Fig. 8.1
Catecholamine and metanephrine synthesis pathway . PNMT phenylethanolamine N-methyltransferase, COMT catechol-O-methyltransferase, MAO monoamine oxidase, VMA vanillylmandelic acid
Consequences of Catecholamine Hypersecretion
Catecholamines and metanephrines bind to the seven transmembrane spanning G-protein coupled α- and β-adrenergic receptors and signal through the inositol trisphosphate (IP3) and cyclic adenosine monophosphate (cAMP) second messenger pathways to regulate many cardiovascular, bronchial, and metabolic processes [7]. The hypersecretion of catecholamines and metanephrines leads to numerous symptoms and signs associated with constitutive activation of these pathways including the classic triad of headaches, palpitations, and diaphoresis (Table 8.1) [8–10]. Hypertension, whether sustained, episodic, orthostatic, or relative, is a common consequence of catecholamine over secretion. Surprisingly, a significant number of patients may be asymptomatic, complicating the diagnosis. Severe cardiovascular complications from PCC/PGL include sudden death, arrhythmias, myocardial infarction, and reversible dilated or hypertrophic cardiomyopathy [11]. Acute cardiomyopathy from catecholamine surges occurs in 11% of patients [12]. Given all of these consequences of hormonal hypersecretion, there is high morbidity and mortality when the PCC/PGL go unrecognized or untreated.
Table 8.1
Clinical signs and symptoms of PCC/PGL
Sign/symptom | Range percentage (%) |
---|---|
Classic | |
Hypertension (sustained or episodic) | 67–94 |
Palpitations/tachycardia | 34–58 |
Headache | 33–52 |
Sweating | 31–49 |
Anxiety | 24–35 |
Tremors | 11–26 |
Nonclassic | |
Nausea | 6–22 |
Pain (abdomen/back) | 15–21 |
Shortness of breath | 9–17 |
Weight loss | 6–17 |
Vertigo | 17 |
Absence of symptoms | 8–10 |
Rare | |
Hyperglycemia | |
Fati gue | |
Syncope | |
Nausea/vomiting |
Metastatic PCC/PGL
Another factor associated with increased morbidity and mortality in patients with PCC/PGL is the presence of metastatic disease . Up to 25% of PCC/PGL are malignant (~10% of PCC and ~20% of PGL) [13] defined by the presence of distant metastases where chromaffin tissue is not normally present [1]. Metastatic disease can have a long latency period and occur even up to 20 years after initial diagnosis. The prognosis for patients with metastatic disease is poor with only a 50% 5-year survival rate [13]. Predicting which tumors will become metastatic has proven difficult. Single histopathologic features alone such as vascular or capsular invasion are not helpful in predicting metastases; therefore, identifying biomarkers of malignant potential is an important focus of research.
Two histopathologic scoring systems taking into account multiple features have been developed to try to predict malignant potential [14, 15]. The first system is the pheochromocytoma of the adrenal gland scaled score (PASS) based on a retrospective analysis of 100 adrenal PCC comparing histopathologic features to the clinical behavior over 10 years of follow-up [15]. Points are assigned to each histopathologic finding such as large nesting patterns, high cellularity, presence of necrosis or vascular or capsular invasion, and high number of mitoses or atypical mitoses, for a maximum score of 20 (Table 8.2). This study suggests that tumors with a PASS less than four were always clinically benign, whereas tumors with a PASS of four or higher had the potential to become metastatic. There are several limitations to this system. It was not developed for PGL, and it is unknown if the same criteria apply. Most importantly, the PASS has extremely high inter-observer and intra-observer variability [16], making it unreliable, and consequently, most centers do not use it.
