(1)
Institut Bergonié, Bordeaux, France
Abstract
The phosphatidylinositol 3-kinase (PI3K) pathway is one of the signalling pathways activated following the interaction of a growth factor with a tyrosine kinase receptor (TKR). It follows a parallel path to the MAP kinase pathway, and similarly, it operates after binding a phosphotyrosine residue of the receptor to an adapter protein. It consists of sequential activations of kinases and leads to multiple effects on cell growth, proliferation and survival.
This pathway is especially interconnected with the MAP kinase pathway through the RAS proteins; it is, in addition, able to integrate metabolic and nutritional signals which allow to coordinate cell growth and proliferation to nutrient availability. This is the pathway adopted by insulin, which can be considered by some aspects as a growth factor, as mentioned in Chap. 1. Multiple oncogenic alterations have been identified in this pathway, which contains for this reason numerous potential targets for therapeutic approaches.
3.1 From Phosphatidylinositol 3-Kinase to AKT Proteins
Phosphatidylinositol 3-kinases (PI3Ks) are a family of lipid kinases related to serine/threonine kinases. They function as heterodimers consisting of a catalytic subunit and a regulatory subunit. In the main PI3Ks, responsible for the proliferation pathway described in this chapter, the catalytic subunit (p110) is encoded by the PIK3CA gene, and the regulatory subunit (p85) is encoded by the PIK3R1 gene. This regulatory subunit bears a SH2 domain allowing binding to phosphotyrosine residues of activated TKRs, which induce the activation of the catalytic subunit (Fig. 3.1). In some cases, especially for the growth factors of the insulin family (Chap. 1), p85 activation occurs via an adapter protein, IRS1 or 2 (insulin receptor substrate 1 or 2); this protein is phosphorylated by the IGF1R receptor after binding to phosphotyrosine residues, and the phosphotyrosine residues thus generated on IRSs are recognised by the SH2 domain of p85.
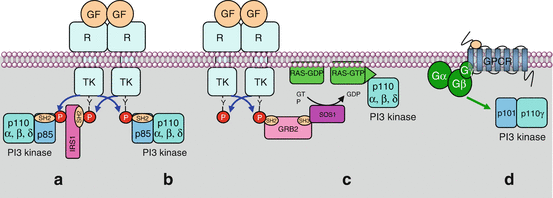
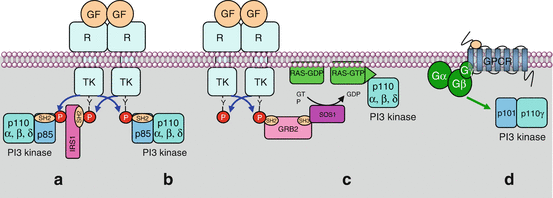
Fig. 3.1
Activation of PI3 kinase by different ways. The regulatory subunit of class I PI3 kinases (p85) (α, β or δ enzymes) can recognise a phosphotyrosine residue of an activated tyrosine kinase receptor (TKR), either directly (b) or via an adapter protein such as IRS1 (insulin receptor substrate 1) (a). The catalytic subunit of PI3 kinase (p110) can also be activated by an activated (GTP-bound) RAS protein (c) or by a heterotrimeric large G-protein activated in response to a GPCR (d). In that case, this is the γ enzyme that is activated
PI3 kinase can also be activated by the RAS protein of the MAP kinase pathway (Chap. 2). The catalytic subunit bears, in its C-terminal portion, a binding site for RAS–GTP. This is a major interconnection between the two signalling pathways, and its consequences are important in the field of targeted therapies. Finally, some PI3 kinases can be activated by large heterotrimeric G-protein-coupled receptors (GPCR) (Chap. 6) (Fig. 3.1).
PI3 kinases catalyse the phosphorylation at position 3 of the inositol moiety of a membrane lipid, phosphatidylinositol 4,5-bisphosphate, to generate phosphatidylinositol 3,4,5-trisphosphate (PIP3) (Fig. 3.2). In contrast to the important signalling pathway involving phospholipases Cβ or γ, which generate 1,4,5-trisphosphoinositol (IP3) as a second messenger (Chap. 6), the message resides in the presence of a phosphate group at the position 3 of the intact lipid, because this phosphate group can be recognised and bound by proteins equipped with a particular domain called pleckstrin homology domain (PH).
