(1)
Health and Caring Professions, Technological Educational Institute of Athens, Athens, Greece
(2)
Centre for Genomics and Applied Gene Technology, Institute of Integrative Omics and Applied Biotechnology (IIOAB), Nonakuri, Purba Medinipur, West Bengal, India
Abstract
Breast cancer is the most common type of cancer and one of the leading causes of death for women. Therapeutic options for breast cancer include tamoxifen, aromatase inhibitors, fulvestrant, chemotherapy, monoclonal antibodies, tyrosine-kinase inhibitors, mTOR inhibitors, and VEGF inhibitors. Thus, endocrine therapy reduces the risk of recurrence and improves survival among women with hormone receptor-positive breast cancer. However, a significant percentage of women who receive therapy in the adjuvant or metastatic setting do not benefit from this therapy, while a number of women who respond will eventually develop disease progression and relapse while on therapy. For example, 30 % of early breast cancer patients treated with tamoxifen acquire tamoxifen resistance and relapse. The observed variability in treatment response to targeted breast cancer treatment could be partly explained by pharmacogenomics–pharmacoepigenomics, i.e., the study of genetic variation in drug response. At the nucleotide level, genetic variation is due to polymorphisms, large insertions, deletions, and duplications. Polymorphisms represent common variations in the DNA sequence that may lead to reduced activity of the encoded gene but, in some cases, to increased activities. Polymorphisms include single-nucleotide polymorphisms (SNPs), microsatellites, and mini-satellites. Pharmacoepigenetics is a novel field of research, with possible relevance in breast cancer treatment.
Epigenomics is another aspect of genetic variation that may affect drug response. The term epigenomics refers to heritable traits in the cells and organisms that do not involve changes to the underlying DNA sequence, i.e., changes in gene expression, caused commonly by environmental factors. These changes may persist through cell division and for the remainder of the organism’s life. Epigenetic processes include methylation of DNA, acetylation, phosphorylation, ubiquitylation and sumoylation of histones, histone modifications, and noncoding RNA-mediated regulation of gene expression. This chapter will review data on pharmacogenomics–pharmacoepigenomics of breast cancer treatment, focusing on clinical implications for drug efficacy and drug safety.
Keywords
Breast cancerPharmacogenomicsTamoxifenAromatase inhibitorsCYP2D6Clinical implicationsIntroduction
Adjuvant endocrine therapy, i.e., tamoxifen and aromatase inhibitors, reduces the risk of recurrence and improves survival among women with hormone receptor-positive breast cancer. Other forms of breast cancer targeted by therapy such as trastuzumab and bevacizumab contribute to the increase in survival of women suffering from breast cancer. However, a significant percentage of women who receive targeted therapy in the adjuvant or metastatic setting do not benefit from this therapy, while a number of women who respond will eventually develop disease progression and relapse while on therapy [1]. For example, about 30 % of early breast cancer patients treated with tamoxifen acquire tamoxifen resistance over the 5-year treatment period and relapse [2]. The observed variability in treatment response to targeted breast cancer treatment could be partly explained by pharmacogenomics, i.e., the study of genetic variation in drug response [3]. DNA sequence variation is due to copy-number variation, i.e., deletions and duplications of genomic DNA segments that are at least one kilobase long, single-nucleotide polymorphisms (SNPs), and microsatellite and mini-satellite repeats [4–7]. In the case of tumors, both pharmacogenomics of the host and pharmacogenomics of the tumor tissue itself could affect response to drug treatment. Pharmacogenomics–pharmacogenetics of breast cancer-targeted therapy is anticipated to contribute to personalized medicine with the choice of the appropriate drug for the appropriate patient.
Metabolism of Tamoxifen
Tamoxifen can be considered a prodrug. The parent drug has weak affinity for the estrogen receptors but undergoes excessive biotransformation catalyzed by phase I and II enzymes into active and inactive metabolites. CYP3A4 is the main CYP450 enzyme involved in the metabolism of tamoxifen to a-hydroxytamoxifen and to N-desmethyltamoxifen [8–11]. CYP2D6 is the major metabolite involved in the hydroxylation of trans-tamoxifen to trans-4-OH-tamoxifen [11]. Endoxifen and 4-hydroxytamoxifen each have at least tenfold higher affinity for estrogen receptors than tamoxifen and are associated with equivalent antiestrogenic potency [11–14]. In patients receiving chronic tamoxifen therapy, endoxifen is found in serum concentration 6- to 12-fold higher than 4-hydroxytamoxifen. Thus, in vitro and in vivo studies suggest that endoxifen is the most important metabolite required for tamoxifen treatment. However, clinical correlative studies have produced mixed results [15].