Table 8.2
Histopathology scoring systems for predicting metastatic potential in PCC/PGL
Histopathologic feature | Pheochromocytoma of the adrenal gland scaled score; points | Grading system for adrenal pheochromocytoma and paraganglioma; points |
---|---|---|
Histology pattern | • Large nests or diffuse growth = 2 | • Zellballen = 0 |
• Large or irregular nests = 1 | ||
• Pseudorosettes = 1 | ||
Cellularity | • High = 2 | • Low: < 150 cells/HPF = 0 |
• Moderate: 15–200 cells/HPF = 1 | ||
• High: > 250 cells/HPF = 2 | ||
Necrosis | • Present = 2 | • Absent = 1 |
• Present = 2 | ||
Invasion | • Vascular invasion = 1 | • Absent – vascular or capsular = 0 |
• Capsular invasion = 1 | • Present – vascular or capsular = 1 | |
Proliferation | • Mitotic figures = 2 (> 3/10 HPF) | • Ki67 index < 1% = 0 |
• Ki67 index 1–3% = 1 | ||
• Ki67 index > 3% = 2 | ||
• Atypical mitoses = 2 | ||
Other features | • Extension to adipose tissue = 2 | • Epinephrine elevated with or without norepinephrine = 0 |
• Cell spindling = 2 | ||
• Cellular monotony = 2 | • Norepinephrine and/or dopamine but without epinephrine = 1 | |
• Nuclear pleomorphism = 1 | ||
• Nuclear hyperchromasia = 1 | • Non-secreting = 0 | |
Maximum score | 20 | 10 |
Histological grade | Not applicable | • Well differentiated (scores 0–2) |
• Moderately differentiated (scores 3–6) | ||
• Poorly differentiated (scores 7–10) |
The second scoring system is the Grading System for adrenal pheochromocytoma and Paraganglioma (GAPP) which scores both PCC and PGL to a maximum of 10 points based on many similar histopathology features as the PASS and includes additional points for the Ki67 proliferation index and biochemical secretion (Table 8.2) [14, 17]. In the GAPP scoring system, tumors are divided into well-differentiated (scores 0–2), moderately differentiated (scores 3–6), and poorly differentiated (scores 7–10) categories similar to the grading system for pancreatic neuroendocrine tumors (PNETS). In a cohort of 163 PCC/PGLs, the GAPP distinguished between benign and malignant PCC/PGL with mean scores ±SEM of 2.08 ± 0.17 and 5.33 ± 0.43, respectively [17]. While the GAPP is promising, it is likely subject to similar intra- and interobserver variability as the PASS. In addition, the GAPP has not been validated by any independent groups and, therefore, cannot yet be applied clinically.
To date, the only reliable predictor of an increased risk of malignancy is a germline SDHB mutation, and about half of patients with metastatic disease are SDHB mutation carriers [13, 18]. In addition, tumors larger than 4–5 cm and/or secreting methoxytyramine, a dopamine metabolite (not yet clinically available in most centers), are associated with an increased risk of malignancy [13, 19]. Unfortunately, the current treatment options for patients with widely metastatic PCC/PGL are limited and none are curative. As the molecular differences between clinically benign and aggressive tumors are investigated, researchers may identify additional biomarkers for malignant transformation as well as potential therapeutic targets.
PCC/PGL Genetics
For a long time, it was taught that PCC/PGL are the “tumor of tens” where 10% of tumors are associated with hereditary conditions. It is now known that the percentage of tumors from patients with germline mutations is much larger [18, 20]; and in addition, genomic studies have identified some common somatic mutations that drive tumorigenesis. In fact, researchers have identified the driver mutation (germline and somatic) in at least 60% of PCC/PGL [20]. However, there is still much to learn about the pathogenesis of disease in the remaining subset of tumors with no identified driver mutation and much to understand with regard to the differing rates of malignant transformation even among tumors with similar driver mutations.
