lymphocytes, an antibody response was induced [165]. These observations have led to human clinical trials attempting to modify the leukocyte exposure and directed to the earlier proposed mechanism of antigen exposure.
Lymphocytes carry class II histocompatibility antigens that are necessary for invoking a recipient immune response against class I HLA antigens. Although class II antigens are not found on platelets, the presence of lymphocytes in the transfusion leads to activation of an immune response that is subsequently directed toward both lymphocytes and platelets (which carry class I antigens) and thus produces the development of alloimmunization and transfusion refractoriness [165, 166].
New technology and enhanced leukodepletion efficiency have led to an evaluation of the degree of leukocyte depletion and thus antigen dose necessary for the development of alloimmunization. It appears that different levels of leukocyte depletion yield specific results. For example, a reduction to less than 107 leukocytes/transfusion will reduce the rate of transfusion reactions. However, it requires a reduction to less than 106 leukocytes to prevent transmission of cytomegalovirus (CMV) and to reduce the development of alloimmunization [167–169].
The TRAP study has demonstrated that this level of leukocyte depletion can be accomplished utilizing both modern apheresis techniques and modern filtration techniques, most with polyester, leukoadherent filters (all provide reduction of greater than 3 logs, to <107 leukocytes) [15, 29, 167–169]. These data are supported by other smaller studies that in fact provided the stimulus to engage in this randomized trial to test the hypothesis of leukodepletion and the prevention of alloimmunization [15, 29, 170–172]. In addition, in the TRAP study, a method of lymphocyte inactivation, utilizing ultraviolet B (UVB) irradiation, was administered to the transfusion products, to inactivate lymphocytes and antigen-presenting cells, such that they became incapable of generating an alloresponse [169, 173–175]. In animal studies, data demonstrated the ability to prevent alloimmunization and to perhaps induce immune tolerance using this technique [173, 174]. Initial studies in humans demonstrated the functional integrity of platelets subjected to UVB irradiation as evaluated by posttransfusion platelet count increments [173, 175], although storage time was somewhat diminished [176]. Therefore, this technique was felt to be appropriate for study in the TRAP trial, since it was not known at the initiation of the trial whether the current techniques would produce sufficient leukodepletion to actually prevent alloimmunization.
Subsequently, the TRAP study has demonstrated that this level of leukodepletion or leukocyte inactivation by UVB does in fact lead to a major reduction in the rate of alloimmunization among patients with leukemia who are transfused multiple units of platelets and RBCs [169]. All three approaches (filtration, apheresis, and UVB inactivation) significantly reduced the rate of alloimmunization compared to unmanipulated platelet products, and they were all equally effective. Among the subjects who received nonmanipulated platelets, the rate of development of lymphocytotoxic antibodies was 45 % (somewhat lower than that in many previous reports), but the rate of development of lymphocytotoxic antibodies among the other three treatment groups was 17–21 %. The rate of alloimmunization leading to refractoriness to platelet transfusion was 13 % among those who received standard, nonmanipulated, pooled random donor platelets (again, lower than that in many reports) compared to 3–4 % in the patients in the other three groups [169].
The overall rate of refractoriness was 27 %. Thus, the current platelet transfusion product recommended for patients expected to receive multiple platelet transfusions, such as those being treated for leukemia or expected to undergo SCT, is to provide leukocyte depleted platelets. Irradiated products are recommended for those who will undergo SCT and those with severe immunodeficiency, such as Hodgkin’s disease. This will be discussed further subsequently.
Hazards of Platelet Transfusion
Platelet transfusions are usually very safe, and the benefits outweigh the potential risks. Nevertheless, certain hazards of platelet transfusion therapy should be considered. Transfusion reactions can occur with platelet transfusions, usually attributable to sensitization [31, 32, 177, 178]. Transfusion reactions occur most commonly during or shortly after infusion (although they may be delayed if the patient is premedicated with acetaminophen) and are usually mild, consisting of a sensation of chilliness (rarely shaking chills) or fever, with or without urticaria. Urticaria is most often associated with plasma-related components. Reactions due to leukoagglutinins (white cell antigen–antibody reactions) are febrile and nonhemolytic. Leukocyte-poor platelet products have been shown to reduce the incidence of platelet transfusion reactions due to leukocyte-specific antigens and even to anti-HLA alloimmunization [178]. Stored platelets, if not prefiltered, may accumulate cytokines released from leukocytes during the storage process, such as interleukin-6 (IL-6) among others. These may lead to a febrile reaction upon transfusion [31, 178, 179]. The presence of IL-6 in the transfusion may also induce what appears to be an allergic reaction with urticaria [179].
Although with modern platelet preparation, red cell contamination is reduced, there is still considerable plasma, which may contain antibodies to ABH blood types. Therefore, ABH-mismatched platelet transfusion may rarely be associated with a hemolytic reaction if the donor has a high-titer hemagglutinin [180–182]. These have been reported over the years as case reports, but a recent report suggests that this type of reaction may be underrecognized in a population receiving numerous blood products [182].
Bacterial contamination of platelets is a rare, but documented cause of platelet transfusion reaction from stored platelets. This must be considered in severe reactions, and the transfusion bag and patient should be cultured [183].
Because platelets must be stored at room temperature, the risk of bacterial growth limits the duration of storage [184, 185]. New methods of testing for bacterial contamination are being applied pre-transfusion [186, 187].