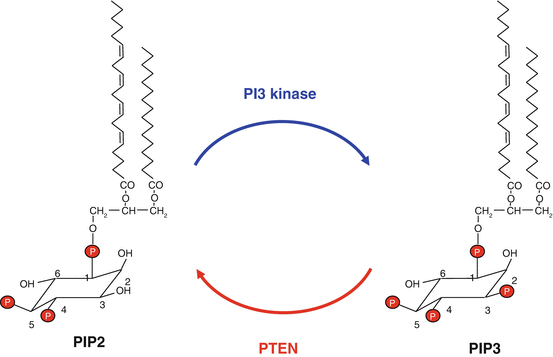
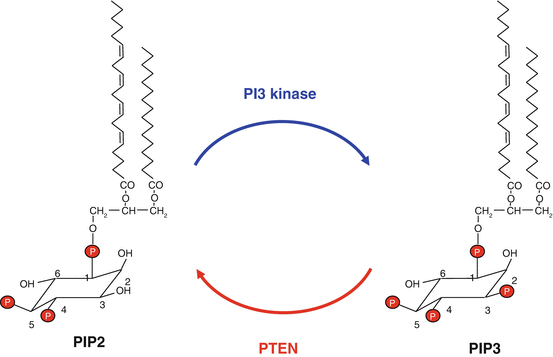
Fig. 3.2
Catalytic activities of PI3 kinase and PTEN. PI3 kinase converts phosphatidylinositol 4,5-bisphosphate (PIP2) into phosphatidylinositol 3,4,5-trisphosphate (PIP3); PTEN catalyses the reverse reaction. Phosphatidylinositol consists of a glycerol skeleton esterifying two fatty acid moieties, generally palmitic acid (C16:0) at position 1 and arachidonic acid (20:4) at position 2 and an inositol (hexahydroxy-cyclohexane) -1-phosphate moiety at position 3
There are indeed three different classes of PI3 kinases, and four members in class I, of interest in this chapter. They are noted PI3Kα, β, γ and δ and have distinct tissue distribution and distinct precise phosphoinositide substrate specificity. Their catalytic subunit is called p110α, β, γ or δ (genes PIK3CA to PIK3CD), and they are activated by diverse regulatory subunits, p85α, p85β or p55 (genes PIK3R1 to PIK3R3) for PI3Kα, β and δ, whereas distinct regulatory subunits, p101 and p84/87 (genes PIK3R5 and PIK3R6), are required for PI3Kγ. These class I PI3 kinases differ according to their capacity of being activated by TKRs, GPCRs, cytokines, integrins and/or RAS proteins. Class II and III PI3 kinases are much less known, and their functions rather concern membrane traffic and receptor internalisation.
The phosphorylation catalysed by PI3K is counterbalanced by dephosphorylation mediated by a lipid phosphatase, PTEN (phosphatase and tensin homologue). This phosphatase ensures the negative regulation of this signalling pathway. It also intervenes as protein phosphatase in other signalling pathways. Figure 3.3 presents the whole PI3 kinase pathway, from the activated receptor to the ultimate effectors.
