Peritoneal surface malignancy is a common manifestation of intra-abdominal malignancies. Systemic chemotherapy offers no long-term survival and poor quality of life for these patients in their terminal stages of disease. By contrast, cytoreductive surgery combined with perioperative intraperitoneal and intravenous chemotherapy has resulted in encouraging clinical results. Further improvements should come from both clinical phase II/III studies and pharmacologic research. This article reviews the current pharmacologic data and controversies regarding the perioperative intraperitoneal and intravenous application of chemotherapy.
The main teaching points of this article are:
- •
To understand the pharmacologic principle of dose intensification, the main rationale for providing locoregional cancer chemotherapy.
- •
To provide an update on the pharmacologic data available concerning the cancer chemotherapy drugs used in locoregional cancer chemotherapy protocols.
- •
To identify current controversies regarding the use of intraperitoneal and intravenous cancer chemotherapy in patients with peritoneal carcinomatosis.
Introduction
Peritoneal surface malignancy (PSM) is a common secondary manifestation of digestive and gynecologic malignancies alike. Less frequently, PSM originates from a primary peritoneal cancer such as mesothelioma. Despite continuing advances in systemic chemotherapy, no long-term survival is reported in PSM patients with this treatment modality alone. By contrast, cytoreductive surgery (CRS) combined with perioperative intraperitoneal (IP) and intravenous (IV) chemotherapy has resulted in encouraging clinical results in both phase II and phase III trials. Now that the proof of principle for these new treatment modalities has been demonstrated, the question remains as to where further improvement is to be sought. Although further clinical trials are needed, an equally important role exists for pharmacologic research in this setting. A disturbing variety of perioperative chemotherapy protocols is used in PSM patients, based on often scant pharmacologic data. This article reviews the current pharmacologic data and offers guidance toward further improvement and standardization of these cancer chemotherapy regimens.
Dose intensification
One of the main limiting factors governing dosimetry in systemic chemotherapy is hematological toxicity. In other words, the actual dose given IV is not so much the dose one wants to give based on cytotoxicity studies, but rather the dose tolerated by the patient’s hematological reserve. By contrast, the dose intensification offered by the peritoneal membrane after IP administration gives a unique opportunity to expose the residual microscopic intraperitoneal tumor cells in PSM patients after CRS to very high doses of the cancer chemotherapy drug. This concept of dose intensification was first explored by Dedrick and colleagues. In his landmark article of 1978 Dedrick concluded that the peritoneal permeability of several hydrophilic anticancer drugs may be considerably less than the plasma clearance of that same drug. Fig. 1 demonstrates his 2-compartment model. The transport over the membrane is modeled according to the equation:
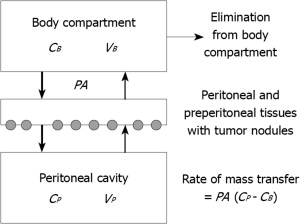
where PA is permeability area (PA = effective contact area × permeability), C P is concentration in peritoneal cavity, and C B is concentration in the blood. Although the equation permits calculation of the pharmacokinetic advantage, the model does not tell anything about the specific penetration of the cancer chemotherapy drug into the tissue or tumor nodule. Neither does it predict the value of the effective contact area. It simply describes the transfer between 2 compartments.
Dose intensification
One of the main limiting factors governing dosimetry in systemic chemotherapy is hematological toxicity. In other words, the actual dose given IV is not so much the dose one wants to give based on cytotoxicity studies, but rather the dose tolerated by the patient’s hematological reserve. By contrast, the dose intensification offered by the peritoneal membrane after IP administration gives a unique opportunity to expose the residual microscopic intraperitoneal tumor cells in PSM patients after CRS to very high doses of the cancer chemotherapy drug. This concept of dose intensification was first explored by Dedrick and colleagues. In his landmark article of 1978 Dedrick concluded that the peritoneal permeability of several hydrophilic anticancer drugs may be considerably less than the plasma clearance of that same drug. Fig. 1 demonstrates his 2-compartment model. The transport over the membrane is modeled according to the equation:
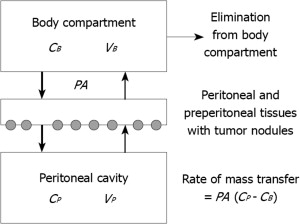
where PA is permeability area (PA = effective contact area × permeability), C P is concentration in peritoneal cavity, and C B is concentration in the blood. Although the equation permits calculation of the pharmacokinetic advantage, the model does not tell anything about the specific penetration of the cancer chemotherapy drug into the tissue or tumor nodule. Neither does it predict the value of the effective contact area. It simply describes the transfer between 2 compartments.