CYP2D6 Pharmacogenomics and Tamoxifen
Pharmacogenetics of CYP2D6 is expected to affect endoxifen concentrations and possibly tamoxifen-associated long-term outcomes [16]. Over 80 allelic variants and a series of subvariants of CYP2D6 have been identified and characterized [17]. They include fully functional alleles, alleles with reduced function, and nonfunctional alleles which convey a wide range of enzyme activity ranging from no activity to ultrarapid metabolism of substrates. Most inactivating mutations in CYP2D6 are point mutations resulting in splicing defects or deletions that result in a truncated protein or no protein at all [18]. Thus, adverse effects or lack of effect may occur if standard doses of a drug are applied [18–20].
The CYP2D6 activity ranges considerably within a population and includes extensive metabolizers, intermediate metabolizers, poor metabolizers, and ultrarapid metabolizers, shown in Table 25.1 [18–20]. Extensive metabolizer phenotype occurs when there is at least one functional wild-type allele at the relevant gene locus. Extensive metabolizers have normal responses to the standard doses of a particular drug [21]. Many individuals are characterized as intermediate metabolizers. Intermediate metabolizers are heterozygous for one of the inactivating mutations or homozygous for alleles associated with impaired metabolism [21]. In European populations, two alleles associated with impaired metabolism, CYP2D6*9 and CYP2D6*41, are quite common [22]. CYP2D6*9 encodes a protein with deletion of one amino acid. CYP2D6*41 includes a number of different polymorphisms including two nonsynonymous mutations that are also seen in CYP2D6*2 allele, an upstream polymorphism at position −1584 and a base substitution in intron 6. The nonsynonymous mutations do not appear to alter the enzyme activity, but the intron 6 polymorphism is associated with altered RNA splicing, resulting in lower levels of protein.
Table 25.1
CYP2D6 alleles
Normal-function alleles | Reduced-function alleles | Null-function alleles | Increased-function alleles |
---|---|---|---|
CYP2D6*2 | CYP2D6*3 | CYP2D6*8 | CYP2D6*1xN |
– | CYP2D6*4 | CYP2D6*11 | CYP2D6*2xN |
– | CYP2D6*5 | CYP2D6*12 | – |
– | CYP2D6*6 | CYP2D6*13 | – |
– | CYP2D6*9 | CYP2D6*14 | – |
– | CYP2D6*10 | CYP2D6*15 | – |
– | CYP2D6*41 | CYP2D6*16 | – |
– | – | CYP2D6*17 | – |
– | – | CYP2D6*18 | – |
– | – | CYP2D6*19 | – |
– | – | CYP2D6*20 | – |
– | – | CYP2D6*21 | – |
– | – | CYP2D6*38 | – |
– | – | CYP2D6*40 | – |
– | – | CYP2D6*42 | – |
– | – | CYP2D6*44 | – |
– | – | CYP2D6*56 | – |
– | – | CYP2D6*62 | – |
The poor metabolizer phenotype occurs when both alleles carry inactivating mutations and give rise to synthesis of enzyme with impaired activity or no synthesis of enzyme at all. About 2–10 % of the population are poor metabolizers. In Caucasians, about 8 % of the population are poor metabolizers of CYP2D6. It is estimated that 95 % of European poor metabolizers have two copies of any combination of four alleles termed CYP2D6*3, CYP2D6*4, CYP2D6*5, and CYP2D6*6, with each encoding defective forms of CYP2D6 [19]. The remaining 5 % of poor metabolizers are homozygous or heterozygous for a range of relatively rare loss-of-function alleles including CYP2D6*7, CYP2D6*8, CYP2D6*11, CYP2D6*12, CYP2D6*13, CYP2D6*14, CYP2D6*15, CYP2D6*16, CYP2D6*18, CYP2D6*19, CYP2D6*20, CYP2D6*21, CYP2D6*38, CYP2D6*40, CYP2D6*42, CYP2D6*44, CYP2D6*56, and CYP2D6*62 [19]. On the other hand, ultrarapid metabolizer phenotype, due to a gene amplification, gives rise to ultrarapid metabolism of drugs metabolized by CYP2D6. There have been reports of up to 12 copies at the same gene locus [19].