Germline Genetics
A unique feature of PCC/PGL is the extensive list of susceptibility genes which when mutated lead to an increased risk of tumor development. Up to 40% of patients with a PCC/PGL have a germline mutation in a susceptibility gene, making this tumor type the most common solid tumor to be associated with cancer risk genes [18, 20]. The American Society for Clinical Oncology suggests that clinical genetic testing be offered for any cancer syndrome which has at least a 10% risk of having an inherited gene mutation [21, 22]. Therefore, it has been recommended that all patients with PCC/PGL be offered clinical genetic testing [23] to help guide screening, treatment, and surveillance for patients and their family members who might be mutation carriers. Recognition of the diverse cancer syndromes associated with PCC/PGL, and the wide range of genetic mutations causing them, has greatly increased our understanding of the pathophysiology of PCC/PGL (Table 8.3).
Table 8.3
Genetic syndromes associated with PCC/PGL
Syndrome | Gene | Inheritance | Associated symptoms/signs |
---|---|---|---|
Neurofibromatosis type 1 | NF1 | Autosomal dominant | Diagnostic clinical criteria (at least two) |
• Cutaneous neurofibromas (at least two) | |||
• Plexiform neurofibromas | |||
• Café au lait spots (at least six, greater than or equal to 0.5cm in prepubertal patients and to 1.5 cm in postpubertal patients) | |||
• Lisch nodules (benign iris hamartoma) | |||
• Inguinal or axillary freckling | |||
• Long bone dysplasia | |||
• Optic gliomas | |||
• First degree relative with NF1 | |||
Increased risk but not part of diagnostic criteria | |||
• PCC (possibly bilateral) | |||
• Malignant peripheral nerve sheath tumors | |||
• Juvenile myelomonocytic leukemia | |||
von Hippel-Lindau | VHL | Autosomal dominant | • Hemangioblastomas of the CNS (including retina) |
• Endolymphatic sac tumors | |||
• Epididymal cystadenomas | |||
• PCC (possibly bilateral) | |||
• Renal cell carcinomas | |||
• Renal cysts | |||
• Pancreatic neuroendocrine tumors | |||
• Pancreatic cysts | |||
Multiple endocrine neoplasia type 2 | RET | Autosomal dominant | Classic type 2A |
• Medullary thyroid cancer | |||
• PCC (possibly bilateral) | |||
• Hyperp arathyroidism | |||
Type 2B | |||
• Medullary thyroid cancer | |||
• PCC (possibly bilateral) | |||
• Marfanoid habitus | |||
• Mucocutaneous neuromas | |||
• Gastrointestinal ganglioneuromas | |||
Hereditary PGL syndrome | SDHA | Autosomal dominant (SDHD, SDHAF2 – paternal inheritance) | • EAPGL (SDHB, SDHD, SDHC, SDHA) |
SDHB | • HNPGL (SDHD, SDHC, SDHAF2, rare SDHB) | ||
SDHC | • PCC (SDHB, SDHD, rare SDHC) | ||
SDHD | • Renal cell carcinoma (clear cell) | ||
SDHAF2 | • GI stromal tumors | ||
• Pituitary adenoma rare | |||
Familial PCC/PGL syndrome | MAX | Autosomal dominant | • PCC (bilateral) |
Familial PCC/PGL syndrome | TMEM127 | Autosomal dominant | • PCC |
• EAPGL and HNPGL possible | |||
• Renal cell carcinoma (clear cell) rare | |||
Polycythemia PGL syndrome | EPAS1 | Autosomal dominant (often somatic mosaic) | • Polycythemia |
• PCC/PGL | |||
• Somatostatinoma | |||
Hereditary leiomyomatosis and renal cell cancer syndrome | FH | Autosomal dominant | • Cutaneous and uterine leiomyomas |
• Renal cell carcinoma (type 2 papillary) | |||
• PCC/PGL rare |
Genetic Syndromes Associated with PCC/PGL
Neurofibromatosis Type 1
Neurofibromatosis type 1 (NF1), also called von Recklinghausen’s disease, is an autosomal dominant cancer syndrome occurring in 1 in 2800 individuals and caused by loss of function mutations in the neurofibromatosis Type 1 (NF1) tumor suppressor gene [24]. NF1 is located on chromosome 17q11.2 and encodes the large 2818 amino acid protein neurofibromin. The most well-defined role of neurofibromin is as a GTPase-inactivating RAS and, thereby, regulating both the PI3K/mTOR (mammalian target of rapamycin) and mitogen-activated protein kinase (MAPK) pathways to control cell proliferation and differentiation [25]. When NF1 is mutated, there is uncontrolled activation of these pathways leading to tumor formation.