Circulatory congestion is a common, preventable problem in very ill patients receiving multiple infusions. This develops not only from the individual transfusions being administered, but also from the additive effects of administration of all blood products, multiple antibiotics, and other intravenous medications. If necessary, the volume of plasma in a platelet transfusion can be decreased immediately before infusion, using centrifugation and resuspension in a smaller volume of plasma. This is routinely done for transfusions to children.
TRALI may be an unrecognized cause of non-cardiogenic pulmonary edema following platelet transfusion. This is a reaction from antibodies in the plasma of the transfusion product. In the past 5 years, a TRALI-risk reduction policy for donor screening has been implemented in a number of countries and has been widely accepted [188]. Female platelet pheresis donors with history of prior pregnancies trigger HLA-Ab testing and those who are positive are redirected to whole blood donation.
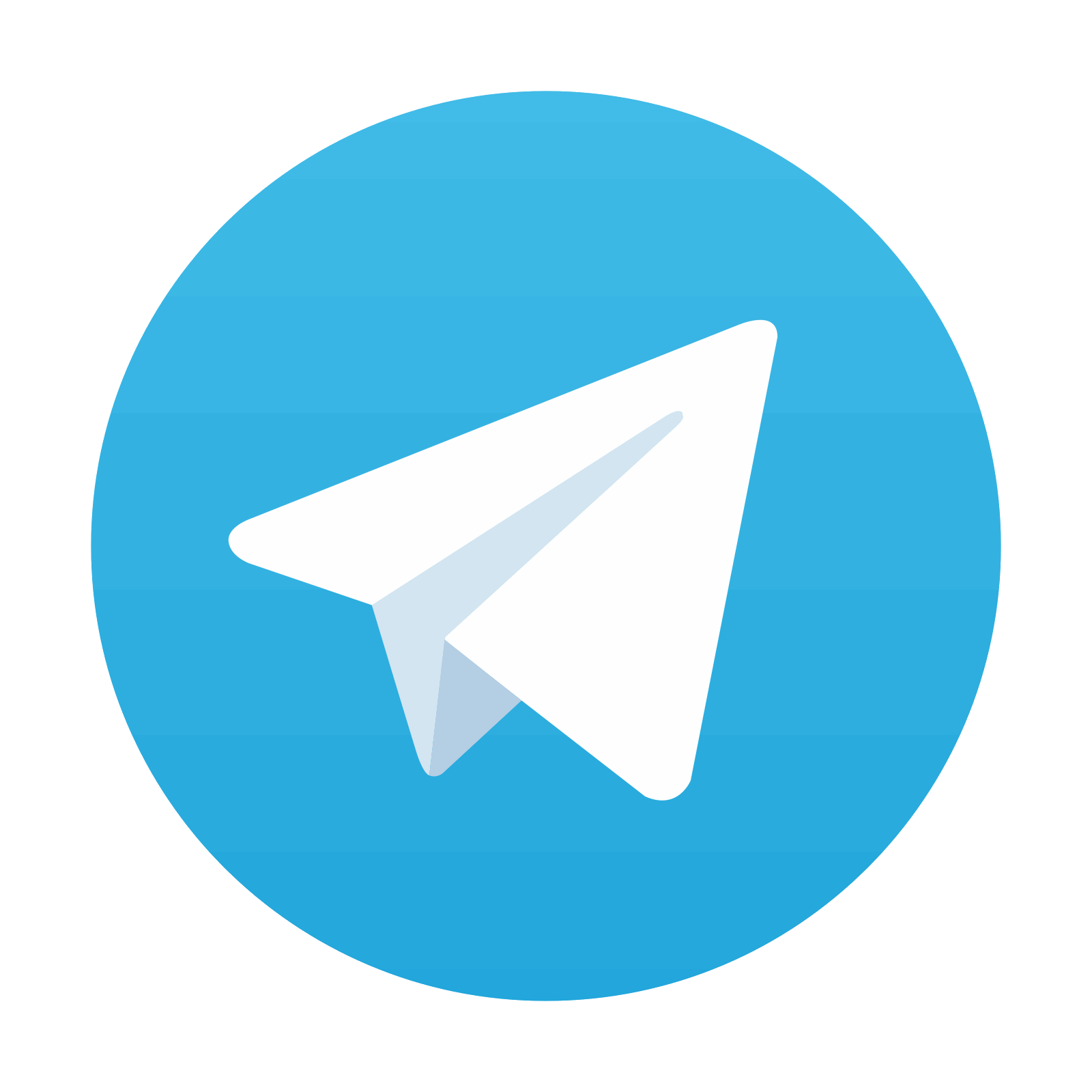
Stay updated, free articles. Join our Telegram channel

Full access? Get Clinical Tree
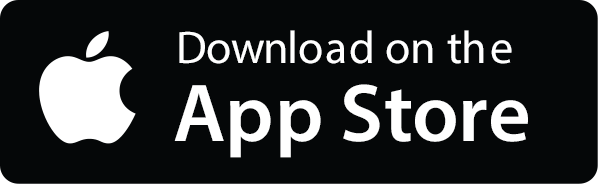
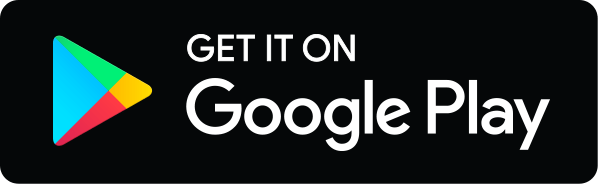