The most relevant clinical question is if CYP2D6 genotyping affects patient outcome. In that aspect, based on the abovementioned data, it was hypothesized that patients who are poor metabolizers of CYP2D6 and therefore are expected to have low plasma concentration of endoxifen might have inferior outcomes taking tamoxifen than do extensive metabolizers [23].
Is there an association between CYP2D6 polymorphisms and endoxifen plasma concentrations? Prospective cohort studies of adjuvant tamoxifen treatment have shown extensive interindividual variation in plasma concentrations of active metabolites tamoxifen and 4-hydroxytamoxifen in breast cancer patients carrying CYP2D6 polymorphisms, shown in Tables 25.2, 25.3, and 25.4 [23–37]. In particular, the patients designated as poor metabolizers show four times lower plasma concentration of endoxifen compared with extensive metabolizers.
Table 25.2
Studies demonstrating a positive association between CYP2D6 polymorphisms and treatment outcome of breast cancer patients treated with tamoxifen in the adjuvant setting
Allele | Outcome | Trial reference |
---|---|---|
CYP2D6*4 | Women with the CYP2D6*4/*4 genotype had shorter relapse-free time and worse disease-free survival compared to women with either one or no *4 alleles | [24] |
CYP2D6*4 or drug-induced impaired CYP2D6 metabolism | Patients with decreased CYP2D6 metabolism had significantly shorter time to recurrence and worse relapse-free survival | [25] |
CYP2D6*10, CYP2D6*41, CYP2D6*4, CYP2D6*5 | Tamoxifen-treated patients carrying at least one of the CYP2D6 alleles *4, *5, *10, and *41 presented with impaired formation of antiestrogenic metabolites had significantly more recurrences of breast cancer, shorter relapse-free periods, and worse event-free survival rates | [26] |
CYP2D6*3, CYP2D6*4, CYP2D6*5, and CYP2D6*41 in patients with familial breast cancer | Patients carrying impaired alleles had worse overall survival | [29] |
CYP2D6*10 | Women with the CYP2D6 *10 T/T genotype variant had worse disease-free survival | [28] |
CYP2D6*10, CYP2D6*41, CYP2D6*3, CYP2D6*4, CYP2D6*5 | The presence of 2 functional CYP2D6 alleles was associated with better clinical outcomes and the presence of nonfunctional or reduced-function alleles with worse outcomes | [29] |
Table 25.3
Studies demonstrating lack of any association between CYP2D6 polymorphisms and treatment outcome of breast cancer patients treated with tamoxifen in the adjuvant setting
Allele | Outcome | Trial reference |
---|---|---|
CYP2D6 *1, *2, *2 L, *3, *4, *5, *10B (*10), *14, *18, *21, *41, *49, *52, and *60 alleles | CYP2D6 polymorphisms were not associated with treatment outcome in the multivariate analysis | [30] |
CYP2D6*1; CYP2D6*4; CYP2D6*5; CYP2D6*6b/c; CYP2D6*9; CYP2D6*10; CYP2D6*41; CYP2D6*UM | CYP2D6*6 may affect breast cancer-specific survival in breast cancer patients treated with tamoxifen. However, no association between more frequent variants like CYP2D6*4 and breast cancer-specific survival was identified | [23] |
27 alleles via AmpliChip CYP450 | No significant effect of CYP2D6 genotyping on the risk of recurrence in early breast cancer patients treated with tamoxifen in the adjuvant setting | [31] |
CYP2D6*10, CYP2D*10, CYP2D6*41 | No association was demonstrated between any of the investigated CYP2D6 variants and disease-free survival | [32] |
9 CYP2D6 single-nucleotide polymorphisms; genotype combinations were used to categorize CYP2D6 metabolism phenotypes as poor, intermediate, and extensive | CYP2D6 phenotypes of reduced enzyme activity were not associated with worse disease control | [33] |
Table 25.4
Effect of CYP2D6 polymorphisms on tamoxifen treatment outcome: tamoxifen indications other than adjuvant breast cancer treatment
Indication | Allele | Outcome | Trial reference |
---|---|---|---|
Breast cancer chemoprevention | CYP2D6*3, CYP2D6*4, CYP2D6*5, CYP2D6*6, CYP2D6*10, CYP2D6*17, CYP2D6*41, CYP2D6*1xN, CYP2D6*1xN | No association was demonstrated between CYP2D6 polymorphisms and breast cancer occurrence | [34] |
Breast cancer chemoprevention | 33 alleles via AmpliChip CYP450 | CYP2D6 poor metabolizers showed increased risk of breast cancer occurrence. Increased efficacy of tamoxifen in patients carrying the CYP2D6*2A allele was suggested from the data of an exploratory analysis | [35] |
Metastatic breast cancer | CYP2D6*10 | Patients carrying the CYP2D6*10/CYP2D6*10 genotype had significantly shorter time to disease progression | [36] |
