Fig. 32.1
a Midline sagittal section of brain MRI depicting the gross anatomical relations of anterior pituitary in a 2.5 year old infant. b Midline sagittal section of brain MRI in an adolescent male with Rathke’s pouch cyst abutting the optic chiasm. c Coronal section of brain MRI at the level of pituitary. d Midline sagittal section of brain MRI in an adolescent female with enlarged pituitary gland. The gland appears to be moulding the optic chiasm. Follow-up MRI after 4 months showed resolution of the enlargement. This type of enlargement is not uncommon during adolescence. Sphenoid sinus is fully developed in this age rendering transphenoidal approach easier. AP anterior pituitary; CC corpus callosum; ICA internal carotid artery; M Medulla oblongata; MB midbrain; NP nasopharynx; OC optic chiasm; ON optic nerve; P pons; PG pituitary gland; PP posterior pituitary; PS pituitary stalk; S sphenoid sinus; T thalamus
The pituitary gland is covered superiorly and largely separated from the brain by a circular fold of dura mater known as the diaphragmatic sella. The diaphragmatic sella has a central opening, or aperture, for the infundibular stalk, which connects the pituitary to the brain. Anteriorly, the diaphragmatic sella separates the anterior part of the pituitary from the optic chiasm, which lies roughly 10 mm above the diaphragmatic sella [3]. The proximity of the pituitary to the optic chiasm accounts for the visual field loss that is often associated with large pituitary tumours.
When viewed from above the diaphragmatic sella may be concave, flat or convex and ranges in width from 5 to 11 mm in adults. The aperture of the sella varies in size and, if wide enough, can result in herniation of the arachnoid mater, or membrane, into the sella turcica. This herniation of the arachnoid membrane into the sella turcica enlarges the chiasmatic cistern, the subarachnoid space below the optic chiasm, and flattens the pituitary. This condition is known as the empty sella [4]. Herniation of the arachnoid membrane into the sella turcica increases the risk of injury to the membrane during pituitary surgery and subsequent cerebrospinal fluid (CSF) leak [2].
The walls of the sella turcica are formed by the folds of dura mater, which also form the medial walls of the cavernous sinuses, the space lateral to pituitary filled with thin walled veins [2]. The oculomotor, trochlear and the first two branches of the trigeminal nerve run in the lateral walls of the cavernous sinuses and the abducens nerve, a portion of the internal carotid artery and the sympathetic plexus are contained within the sinuses [2]. The pituitary gland is separated inferiorly from the floor of the sella turcica by a venous sinus that communicates with the circular sinus, the veins in the spaces anterior and posterior to the sella turcica that connect the two lateral cavernous sinuses [1].
Anterior Pituitary
The anterior pituitary consists of epithelial cells that derive from the oral ectoderm [5]. It consists of the pars anterior (or pars distalis), which is the anterior or distal part of the anterior pituitary, the pars intermedia which is the intermediate part of the pituitary between the anterior and posterior pituitary, and pars tuberalis which surrounds the infundibular stem of the posterior pituitary. The pars anterior and intermedia are separated by a cleft, which is a remnant of Rathke’s pouch from which the anterior pituitary develops (see section on Embryology). Pathologic enlargement of the cleft may lead to a cyst known as a Rathke’s cleft cyst (Fig. 32.1b). In humans, in contrast to the mouse, the pars intermedia largely disappears during embryogenesis and is rudimentary and ill-defined in the adult pituitary [6]. Melanocyte-stimulating hormone (MSH) is traditionally thought to be generated in the pars intermedia; however, it may actually be produced in the pars anterior [7].
Histologically distinct cells found in the anterior pituitary include chromophil cells, chromophobe cells, and folliculostellate cells. Folliculostellate cells do not produce hormones while the chromophil and chromophobe cells produce hormones. The hormone producing cells are named for the hormone(s) they produce. The anterior pituitary produces six different peptide hormones: (1) growth hormone (GH) or somatotrophin, (2) prolactin (PRL) or mammotrophin, (3) adrenocorticotropic hormone (ACTH) or corticotrophin, (4) thyroid-stimulating hormone (TSH) or thyrotrophin, (5) follicle-stimulating hormone (FSH), and (6) luteinising hormone (LH).
The chromophobe cells comprise the majority of the cells of the anterior pituitary making up about 50% of the epithelial cells. These cells are small and do not react to routine staining. This population comprises different cell types such as degranulated secretory cells and stem cells.