Peritoneal membrane
The rationale of administering chemotherapeutic drugs into the peritoneal cavity is based on the relative transport barrier formed by the tissue surrounding the peritoneal space. The peritoneum is a complex 3-dimensional organ covering the abdominopelvic organs and the abdominal wall, and contains a potentially large space. The most elaborate description of the ultrastructure of the peritoneum in man was presented in 1941 by Baron. The peritoneum consists of a monolayer of mesothelial cells supported by a basement membrane and 5 layers of connective tissue, which account for a total thickness of 90 μm. The connective-tissue layers include interstitial cells and a matrix of collagen, hyaluron, and proteoglycans. The cellular component consists of pericytes, parenchymal cells, and blood capillaries. This complex is often referred to as the peritoneal membrane, and the description is a working model derived from research on the peritoneum as a dialysis membrane. Contrary to intuitive thinking, the elimination of the mesothelial lining during peritonectomy procedures does not alter the pharmacokinetic properties of the peritoneum in the transport of chemotherapeutic agents from the peritoneal cavity to the plasma compartment. In a rodent model, Flessner and colleagues demonstrated that neither removal of the stagnant fluid layer on the mesothelium nor removal of the mesothelial lining influences the mass transfer coefficient (MTC) over the barrier. There is indirect evidence supporting this hypothesis in humans in that the extent of the peritonectomy in peritoneal carcinomatosis (PC) patients does little to alter the intraperitoneal chemotherapy pharmacokinetics (PK) of mitomycin C or 5-fluorouracil. Basic research indicates it is the blood capillary wall and the surrounding interstitial matrix that are the principal barriers for clearance of molecules from the abdominopelvic space, not the mesothelial lining.
Pharmacokinetics versus pharmacodynamics
PK describes what the body does to the drug whereas pharmacodynamics (PD) describes what the drug does to the body.
The basic way of depicting PK data is by a concentration × time graph. Fig. 2 shows such a PK graph for IP doxorubicin during hyperthermic intraperitoneal perioperative chemotherapy (HIPEC). The subsequent pharmacologic advantage is quantified by determining the area-under-the-curve (AUC) ratio of the peritoneal exposure over the intravenous exposure after IP administration. In this equation, AUC IP is responsible for efficacy and AUC IV is a measure of toxicity. Until recently all pharmacologic research regarding perioperative chemotherapy was PK research.
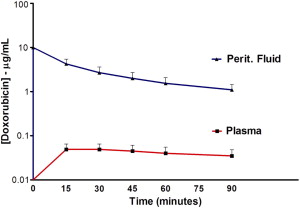
This PK strategy, however, leaves out all PD variables that are equally important for the final ability of the cancer chemotherapy drug to kill tumor cells. The basic way of depicting PD is by plotting a concentration × effect graph. However, this is not possible in vivo. The closest we can come is by adding the concentrations of the cancer chemotherapy drug inside the tumor nodule to the PK graph, as in Fig. 3 . The higher concentrations of the doxorubicin inside the tumor nodule when compared with the peritoneal levels are the result of irreversible binding at the level of the tumor cell membrane.