Metastatic breast cancer | CYP2D6*3, CYP2D6*4, CYP2D6*5, CYP2D6*6, CYP2D6*10, and CYP2D6*41 | Overall survival was significantly shorter in women with a CYP2D6 poor metabolizer phenotype | [37] |
Goetz et al. [24] were the first to show evidence that genetic variability in CYP2D6 may affect the treatment outcome of patients receiving tamoxifen. The authors performed a retrospective analysis of a prospective adjuvant tamoxifen trial (NCCTG 89-30-52) in postmenopausal women with surgically resected ER-positive breast cancer (stages I to III) to determine the role of genetic variation in CYP2D6 on patient outcome. Because of the difficulty in amplifying DNA from formalin-fixed paraffin-embedded tissue, only the CYP2D6*4 that is the most common null allele contributing to the poor metabolizer phenotype and the CYP2D6*6, an infrequent null allele, were studied. No *6 variants were detected. Women with the CYP2D6*4/*4 genotype had shorter relapse-free time and worse disease-free survival compared to women with either one or no *4 alleles. Subsequent retrospective studies verified that the CYP2D6 genotype plays a role in tamoxifen treatment outcome [25].
Schroth et al. [29] investigated the association between metabolic phenotypes of CYP2D6 and outcome of adjuvant tamoxifen treatment in estrogen receptor-positive patients suffering from early breast cancer. After a follow-up period of 9 years, it was demonstrated that the risk of recurrence was higher in patients who carried either the extensive/intermediate or the poor genotype of CYP2D6, while there was no significant difference in overall survival. CYP2D6 genotype was associated with an increase in the odds of breast cancer recurrence in the Austrian Breast and Colorectal Cancer Study Group (ABCCSG) trial, association observed only in patients receiving tamoxifen monotherapy, and not in those receiving anastrozole, an active drug not metabolized by CYP2D6, following tamoxifen.
Other investigators, focusing on the CYP2D6*4 allele, the most common poor metabolizer allele in Caucasians, have established a model using data from Goetz’s study to estimate whether women with wild-type CYP2D6 have longer disease-free survival if they receive tamoxifen rather than an aromatase inhibitor. By applying the model, the investigators proposed that women with wild-type CYP2D6 had a similar or lower rate of relapse when treated with tamoxifen compared with aromatase inhibitor treatment.
However, there are also a number of relevant negative or conflicting studies [23, 30, 38–41], with a few studies suggesting an inverse association between CYP2D6 genotype and tamoxifen response [42]. Retrospective analysis from two large randomized clinical trials (ATAC and BIG), comparing tamoxifen with aromatase inhibitors as treatment for postmenopausal women with early breast cancer in the adjuvant setting, demonstrated no association between CYP2D6 genotype and outcome in patients taking tamoxifen [43, 44]. In a well-designed case control study, Morrow et al. [31] investigated the effect of CYP2D6 polymorphisms of breast cancer recurrence. The investigators performed CYP2D6 genotyping from whole-blood and fresh frozen tumor samples using the AmpliChip CYP450 Test from patients treated with tamoxifen for early breast cancer in the adjuvant setting. Patients experiencing breast cancer recurrence were matched by date of diagnosis, menopausal status, and clinical stage to patients without recurrence. This study demonstrated no significant effect of CYP2D6 genotyping on the risk of recurrence in early breast cancer patients treated with tamoxifen in the adjuvant setting. The inconsistency can be attributed to the retrospective design of the trials, since only a few trials have used specimens collected and archived from prospective clinical trials and no trial has been designed for the investigation of the issue as a primary objective [31].
CYP2C19 and Other CYP Enzyme Polymorphisms and Tamoxifen Pharmacogenomics
A potential effect of CYP2C19 polymorphism on breast cancer risk prolapse during tamoxifen therapy has also been suggested. CYP2C9 and CYP2C19 seem to play a minor role in metabolic pathways of tamoxifen (Table 25.5). A large number of CYP2C19 polymorphisms (CYP2C19*2, CYP2C19*3, CYP2C19*4, CYP2C19*5, CYP2C19*6, CYP2C19*7, CYP2C19*8) are associated with reduced enzyme activity [45]. CYP2C19*17 is associated with ultrarapid metabolism [46]. Limited data exist on the effect of these polymorphisms on the outcome of women treated with tamoxifen, as shown in Table 25.6 [26, 33, 47, 48].