The chromophil cells are either acidophils or basophils. Acidophils, also known as α-cells, include somatotrophs (producing GH), mammotrophs or lactotrophs (producing prolactin), and somatomammotrophs (producing both GH and prolactin). Basophils, also known as β-cells, include corticotrophs (producing ACTH), thyrotrophs (producing TSH), and gonadotrophs (producing LH and FSH). Pituitary tumours are often characterised as basophil or acidophil depending upon the dominant cell type. The somatotrophs are the largest and most abundant making up approximately 50% of the chromophil cells in the anterior pituitary. The thyrotroph cells make up 6–10% of the chromophil cells [8]. Corticotrophs in humans are distinguished by 300 nm secretory granules [8], and account for 10% of the chromophil cells. Gonadotrophs make up another 10–15% of the chromophil cells. They are located throughout the anterior pituitary, often in close proximity to mammotrophs [6]. The majority of gonadotroph cells have granules of 200 nm but often larger—500 nm—granules are seen. All cells that produce glycoproteins (thyrotrophs and gonadotrophs) stain PAS (periodic acid-Schiff) positive [8].
The folliculostellate cells constitute the supporting and trophic network of the hormone producing cells and contain peptides with growth factor or cytokine activity. Basic fibroblast growth factor is produced in folliculostellate cells and is implicated in the control of the production of pituitary hormones in a paracrine manner.
Posterior Pituitary
The posterior pituitary arises from the diencephalon (the posterior forebrain located between the midbrain and the cerebrum) and consists primarily of axons from supraoptic and paraventricular nuclei of the hypothalamus. These neurons secrete vasopressin (or antidiuretic hormone, ADH) and oxytocin. The posterior pituitary includes the median eminence at the base of the infundibular stalk, the infundibular stalk (or infundibulum, or stalk) and the pars posterior (or posterior lobe, or neural lobe). The infundibulum of the pituitary is a conical process originating from the tuber cinereum of the hypothalamus that connects the posterior pituitary to brain. In the infundibulum the thin non-myelinated axons are ensheathed by typical astrocytes [1]. As the axons continue into the posterior lobe, the astrocytes are replaced by pituicytes, which are dendritic in origin.
Blood Supply of the Pituitary
The pituitary gland receives arterial supply by a single inferior and several superior hypophyseal arteries, which arise from the internal carotid artery. Branches of these arteries supply the posterior pituitary directly and supply the anterior pituitary indirectly via the hypophyseal portal venous system [1].
The inferior hypophyseal artery originates from the cavernous part of the internal carotid artery and divides into medial and lateral branches. These branches anastomose and form a ring around the infundibulum. Branches from this circular anastomosis enter the posterior lobe to supply its capillary bed [1]. The median eminence and the upper part of the infundibulum receive an arterial supply from the superior hypophyseal arteries which arise from the supraclinoid part of the internal carotid artery and the anterior and posterior cerebral arteries [1]. The lower part of the infundibulum and pars posterior receives its supply directly from the inferior hypophyseal artery and indirectly from the superior hypophyseal artery via the trabecular arteries [1].
The hypophyseal portal system that supplies the anterior lobe consists of long and short portal vessels. The long vessels arise from the external capillary plexus and the posterior part of the internal capillary plexus of the median eminence. The two plexi form a continuum with the infundibular capillary plexus which drains into the long portal veins [1]. The long portal veins allow secretions from the median eminence to reach the anterior pituitary directly. The short portal vessels come from the capillary net of the lower infundibulum. The portal system is essential for the endocrine function of the anterior pituitary, since hormone releasing and inhibiting factors secreted from the hypothalamic nuclei collect in the median eminence and infundibulum and are transferred directly to the anterior pituitary without the dilution and metabolism that would occur if they were transported via systemic venous drainage to the heart and arterial supply back to the anterior pituitary.
The venous drainage of the anterior pituitary is restricted and only a few efferent vessels connect directly to the systemic veins of the cavernous sinuses. Venous drainage of the posterior pituitary is via the inferior hypophyseal vein to the dural sinuses, via the long and short portal veins to the anterior pituitary, and via capillaries passing through the median eminence to the hypothalamus.
Embryology of the Pituitary
Studies in different species have demonstrated that pituitary development is highly conserved from lower vertebrates through to higher mammals [9]. The mature pituitary gland has a dual embryonic origin—the anterior and intermediate lobes of the pituitary derive from the oral ectoderm, while the posterior pituitary derives from the neural ectoderm.
The pituitary or adenohypophyseal placode originates from the midline of the anterior neural ridge which is the most anterior part of the neural plate in the embryo and forms the boundary between the anterior part of the ectoderm and the neuroectoderm [10]. The pituitary placode is immediately adjacent to the neural plate cells that give rise to the telencephalon, hypothalamus and posterior pituitary.