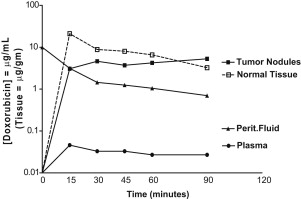
Box 1 summarizes all PK and PD variables involved in protocols for perioperative cancer chemotherapy. One could state that the PK variables influence the amount of drug showing up at the level of the tumor nodule and that the PD variables subsequently determine what goes on inside the tumor nodule. As such the tumor nodule should be considered the most appropriate end point in the pharmacologic exploration regarding these treatment strategies.
Pharmacokinetic VR
- •
Dose
- •
Volume
- •
Duration
- •
Carrier solution
- •
Pressure
- •
Molecular weight
Pharmacodynamic VR
- •
Tumor nodule size
- •
Density
- •
Vascularity
- •
Interstitial fluid pressure
- •
Binding
- •
Temperature
Timing of perioperative cancer chemotherapy in relation to timing of CRS
In the clinical application of chemotherapy in PSM patients, intervention can occur at 4 points in the timeline. First, induction intraperitoneal and/or intravenous chemotherapy is suggested as an option for reducing dissemination to the extra-abdominal space, testing the tumor biology, and for reducing the extent of small PC nodules, and theoretically this approach, called neoadjuvant intraperitoneal and systemic chemotherapy (NIPS), may facilitate definitive CRS after initial exploratory laparoscopy or laparotomy. Radiologic and clinical responses with NIPS have been reported by several groups. However, although NIPS may reduce the tumor load to be addressed by CRS, it has several disadvantages. Adhesions from prior surgical interventions may interfere with adequate intraperitoneal drug distribution and, as complete responses are unusual, further cytoreduction chemotherapy is necessary if the approach is to be curative. NIPS is reported to add to morbidity and mortality of further surgical treatment, and extensive fibrosis, as a response to chemotherapy, may occur and render judgments concerning the extent of PC difficult or impossible to assess.
HIPEC is the most widely explored modality that has shown consistent clinically improved outcomes in many phase II trials and several phase III trials.
Early postoperative intraperitoneal chemotherapy (EPIC) has some conceptual advantages. It is administered after CRS at the time of minimal residual tumor burden, and intraperitoneal treatments initiated before wound healing occurs can minimize nonuniform drug distribution and eliminate residual cancer cell entrapment in postoperative fibrin deposits. The proper selection of chemotherapy agents based on pharmacologic principles suggests the use of cell-cycle–specific drugs such as 5-fluorouracil and the taxanes. Most EPIC regimens are administered postoperatively (day 1 to day 4/5) through both an inflow catheter and outflow drains inserted at the time of CRS, and can be applied with or without HIPEC.
Several phase III trials of long-term combined intraperitoneal and systemic chemotherapy have demonstrated that intravenous plus intraperitoneal chemotherapy improves survival in patients with optimally debulked stage III ovarian cancer, compared with intravenous chemotherapy alone. This approach may be used as chemotherapeutic bridging between incomplete initial surgery and definitive cytoreduction or second-look surgery. This type of chemotherapy is an adjuvant and not a perioperative use of chemotherapy.
Failure analysis for CRS plus perioperative chemotherapy indicates that recurrent cancer occurs most frequently within the abdominal and pelvic cavity. Although systemic metastases occur, treatment failures rarely occur in liver, lungs, or other systemic sites. To optimize the treatment of patients with PC, the greatest benefit will probably result from a combination of the 4 treatment strategies.
An update on cancer chemotherapy drugs used in protocols for perioperative cancer chemotherapy
Table 1 provides an overview of drugs commonly used in protocols for perioperative cancer chemotherapy, and their main pharmacologic characteristics.