Traditionally, NF1 is diagnosed based on clinical criteria rather than genetic testing due to the large size of the gene and lack of mutational hotspots. Up to 50% of patients with NF1 have de novo mutations, and there is variable penetrance and expressivity for the disease even in those family members with the same mutation [26]. The clinical diagnosis is made when patients have two or more of the following characteristics: six or more café au lait spots of varying size based on pubertal status, two or more neurofibromas or one plexiform neurofibroma, Lisch nodules (benign iris hamartomas), optic glioma, skeletal dysplasia (thinning of the long bones), or a first degree family member with NF1 (Table 8.3) [24]. Although not part of the diagnostic criteria, patients with NF1 also are at increased risk of developing PCC, malignant peripheral nerve sheath tumors, and juvenile myelomonocytic leukemia. The prevalence of PCC in patients with NF1 varies from 1 to 15% depending on case detection testing [27–29]. Guidelines for treating patients with NF1 recommend screening for PCC in individuals with hypertension [24]. PCCs in NF1 are usually unilateral, but can be bilateral, and often secrete both epinephrine and norepinephrine. The mean age of diagnosis of PCC in patients with NF1 is 43 years old [30], similar to those with sporadic disease. Although PCCs are rare in individuals with NF1, the rate of malignancy is elevated to approximately 12% [30].
von Hippel-Lindau Disease
von Hippel-Lindau disease (vHL) is another autosomal dominant cancer syndrome occurring in 1 in 36,000 individuals per year and caused by loss of function mutations in the von Hippel-Lindau (VHL) tumor suppressor gene [31]. VHL is located on chromosome 3p25-26 and encodes two isoforms of the von Hippel-Lindau protein (pVHL), an E3 ubiquitin ligase: one full-length 213 amino acid protein and one smaller protein which lacks the first 53 amino acids [32]. Under normoxic conditions, pVHL binds to a hydroxylated proline residue on hypoxia-inducible factor α (HIFα) and marks it for proteasomal degradation by ubiquitination [33]. Under hypoxic conditions, or if pVHL is inactivated by mutations, pVHL will not bind to HIFα which is then free to dimerize with HIFβ, translocate to the nucleus, and act as a transcription factor for downstream genes involved in the hypoxia signaling pathway [32].
vHL is diagnosed based on clinical features and by identifying the VHL gene alteration through clinical genetic testing. Patients with vHL develop a wide range of benign and malignant tumors including renal cysts and clear cell renal cell carcinomas (RCC), pancreatic cysts and PNETS, hemangioblastomas of the central nervous system, endolymphatic sac tumors, and PCC (Table 8.3) [34]. Up to 20% of all patients with vHL will develop a PCC with the mean age of diagnosis at 30 years, and PCC may be the presenting sign of disease [35]. Interestingly, there are strong genotype-phenotype correlations between PCC and VHL mutations [36–41]. Patients with vHL type 1 have truncating gene mutations or deletions, and these patients have a low risk of PC C and a higher risk of RCC. On the other hand, patients with vHL type 2 have missense mutations in VHL and carry a high risk of PCC or RCC depending on the location of the missense mutation. Missense mutations on the surface of pVHL portend a higher risk of PCC than missense mutations in the core of the protein [42]. Mutations that disrupt the interaction between pVHL and HIF are associated with RCC, while mutations in other parts of pVHL are associated with PCC [37, 40, 43, 44]. This suggests that increased HIF activity is related to renal cell carcinoma formation, while other HIF-independent functions of pVHL may be causative for PCC development. However, by cDNA microarray analysis, VHL-associated PCC display a pseudohypoxia gene expression pattern as expected to occur secondary to increased HIF activity [45], so the exact mechanism by which the genotype-phenotype correlations exist remains to be elucidated.