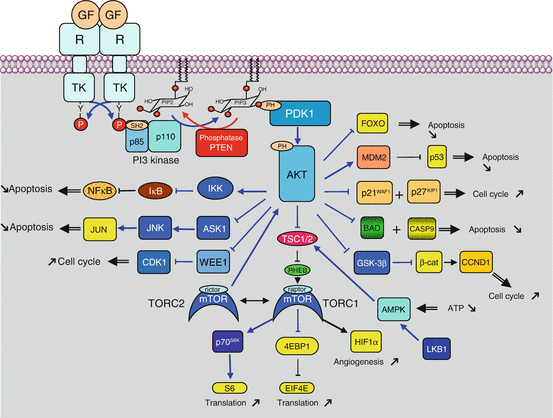
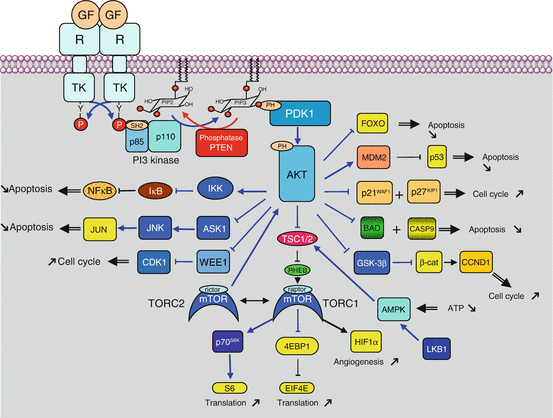
Fig. 3.3
PI3 kinase pathway after activation by a TKR. After activation of a tyrosine kinase receptor (TKR) by a growth factor (GF), the PI3 kinase regulatory subunit (p85) binds a phosphotyrosine residue of the activated receptor via an SH2 domain. The PI3 kinase catalytic subunit (p110) then phosphorylates PIP2 into PIP3, while the PTEN phosphatase catalyses the reverse reaction. The phosphate group at position 3 of the inositol moiety of PIP3 is recognised and bound by serine/threonine kinases equipped with a PH domain, PDK1 and AKT, with the first one phosphorylating the second. AKT is activated by this phosphorylation and can catalyse the phosphorylation of a large array of protein substrates, which are thus activated or inhibited. AKT substrates include TSC2, which is responsible for the control of mTOR activation (which will be detailed in the next figure), BAD, MDM2, p21CIP1, p27KIP1, GSK3β, IKK, WEE1, ASK1, FOXO3 and others. All these proteins are involved in cell survival and proliferation, through the control of apoptosis, cell cycle entry or protein synthesis
The presence of a phosphate moiety at position 3 of an inositol-containing lipid enables to recruit to the membrane PH domain-containing protein kinases, especially PDK1 (phosphoinositide-dependent kinase 1, gene PDPK1), and the AKT proteins, also known as PKBs (protein kinase B). Likely because of a particular arrangement of these two kinases when bound to phosphoinositides, PDK1 can phosphorylate and activate AKT; this occurs on a threonine residue, Thr308 (Thr309 for AKT2 and AKT3), located in the catalytic centre. Another PH domain-containing kinase is Bruton kinase (BTK), which is specifically expressed in B lymphocytes (Chap. 13).
3.2 AKT Proteins and Their Substrates
There are three AKT proteins, AKT1, 2 and 3, the most common (and the most commonly involved in cancer) being AKT1. They have a catalytic function of serine/threonine kinase, they are activated by phosphorylation and they activate or deactivate by phosphorylation a large variety of proteins, including other serine/threonine kinases. Among the main substrates of AKT proteins, and without the possibility of being exhaustive, one can mention (Fig. 3.3):
The TSC2 (tuberous sclerosis complex 2) protein, also known as tuberin. This protein is inhibited by AKT-induced phosphorylation on Ser939 and Thr1462 residues; once combined with TSC1 (hamartin), the complex constitutes a GTPase-activating protein (GAP) for a small G-protein of the RAS family called RHEB (RAS homologue enriched in the brain); this pathway leads to mTOR activation (see below).
BAD (BCL2-associated agonist of cell death), which is a ‘BH3-only’ protein involved in the positive regulation of mitochondrial apoptosis (Chap. 18); this protein is inactivated by phosphorylation.
MDM2 (murine double-minute p53 binding) protein, activated by phosphorylation, which is the E3 ubiquitin ligase of protein p53 (see Chap. 17); MDM2 activation is thus in opposition to the p53 effects on cell cycle arrest and apoptosis induction.
p21CIP1 (gene CDKN1A) and p27KIP1 (CDKN1B), which are CDK (cyclin-dependent kinase) inhibitors and therefore are negative regulators of the advancement of cells in the G1 phase of the cycle (see Chap. 17); AKT-mediated phosphorylation inhibits these CDK inhibitors.
GSK3β (glycogen synthase kinase 3β), which integrates signals of metabolic origin (see below) and which plays an important role in the Wnt pathway (see Chap. 7). This protein is inhibited by AKT-mediated phosphorylation; as a consequence, GSK3β can no longer phosphorylate β-catenin, and this last protein can relocalise in the nucleus and activate transcription programmes leading to cell proliferation and cell cycle entry, instead of being directed to the proteasome for proteolysis.