Table 25.5
Phase II metabolizing enzymes and transporters involved in tamoxifen disposition
CYP 450 enzymes | Phase II metabolizing enzymes | Drug transporters |
---|---|---|
CYP3A4 | UGT1A8 | ABCB1 |
CYP3A5 | UGT1A4 | – |
CYP2D6 | UGT1A10 | – |
CYP2C9 | UGT12B7 | – |
CYP2C19 | UGT2B15 | – |
CYP2D9 | UGT2B17 | – |
CYP2C8 | SULT1A1 | – |
Table 25.6
Effect of CYP2C19 polymorphisms on tamoxifen treatment outcome
Tamoxifen indication | Allele | Outcome | Trial reference |
---|---|---|---|
Adjuvant breast cancer treatment | CYP2C19*1, CYP2C19*2, CYP2C19*3, CYP2C19*17 | CYP2C19*7 high enzyme activity variant identified patients likely to benefit from tamoxifen | [26] |
Adjuvant breast cancer treatment | CYP2C19*2, CYP2C19*3 | The investigated genotypes were not associated with any difference in disease-free survival compared with wild-type genotype. The investigated alleles were also not associated with endometrial cancer nor with bone mineral density | [47] |
Adjuvant advanced breast cancer treatment | CYP2C19*2, CYP2C19*17 | CYP2C19*2 was independently associated with time to treatment failure. CY2C19*17 was independently associated with a longer disease-free survival | [48] |
Breast cancer chemoprophylaxis | CYP2C19*1, CYP2C19*2, CYP2C19*3, CYP2C19*17 | CYP2C19 polymorphisms did not show any correlation with tamoxifen efficacy | [35] |
In one retrospective study of 621 patients with breast cancer treated with adjuvant tamoxifen, archived specimens were analyzed for various genotypes. Among the tamoxifen-treated women, the presence of one or two CYP2C19*17 alleles was associated with more favorable relapse-free survival compared with CYP2C19*1, CYP2C19*2, and CYP2C19*3 carriers [26]. On the other hand, in a Japanese study, 173 patients with ER- and/or PR-positive breast cancer treated with adjuvant tamoxifen had peripheral blood analyzed for CYP2C19 genotype. Genotypes associated with reduced enzyme activity were not associated with any difference in disease-free survival compared with wild-type genotype [47]. Thus, currently, there is no clinical reason for testing CYP2C19 genotype in breast cancer patients treated with tamoxifen.
Other CYP enzymes involved in tamoxifen metabolism are CYP3A4 and CYP3A5. CYP3A4 is the human enzyme known to be involved in the metabolism of the largest number of medications. It has been estimated that CYP3A4 is responsible for approximately 50 % of all cytochrome P450-mediated reactions of prescribed drugs. There is interindividual variation in overall levels of activity of CYP3A4 and several polymorphisms of this gene have been described, but the allelic frequencies are low. The association of CYP3A5 polymorphisms with plasma concentrations of tamoxifen metabolites or with treatment outcome of tamoxifen therapy has also been investigated, but no clinically significant association has been identified. A CYP3A4 promoter variant has been identified, CYP3A4*1B, with no studies linking CYP3A4*1B with altered tamoxifen metabolism [1]. However, it has been reported that CYP3A4*1B confers a threefold increased risk of endometrial carcinoma in tamoxifen-treated women [49].