PCC in vHL can be unilateral or bilateral, and there are rare cases of extra-adrenal PGL [46, 47]. Interestingly, vHL-associated PCC tend to secrete norepinephrine despite forming in the adrenal medulla. The reason for this deviation in the usual adrenal medullary hormonal secretion pattern is due to decreased PNMT expression secondary to promoter hypermethylation, leading to a decreased conversion of norepinephrine to epinephrine [48, 49]. The malignant PCC rate in patients with vHL is about 5% [34]. Guidelines suggest that patients with high-risk VHL mutations be screened annually for PCC starting at age 5 [50].
Multiple Endocrine Neoplasia Type 2
Multiple endocrine neoplasia type 2 (MEN2) is the third classic autosomal dominant cancer syndrome associated with PCC/PGL risk. MEN2 occurs in 1 in 30,000 people and is caused by activating mutations in the RET (rearranged during transfection) proto-oncogene. RET is on chromosome 10q11.2 and encodes the 860 amino acid RET protein, a transmembrane tyrosine kinase required for neural crest development [51]. When bound by ligand, the RET protein dimerizes, autophosphorylates, and signals through the PI3K pathway to regulate proliferation and apoptosis [52]. Activating mutations in RET cause constitutive upregulation of PI3K pathway leading to tumor development.
The MEN2 diagnosis is suspected based on clinical features and confirmed by mutation testing of the RET gene. Patients with MEN2 are divided into two groups per the most recent guidelines, MEN2A and MEN2B [53] (Table 8.3). Classical MEN2A is defined by medullary thyroid cancer (MTC) (95% of patients), primary hyperparathyroidism (15–30% of patients), and PCC (50% of patients). MEN2A also includes three additional subtypes: (1) patients with MTC and cutaneous lichen amyloidosis, (2) patients with MTC and Hirschsprung’s disease, and (3) patients who develop only MTC (the old familial MTC subtype of MEN2). MEN2B is defined by MTC (100% of patients), PCC (50% of patients), mucosal ganglioneuromas, and a marfanoid habitus.
Ninety-five percent of patients with MEN2 have type 2A and 5% have type 2B. There are strong phenotype/genotype correlations based on the mutated RET codon. For example, patients with codon 634 mutations have high risk for classical MEN2A, while patients with mutations at codon 918 almost exclusively have the MEN2B phenotype [54]. The 2015 guide lines suggest that patients with high-risk mutations (codons 918, 634, and 883) be screened annually for PCC starting at age 11 and those with moderate-risk mutations (all other codons) be screened annually for PCC starting at age 16 [53]. The mean age at diagnosis of PCC in patients with MEN2 is 28 years [55], and patients develop bilateral PCC more than 50% of the time [55, 56]. There are rare reports of extra-adrenal PGL [46]. MEN2-associated PCC usually have epinephrine predominance [57], and malignant PCC occurs in less than 5% of cases [56].
Hereditary Paraganglioma Syndromes
The hereditary paraganglioma syndromes are autosomal dominant disorders caused by loss of function mutations in one of the succinate dehydrogenase subunit (SDH) genes (SDHA, SDHB, SDHC, SDHD) or the cofactor SDHAF2 [58–62]. The SDH complex, complex II of the mitochondrial respiratory chain, is a highly conserved heterotetrameric protein and the only respiratory chain complex also involved in the Krebs cycle. The SDH complex couples the oxidation of succinate to fumarate with the electron transfer to the terminal acceptor ubiquinone in the electron transport chain. SDHA and SDHB are the catalytic subunits located in the mitochondrial matrix, and these two proteins are anchored to the inner mitochondrial membrane by subunits SDHC and SDHD. Loss of function mutations in the SDH complex subunits result in accumulation of succinate. Because succinate has structural similarity to α-ketoglutarate (also called 2-oxoglutarate), high levels of succinate competitively inhibit 2-oxoglutarate-dependant dioxygenases such as histone and DNA demethylases as well as HIF prolyl-hydroxylases [63, 64]. This inhibition leads to two main downstream consequences: (1) pseudohypoxia conditions because the inhibition of HIF prolyl-hydroxylase enzymes prevents the hydroxylation of the proline residue on HIFα thereby preventing pVHL binding and subsequent proteasomal degradation and (2) global DNA hypermethylation and other epigenetic changes because of the inhibition of histone and DNA demethylases [65, 66]. Mutations in any of the SDH subunits or cofactor AF2 lead to increased risk of PCC/PGL formation as discussed below (Table 8.3).