IKK (IκB kinase), activated by phosphorylation, which phosphorylates and inhibits the IκB (inhibitor of NFκB) protein, an inhibitor of the transcription factor NFκB (Chap. 12); as a consequence, AKT positively regulates NFκB, which is involved in cell survival, proliferation, invasion, angiogenesis and chemoresistance.
WEE1, inhibited by this phosphorylation, which is a CDK1-inhibiting kinase, a master component of mitosis triggering (Chap. 17);
ASK1 (apoptosis signal-regulating kinase 1), inhibited by AKT-mediated phosphorylation, which is an MAP3K activating the JNK pathway (Chap. 2) involved in apoptosis induction in response to proapoptotic signals and to endothelial reticulum stress (ERS) (Chap. 18 and Annex C).
FOXO (forkhead box class O), already mentioned in Chap. 2, which are apoptosis-inducing transcription factors acting both on the extrinsic pathway (TRAIL, FASL) and on the mitochondrial pathway (BH3-only proteins) (Chap. 18); their phosphorylation by AKT on Thr32, Ser253 and Ser315 induces their cytoplasmic relocalisation and the loss of their transcriptional functions.
All AKT actions, therefore, drive the cells toward proliferation and survival.
3.3 mTOR Protein and the TORC Complexes
The mTOR protein (mammalian target of rapamycin, now mechanistic target of rapamycin) was named by analogy with a yeast protein that is inhibited by a natural product, rapamycin, after binding to a small protein, FKBP12 (FK506-binding protein 12). This is a serine/threonine kinase operating downstream AKT and is involved in the phosphorylation of a variety of substrates. mTOR, as well as the PI3Ks, belongs to the family of PIKKs (phosphoinositide 3-kinase-related protein kinases), like ATM and ATR involved in DNA repair (Chap. 17). A scheme of the various domains of the protein is presented in Fig. 3.4. mTOR is not directly activated by AKT: its activation results from the action of the small G-protein RHEB, mentioned earlier, which is active when combined to GTP. The double inhibition of TSC2 by AKT and of RHEB by TSC2 ultimately results in an activation of mTOR by AKT (Fig. 3.5).
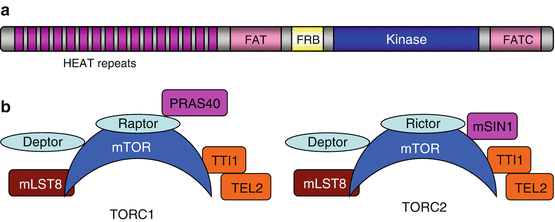
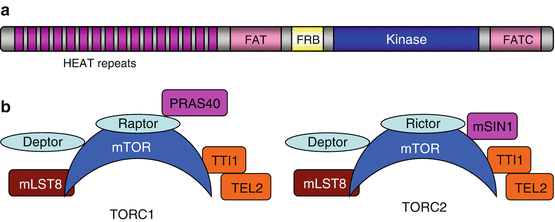
Fig. 3.4
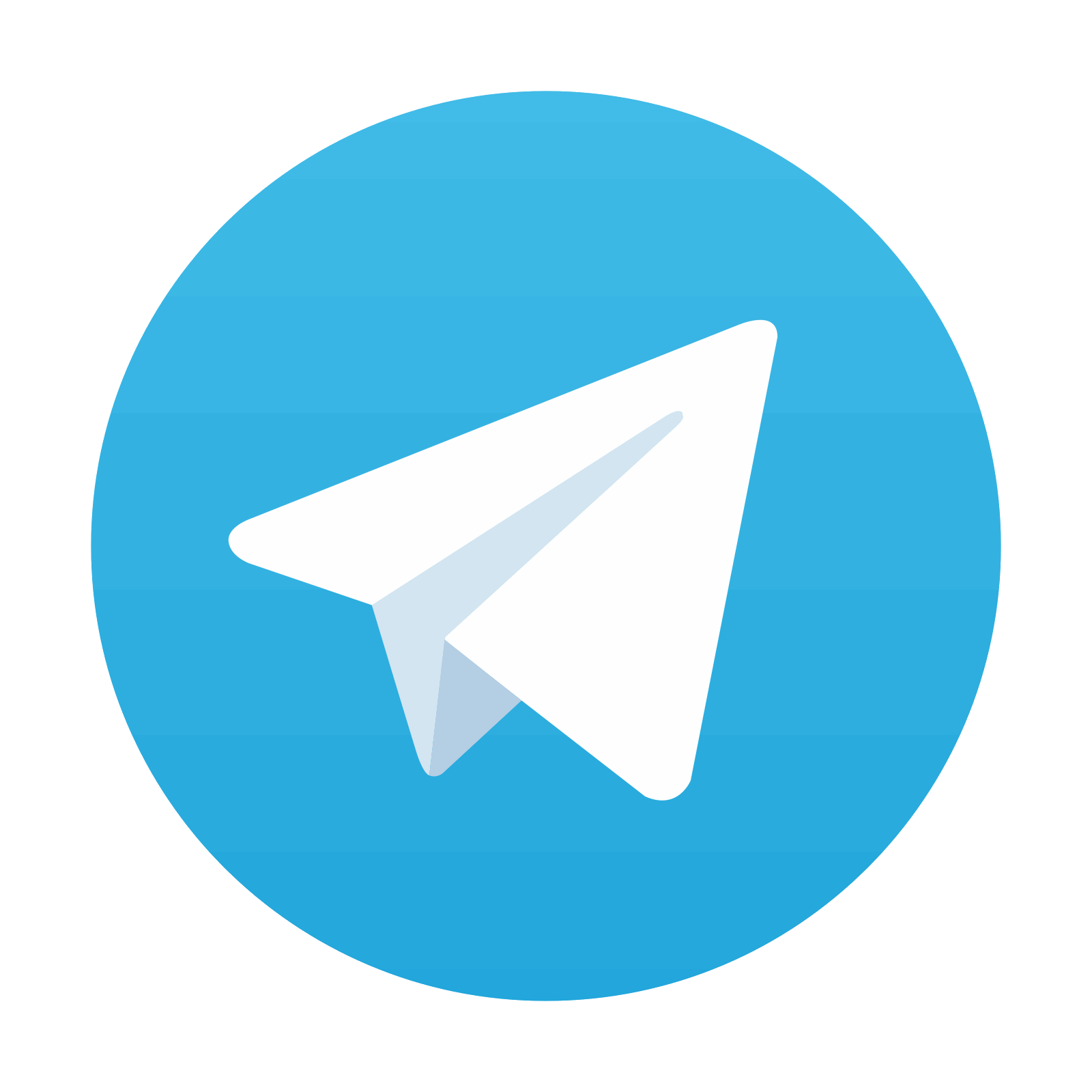