Drug | Molecular Weight (Da) | Area-Under-the-Curve Ratio |
---|---|---|
5-Fluorouracil | 130.08 | 250 |
Carboplatin | 371.25 | 10 |
Cisplatin | 300.1 | 7.8 |
Docetaxel | 861.9 | 552 |
Doxorubicin | 579.99 | 230 |
Etoposide | 588.58 | 65 |
Floxuridine | 246.2 | 75 |
Gemcitabine | 299.5 | 500 |
Irinotecan | 677.19 | No data available |
Melphalan | 305.2 | 93 |
Mitomycin C | 334.3 | 23.5 |
Mitoxantrone | 517.41 | 115–255 |
Oxaliplatin | 397.3 | 16 |
Paclitaxel | 853.9 | 1000 |
Pemetrexed | 597.49 | 40.8 |
Mitomycin C
Mitomycin C is an alkylating antibiotic extracted from Streptomyces species, whose most important mechanism of action is through DNA cross-linking. Although mitomycin C is not regarded as a prodrug, it is not active against cancerous tissue as the unchanged molecule. The drug is modified as it enters the cell into an active state. It is inactivated by microsomal enzymes in the liver and is metabolized in the spleen and kidneys. Jacquet and colleagues reported a clear pharmacokinetic advantage after IP administration with an AUC IP/IV ratio of 23.5. Mitomycin C has been extensively studied in preclinical and clinical work, and is widely used for PC ; it is used for peritoneal carcinomatosis from colorectal cancer, appendiceal cancer, ovarian cancer, gastric cancer, and diffuse malignant peritoneal mesothelioma. Barlogie and colleagues suggested in vitro thermal enhancement of mitomycin C. A recent pharmacologic analysis of 145 HIPEC patients by the authors’ group revealed that the largest proportion (62%) of the total drug administered remained in the body at 90 minutes. Jacquet and colleagues and Van Ruth and colleagues presented similar data. The location and chemical state of this large amount of retained mitomycin C remains to be determined. It is possible that active drug remains in visceral surfaces, parietal peritoneum, and preperitoneal tissues. Unfortunately, a reliable assay of tissue mitomycin C concentrations does not exist; determination of the anatomic site and anticancer activity of this large proportion of the total mitomycin C administered has not been determined. Controversies still exist regarding the proper dosimetry of the chemotherapy solution. Some institutions use a single dose of mitomycin C, others a double dose, and still others a triple dose of the drug over a 90-minute time period. A remarkable difference in drug dosimetry between different groups of investigators is reported. Van Ruth and colleagues at the Dutch Cancer Institute reported a dose-finding study. Their data suggest that a dose of 35 mg/m 2 resulted in the highest peritoneal/plasma AUC ratio with acceptable toxicity. To maintain the concentration throughout the 90 minutes of perfusion time, the dose was divided into 3 fractions: 50% at the start, 25% after 30 minutes, and 25% at 60 minutes. The toxicity profile of mitomycin C, including anastomotic dehiscence and impaired wound healing, has been well characterized.
Doxorubicin
Doxorubicin (C 27 H 29 NO 11 ) or hydroxyldaunorubicin (adriamycin) is an anthracycline antibiotic. Although traditionally categorized as a DNA-intercalating drug, the actual mechanism of action is a critical interaction of doxorubicin with the cell surface membrane as demonstrated by Tritton. Lane and colleagues reported on the temperature dependence of this phenomenon. Because of its wide in vitro and in vivo activity against a broad range of malignancies, its slow clearance from the peritoneal compartment owing to the high molecular weight of the hydrochloride salt (579.99 Da), its favorable AUC ratio of intraperitoneal to intravenous concentration times of 230, and the absence of risk for dose-limiting cardiotoxicity when used IP, doxorubicin was considered a potential beneficial agent for perioperative IP delivery. This notion was supported by both experimental and clinical PK data. A moderate temperature enhancement has been postulated. Based on ex vivo experiments, Pilati and colleagues suggested that this hyperthermic augmentation was based on increased drug uptake and sensitization of tumor cells (but not normal mucosal cells) to the cytotoxic effects of doxorubicin. More recently, PEGylated liposomal doxorubicin has generated interest for HIPEC application because of its favorable PK. Doxorubicin-based HIPEC has been used in PSM from appendiceal, gastric, ovarian, and colon cancer, as well as in peritoneal mesothelioma.