The SDHB subunit is the most commonly mutated subunit of the complex associated with PCC/PGL. SDHB is located on chromosome 1p36.1 and encodes the 280 amino acid iron sulfur subunit of the SDH complex. Patients with SDHB mutations most often develop extra-adrenal PGL but also are at risk for HNPGL as well as adrenal PCC. The SDHB-associated tumors tend to secrete norepinephrine and/or dopamine, and the mean age at initial diagnosis ranges from 28.7 to 36.7 years [67–69]. Compared to all of the other PCC/PGL susceptibility genes, the risk of malignancy is highest in patients with SDHB associated PCC/PGL (~23%) [70]. Of those patients with metastatic disease, about half have germline SDHB mutations [18].
SDHD is the next most common subunit of the complex that is mutated in association with PCC/PGL. SDHD is located on chromosome 11q23.1 and encodes the 103 amino acids anchoring subunit with an ubiquinone binding site to which the electrons are transferred from the iron sulfur clusters within the SDHB subunit [71, 72]. SDHD mutations are expressed with a parent-of-origin effect, almost exclusively showing paternal inheritance with extremely rare exception [67, 73–76]. Patients with paternally inherited SDHD mutations develop HNPGL and are at risk to develop multiple primary tumors including adrenal PCC and extra-adrenal PGL as well [75, 77, 78]. The mean age at diagnosis is 35.7 years [67], and the rate of malignancy is less than 5% [70]. The SDHD-associated HNPGLs tend to be biochemically silent or produce only dopamine or methoxytyramine, whereas the PCCs can have norepinephrine secretion [57].
The SDHC, SDHA, and SDHAF2 genes are mu tated at a lower frequency in association with PCC/PGL. SDHC is located on chromosome 1q23.3, spans 35 kb, and encodes a large subunit with the cytochrome b in the SDH complex. Patients with SDHC mutations develop HNPGL and thoracic PGL most commonly, although PCC/PGL in other locations can be seen [79–81]. The mean age at initial diagnosis is 29–38 years [67, 79], and there is a negligible risk of malignant disease in SDHC mutation carriers [67, 79]. SDHA is located on chromosome 5q15 and encodes the flavoprotein catalytic subunit of SDH complex. Biallelic mutations in SDHA lead to Leigh’s syndrome (an early onset neurodegenerative disorder) [82, 83]. It was subsequently discovered that SDHA mutations also are associated with PCC/PGL and account for about 3% of PCC/PGL cases with low penetrance [60, 84]. The phenotype associated with SDHA-related PCC/PGL is still being uncovered. SDHAF2 is located on chromosome 11q12.2 and encodes the protein needed for flavanation of the SDHA catalytic subunit. Only a few families have been described with mutations in SDHAF2, and those affected mutation carriers tend to have multiple HNPGL [61, 85, 86]. Similar to SDHD mutations, SDHAF2 mutations show a parent-of-origin effect associated with paternal transmission of disease. The average age of onset is 33 years and the rate of malignancy is negligible.
Inherited SDHx gene mutations are associated with increased risk of developing other cancers in addition to PCC/PGL [87] (Table 8.3). Renal cell carcinoma occurs in about 14% of SDHB and 8% of SDHD mutation carriers [75]. SDHC and SDHA mutation carriers also are at higher risk than the general population to develop RCC albeit at a low frequency [88]. In addition, all SDHx mutation carriers are at increased risk for developing gastrointestinal stromal tumors (GISTs) [89], and numerous case reports suggest an association between SDHx mutations and pituitary adenomas [90]. Thus far, no strong genotype/phenotype correlations have been discovered between the specific mutation types and the spectrum of disease.