Structural organisation of the mTOR protein and of the TORC complexes. (a) Structural organisation of the mTOR protein. The N-terminal part of the protein contains a tandem repeat of HEAT (antiparallel α-helices found in huntingtin, elongation factor 3, PP2A and TOR) domains, which are involved in protein interactions; an FAT (FRAP, ATM and TRAPP) domain; an FRB (FK506-binding protein 12-rapamycin binding) domain, which is the binding site for the rapamycin-FKBP12 complex; the classical kinase domain, organised in two lobes as for all kinases; and a second FAT domain in the C-terminal part (FATC). (b) Protein composition of the two complexes involving mTOR, TORC1 and TORC2. The two complexes share, in addition to mTOR, the proteins mLST8, TTI1 and TEL2 and the protein DEPTOR (DEP domain-containing mTOR-interacting protein). They differ by their association with RAPTOR (regulatory associated protein of TOR) in TORC1 and RICTOR (rapamycin-insensitive companion of TOR) in TORC2 and of the associated proteins, PRAS40 (or AKT1S1) and mSIN1 (or MAPKAP1), which are substrates of AKT and of a MAPK, respectively
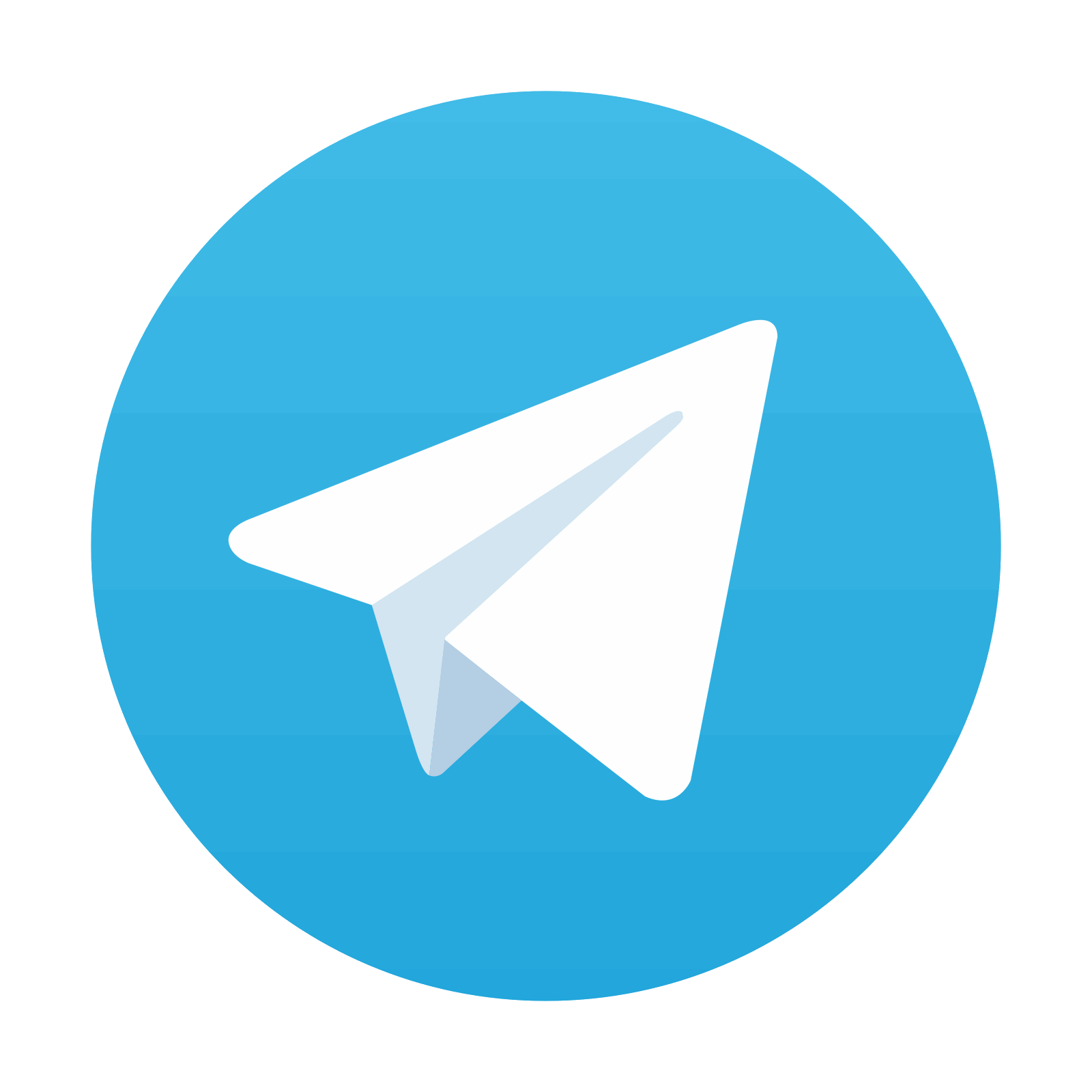
Stay updated, free articles. Join our Telegram channel

Full access? Get Clinical Tree
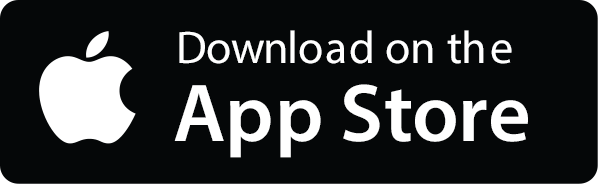
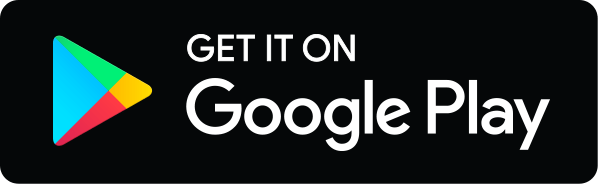