Cisplatin
Cisplatin (cis-diamminedichloroplatinum-III, CDDP) causes apoptotic cell death by formation of DNA adducts. It has been well studied in the setting of adjuvant normothermic postoperative IP chemotherapy for residual small-volume ovarian cancer after CRS. Three randomized trials showed a significant survival benefit. In the setting of CRS and HIPEC, cisplatin has been used for intracavitary therapy for ovarian cancer, gastric cancer, desmoplastic small round cell tumor, and peritoneal mesothelioma. It is eliminated by renal excretion, and consequently the main concern with its use is renal toxicity. Urano and colleagues showed an excellent in vitro and in vivo thermal augmentation of cisplatin. The penetration of cisplatin into tumor nodules was studied by several groups. Los and colleagues for the first time described intratumoral distribution of cisplatin after IP administration and suggested that the advantage over IP versus IV administration was maximal in the first 1.5 mm. Van der Vaart and colleagues investigated the cisplatin-induced DNA-adduct formation and were able to measure this 3 to 5 mm into the tumor tissue. Esquis and colleagues, in an experimental model, reported an enhanced cisplatin penetration when cisplatin was administered with increased pressure.
Carboplatin
Carboplatin ((1,1-cyclobutanedicarboxylato)platinum(II)) is a platinum compound of higher molecular weight than cisplatin. Its main advantage is its decreased renal toxicity. As such it is currently explored in normothermic IP chemotherapy protocols in patients with advanced ovarian cancer. Czejka and colleagues, in a clinical study with normothermic carboplatin, reported a relative bioavailability (calculated as AUC values) that was at least 6 times higher in the IP fluid than in the serum for 48 hours. In 1991, Los and coworkers compared carboplatin and cisplatin after IP administration in a rat model of PC. Their data demonstrated that despite a clear pharmacokinetic advantage of IP carboplatin over cisplatin; its capacity to penetrate into peritoneal cancer nodules and tumor cells is far lower than that of cisplatin. These data have limited its clinical application in the past. By contrast, a more recent direct comparison reveals a comparable or better drug penetration of IP carboplatin when compared with IP cisplatin given at equitoxic doses. This finding has recently revived clinical interest in carboplatin for IP application.
Oxaliplatin
Oxaliplatin (oxalato-1,2-diaminocyclohexane-platinum(II)) is a third-generation platinum complex with a similar cytotoxic mechanism to that of cisplatin. In contrast to cisplatin, it has proven activity in colorectal and appendiceal malignancies. Its clinical use in colorectal PSM patients was initiated by Elias and Sideris. In a dose-escalation and pharmacokinetic study, they showed that 460 mg/m 2 of oxaliplatin in 2 L/m 2 of chemotherapy solution over 30 minutes was well tolerated. The low AUC ratio is compensated by the rapid absorption of the drug into the tissue. In contrast to cisplatin and mitomycin, oxaliplatin is not stable in chloride-containing solutions, thus necessitating a dextrose-based carrier that may result in serious electrolyte disturbances and hyperglycemia during the intracavitary therapy. A recent murine pharmacokinetic study with oxaliplatin confirmed its substantial heath augmentation. In a single-institution comparative study, Votanopoulos and colleagues reported a higher hematologic toxicity with oxaliplatin-based HIPEC in comparison with mitomycin-based HIPEC. However, this was only statistically significant in the patients undergoing splenectomy as part of their CRS.
Taxanes
Paclitaxel and docetaxel are taxanes considered for IP chemotherapy. The taxanes stabilize the microtubule against depolymerization; thereby disrupting normal microtubule dynamics. These agents exert cytotoxic activity against a broad range of tumors. Because of their high molecular weight, these molecules have a remarkable high AUC ratio of 853 and 861, respectively, which translates into a clear pharmacokinetic advantage for intraperitoneal administration. The data regarding possible thermal augmentation of taxanes are conflicting. Taxanes have been used in a neoadjuvant intraperitoneal setting as well as intraoperatively and postoperatively. Their cell-cycle–specific mechanism of action makes them a particularly good candidate for repeat application such as in EPIC, NIPS, or normothermic adjuvant postoperative intraperitoneal chemotherapy. Novel formulations of taxanes aiming at an increased bioavailability are under investigation.