There are no formal guidelines for screening asymptomatic or unaffected SDHx mutation carriers. PCC/PGL have been reported in children as young as 5 years old, yet the penetrance of PCC/PGL and the other SDHx-associated tumors is not well defined. Most experts suggest screening for SDHx-associated tumors in asymptomatic mutation carrier patients with whole body MRI every 2–5 years starting between ages 5 and 10 along with annual biochemical screening for PCC/PGL.
Familial Pheochromocytomas and Paragangliomas
Several families with inherited PCC/PGL had none of the above susceptibility gene mutations. Applying genomic approaches to DNA and RNA from tumors from these families, identified additional susceptibility genes as causing autosomal dominant transmission of PCC/PGL, including TMEM127, MAX, and FH (Table 8.3), discussed below. In addition, there are rare case reports of families with germline mutations in MDH2, KIF1B, and EGLN1 [91–93].
The TMEM127 gene is located on chromosome 2q11.2 and loss of function mutations lead to PCC/PGL [94]. TMEM127 encodes transmembrane protein 127 believed to play a role in mTORC1 signaling pathway [94]. The patients with germline TMEM127 mutations have a low penetrance of disease. These mutation carriers can develop PCC/PGL in any location, often having bilateral adrenal PCC [95, 96]. The average age of onset is similar to sporadic tumors at 45 years old, and the rate of malignancy is low. Rare cases of RCC have been found in TMEM127 mutation carriers [97].
The MAX gene, located on chromosome 14q23, was found to be a PCC/PGL susceptibility gene through germline exome sequencing [98]. MAX encodes a basic helix-loop-helix leucine zipper protein called MYC-associated protein X (MAX), which heterodimerizes with MYC or MYC repressors to act as a transcription factor for numerous genes involved in cell proliferation, differentiation, and apoptosis [99, 100]. Patients with MAX mutations tend to have adrenal PCC which are often bilateral and can be multifocal within a single adrenal gland; furthermore, there may be an association with an increased risk of malignancy, although this has not been replicated in a second cohort study [98, 101]. The number of patients with MAX mutations identified thus far is quite small making the phenotype difficult to define.
Inactivating mutations in the fumarate hydratase (FH) gene cause hereditary leiomyomatosis and Renal Cell Cancer (HLRCC) syndrome which is an autosomal dominant condition where patients develop smooth muscle tumors (leiomyomas) and type 2 papillary renal cell carcinoma [102]. Rare families with PCC/PGL have been found to carry germline FH mutations, and there may be an associated increased risk of metastatic disease [103, 104]. The FH gene is located on chromosome 1q42.1 and encodes the enzyme fumarate hydratase which converts fumarate to malate in the Krebs cycle. PCC/PGL with FH mutations have similar molecular consequences to PCC/PGL with SDHx mutations including pseudohypoxia and hypermethylation as fumarate shares structural similarities to succinate leading to similar inhibition of the 2-oxoglutarate-dependent dioxygenases [64, 103, 104].