The pituitary placode is the site of an invagination of the oral ectoderm called Rathke’s pouch which will give rise to the anterior and intermediate lobes of the pituitary (Fig. 32.2). In humans, the oral ectoderm containing the pituitary placode is formed by the third week of gestation. A week later Rathke’s pouch begins to develop and by the end of the sixth gestational week the initial invagination completely disconnects from the oral ectoderm.
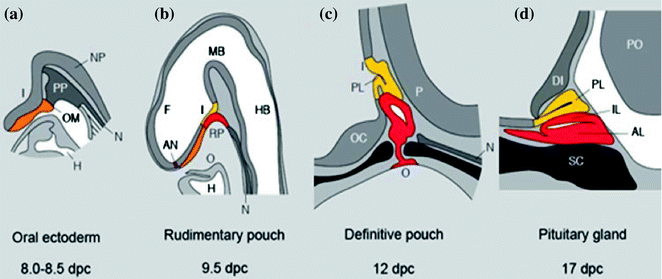
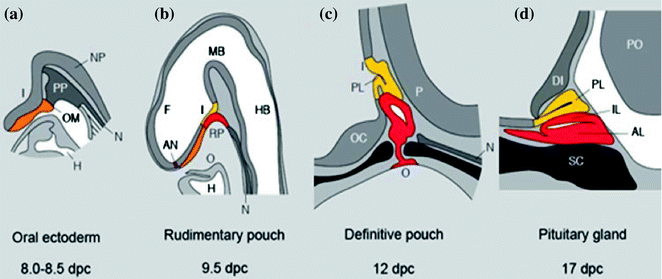
Fig. 32.2
Mouse pituitary development in sagittal section. Stages of development are indicated in dpc. AL Anterior lobe, AN anterior neural pore, DI diencephalon, F forebrain, H heart, HB hindbrain, I infundibulum, IL intermediate lobe, MB midbrain, N notochord, NP neural plate, O oral cavity, OC optic chiasm, OM oral membrane, P pontine flexure, PL posterior lobe, P pons, PP prechordal plate, RP Rathke’s pouch, SC sphenoid cartilage. Adapted from Sheng and Westphal [21], with permission from Elsevier
Pituitary development has been extensively studied in mice and the detailed description that follows refers to this species. Anterior pituitary development occurs in four stages: (1) formation of the pituitary placode, (2) development of a rudimentary Rathke’s pouch, (3) formation of the definitive Rathke’s pouch, and (4) terminal differentiation of various cell types [9].
At 7.5 dpc, the pituitary placode develops as a thickening of the ectoderm at the roof of the primitive oral cavity. The pituitary placode makes contact with the floor of the ventral diencephalon and this is a critical event in the development of the pituitary. At approximately 9 dpc, the oral ectoderm invaginates to form a rudimentary Rathke’s pouch, the primordium of the anterior pituitary while the ventral diencephalon evaginates to form the infundibulum and the posterior pituitary (Fig. 32.2). Subsequently, the definitive Rathke’s pouch is formed and the spatial and temporal differentiation of the various cell types within the mature anterior pituitary gland takes place [11]. At approximately 12.5 dpc, differentiated corticotrophs appear in the ventral region of the pouch and thyrotrophs on 13.5 dpc. Differentiated melanotrophs appear in the intermediate lobe a day later, and somatotroph, lactotroph and gonadotroph cells arise temporally between 15.5 and 16 days. By 17.5 dpc all hormone-secreting cell types have undergone terminal differentiation and are organised into distinct spatial networks within the gland [11].
The juxtaposition of the oral ectoderm forming Rathke’s pouch and the neural ectoderm of the diencephalon which later develops into the hypothalamus is maintained in the early stages of pituitary organogenesis [11]. Inductive tissue interactions resulting from this contact and extrinsic signalling from the neuroectoderm of the infundibulum are critical for the initial development of the pituitary gland [12]. A cascade of signalling molecules and transcription factors play crucial roles in organ commitment, cell proliferation, cell patterning and terminal differentiation events within the developing pituitary.
HESX1, PROP1, POUIF1/PIT1, LHX3, LHX4, TBX19 (TPIT), PITX1, PITX2, SF1, SOX3 and SOX2 are transcription factors implicated in pituitary organogenesis (Fig. 32.3) and mutations in these factors in humans are associated with septo-optic dysplasia, combined pituitary hormone deficiency, isolated growth hormone deficiency, or isolated adrenocorticotropic hormone deficiency (Table 32.1). This is not an exhaustive list and the roles of other transcription factors are being defined. Transcription factors act as activators or repressors and play a significant role in the cell type specification and cell fate within the pituitary gland.