Pemetrexed
Pemetrexed is a multitargeted antifolate that belongs to the antimetabolites. It is an analogue of folic acid with cytotoxic activity against a variety of malignancies, especially mesothelioma, ovarian cancer, and colon cancer. Significant improvement in survival of patients with peritoneal and pleural mesothelioma after IV administration, and favorable PK, have generated interest in permetrexed’s IP application. Pemetrexed acts mainly as a thymidylate synthase inhibitor but is also unique in terms of cellular transport and lipid solubility. Pestieau and colleagues reported favorable IP PK with a 24-fold increase of peritoneal exposure after IP instillation when compared with IV administration. Pemetrexed is currently under investigation for the IP treatment of peritoneal mesothelioma and ovarian cancer.
Gemcitabine
Gemcitabine (2′,2′-difluorodeoxycitidine) is a pyrimidine analogue with a wide range of in vitro cytotoxic activity, particularly against pancreatic cancer. Pestieau and colleagues investigated the PK and tissue distribution of IP gemcitabine in a rat model. The AUC ratio (IP/IV) after IP administration was 26.8 ± 5.8, and as such was favorable for IP administration. Several investigators explored the use of normothermic IP gemcitabine in advanced cancer outside the setting of CRS. Resected advanced pancreatic cancer with high risk of recurrence in the operative field is a potential indication for intraoperative IP administration of heated gemcitabine in an adjuvant setting.
5-Fluorouracil
Since their introduction in 1957 by Heidelberger and colleagues, the fluorinated pyrimidines have been successfully used for a wide variety of tumors, and are still an essential component of all successful chemotherapy regimens to treat gastrointestinal cancer. The thymidylate synthase inhibitor 5-fluorouracil binds covalently with the enzyme and prevents the formation of thymidine monophosphate, the DNA nucleoside precursor. The action of 5-fluorouracil is therefore cell-cycle specific. Moreover, 5-fluorouracil, via its metabolites 5-fluorouridine diphosphate and 5-fluorouridine triphosphate, is incorporated in RNA, resulting in a second cytotoxic pathway. Minor augmentation of 5-fluorouracil by mild hyperthermia is reported. 5-Fluorouracil is not chemically compatible with other drugs in a mixed solution for infusion or instillation. These characteristics limit the use of 5-fluorouracil perioperatively to either EPIC or intraoperative IV 5-fluorouracil.
Ifosfamide
Ifosfamide is a prodrug that needs the cytochrome P450 system of liver or red blood cells to be activated to its active metabolite 4-hydroxyifosfamide. Consequently it requires IV administration rather than IP instillation for its cytotoxic activity. It is 1 of 4 drugs that show true heat synergy, with 5 to 10 times the duration of tumor control with 41.5°C heat in comparison with normal temperatures. It may be an ideal systemic drug to increase the cytotoxicity of hyperthermic intraperitoneal perioperative chemotherapy. Pharmacokinetic data show the presence of ifosfamide and its active metabolite in peritoneal tumor nodules after continuous IV during bidirectional intraoperative chemotherapy.