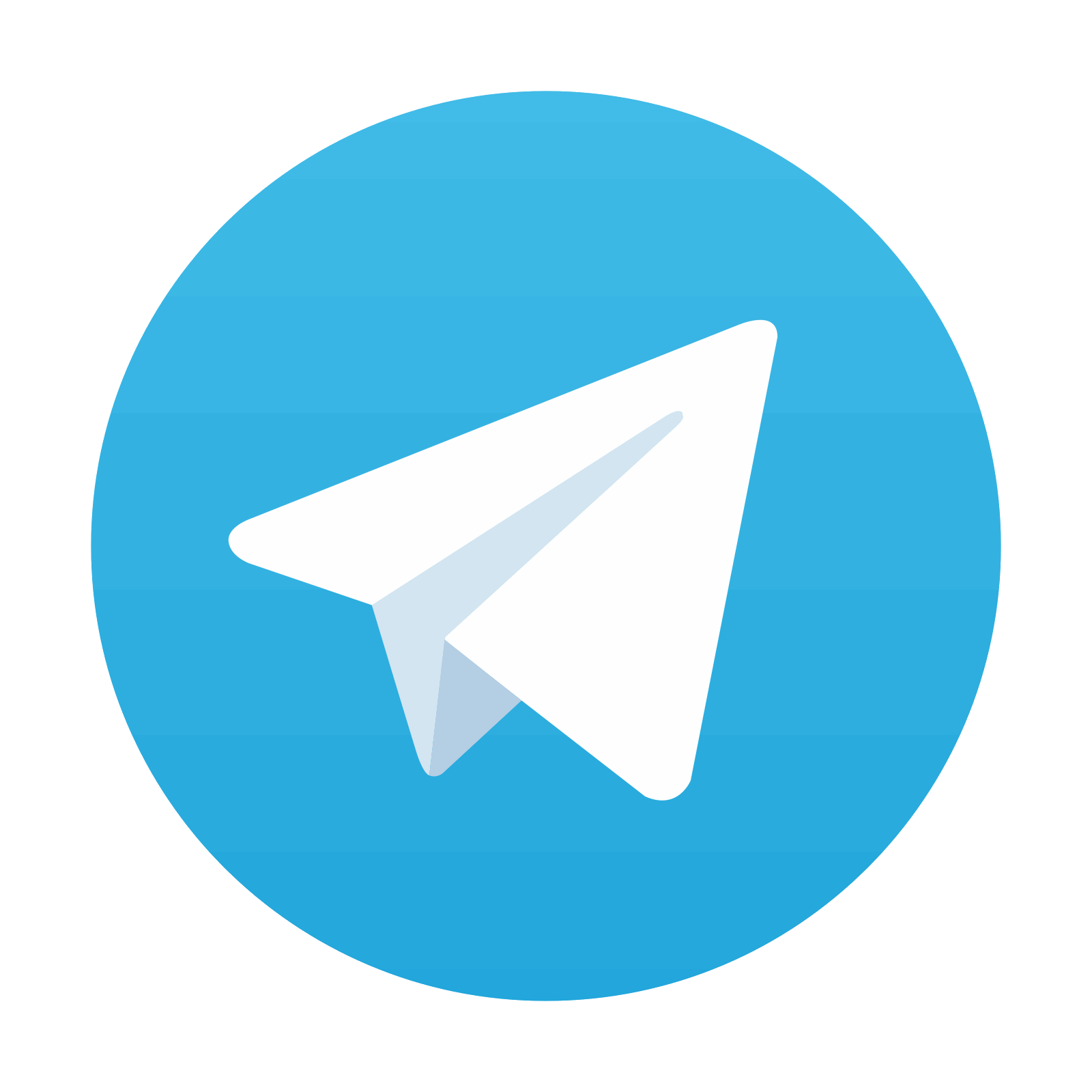
Stay updated, free articles. Join our Telegram channel

Full access? Get Clinical Tree
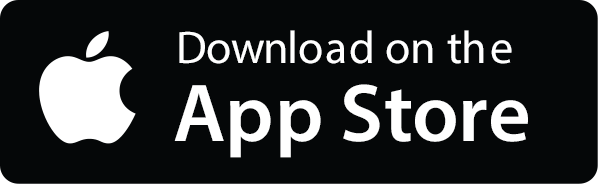
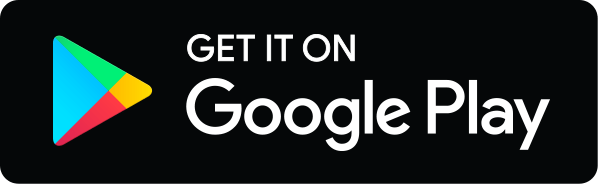