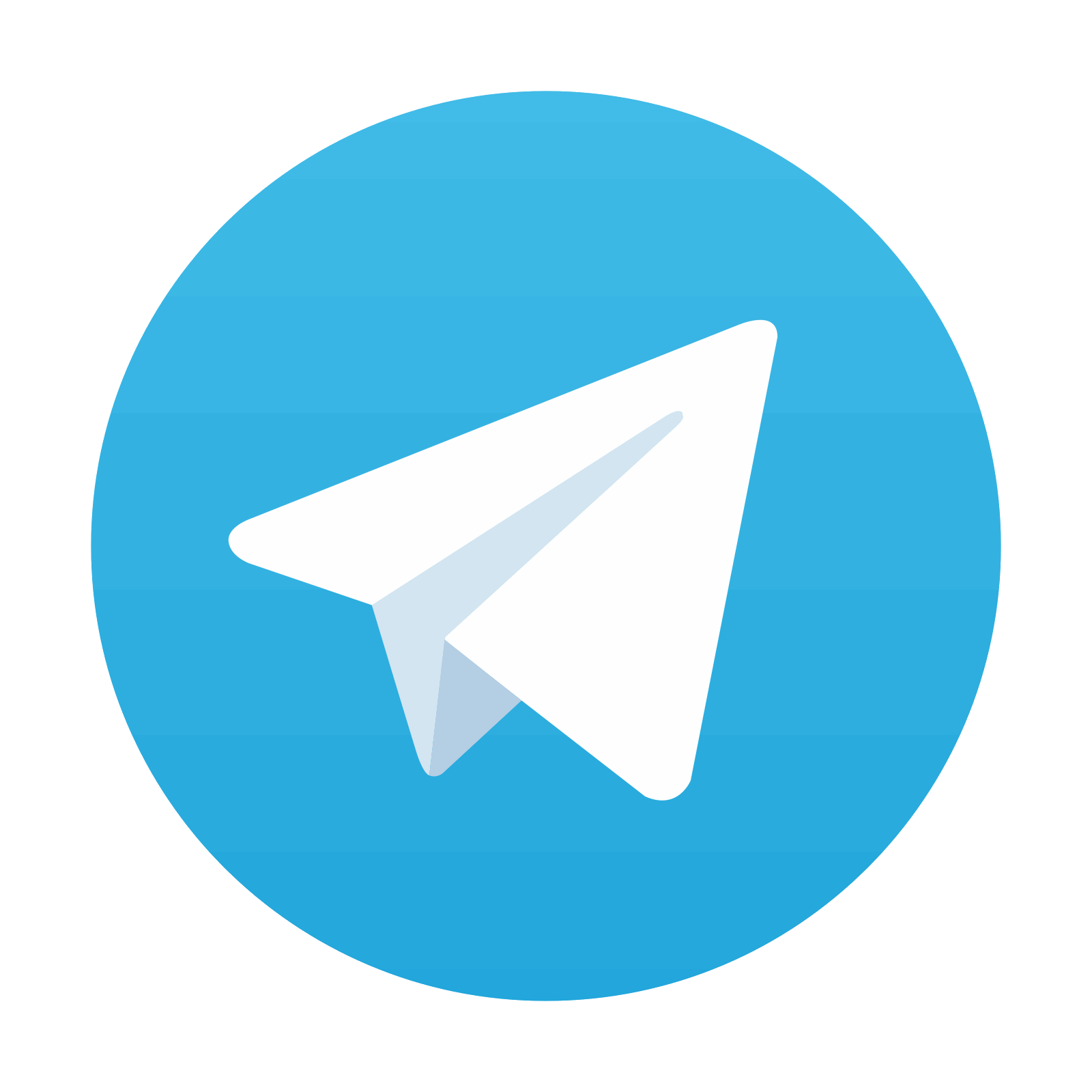
Stay updated, free articles. Join our Telegram channel

Full access? Get Clinical Tree
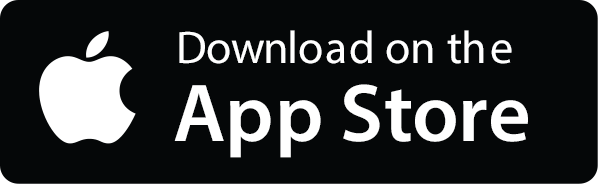
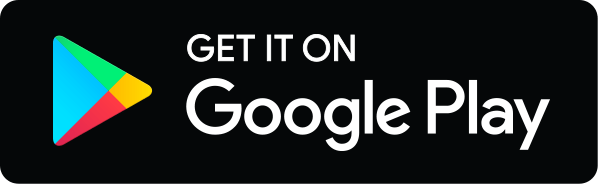