The most frequent and functionally relevant polymorphism in the CYP3A5 gene consists of an A6986 gene transition with intron 3 (CYP3A5*3). This polymorphism creates an alternative splice site, resulting in a frame shift and truncation of the protein [50]. CYP3A5*3 results in decreased CYP3A5 activity. However, relevant studies have shown conflicting results regarding the association of CYP3A5*3 with tamoxifen metabolism and/or breast cancer outcome [24, 51, 52]. Goetz and colleagues [24] investigated the effect of CYP3A5*3 polymorphism on outcome of tamoxifen-treated women enrolled in a North Central Cancer Treatment Group. CYP3A5*3 genotype was determined from paraffin-embedded tumor samples and buccal cells (living patients). No effect of CYP3A5*3 polymorphism was displayed on patient outcome, disease-free survival, or overall survival [24]. Tucker et al. [51] investigated whether polymorphisms in CYP3A5 were associated with altered metabolism of tamoxifen; they found no difference in tamoxifen or metabolite concentration by CYP3A5*3 status in breast cancer-treated patients [51]. However, Wegman et al. [52] in a study investigating the influence of genetic variants of CYP3A5, CYP2D6, SULT1A1, and UGT2B15 on patient outcome found that postmenopausal women homozygous for the CYP3A5*3C variant, treated with adjuvant tamoxifen, displayed significantly improved recurrence-free survival [52]. Yet, as the authors point out, this was an unexpected finding, as CYP3A5*3 genotype represents an inactive form of the enzyme. Other investigators have suggested that CYP3A5 could be a minor contributor to the overall metabolism of CYP3A5 [53]. In addition, since there is overlap in substrate specificity between CYP3A4 and CYP3A5, the contribution of each to total CYP3A activity could depend on the drug and the individual treated with this drug [25]. Current evidence, although limited, suggests that there is no clinically meaningful need in genotyping CYP3A in breast cancer patients treated with tamoxifen.
Phase II Metabolizing Enzyme and Drug Transporters, Pharmacogenomics, and Tamoxifen
Apart from CYP450-mediated pathways, other non-CYP450-mediated pathways seem to be important in terms of overall metabolism and activity profile of tamoxifen. At the level of phase II tamoxifen metabolism, sulfation and glucuronidation are the major mechanisms [54, 55].
Glucuronosyltransferases (UGTs) catalyze the glucuronidation of many lipophilic xenobiotics and endobiotics to make them more water soluble and therefore enhance their elimination. The human UGT superfamily has been classified into the UGT1 and UGT2 families, further classified into three subfamilies, UGT1A, UGT2A, and UGT2B. Overall, 30 UGT isoforms with overlapping specificities have been identified. Functional polymorphisms have been described for UGT1A1, UGT1A6, UGT1A7, UGT2B4, UGT2B7, and UGT2B15. Overall, polymorphisms of UGTs have not been investigated adequately due to the overlapping activities of the UGTs and the lack of selective probes, due to the complexity of the glucuronidation cycle and due to the difficulty of developing analytic methods to measure glucuronides [56]. Variation in drug metabolism due to altered UGT activity as a consequence of polymorphisms has been described for UGT1A1 and UGT2B7 [57–59].
It seems that the most important route of elimination of tamoxifen and its metabolites is via glucuronidation by the uridine diphosphate (UDP)–glucuronosyltransferases (UGTs), UGT1A8, 1A10, 2B7, 2B15, and 2B17 isoforms, with UGT2B7 being the most active hepatic UGT [60, 61]. UGTs catalyze the addition of glucuronide moieties to 4-OH-tamoxifen and endoxifen, which negate their antiestrogenic properties and promote their excretion [60, 61]. Tamoxifen is excreted predominantly through the bile, a process that is facilitated by tamoxifen conjugation to glucuronic acid during the glucuronidation process [62]. Missense polymorphisms have been identified in the UGTs active against metabolites of tamoxifen including nonsynonymous SNPs at codons 24 and 48 of the UGT1A4 gene, at codon 268 of the UGT2B7 gene, at codon 139 SNP of the UGT1A10 gene, and at codons 173 and 277 of the UGT1A8 gene [63].
In vitro studies suggested that UGT1A4 variant Leu48Val shows increased glucuronidation activity against tamoxifen and its metabolites. However, the clinical significance of this finding was not further explored [64]. The effect of a nonsynonymous polymorphism in UGTB215 has been investigated in breast cancer patients treated with adjuvant tamoxifen, and it was found that patients with SULT1A1*2*2 and either UGT2B15*1*2 or UGT2B15*2*2 had significantly reduced 5-year survival [65]. In addition, it has been demonstrated that O-glucuronidation of both trans-4-OH-TAM and trans-endoxifen in human liver tissue specimens was significantly associated with UGT2B7 genotype and with lower activities correlating with increasing numbers of the UGT2B7*2 (UGT2B7268268 Tyr) alleles [66]. The UGT2B7*2 allele is present in about 50 % of Caucasians and Asians.
Sulfotransferases are highly polymorphic. SULT1A1 is regarded to be the primary SULT responsible for the sulfation of 4-hydroxytamoxifen and endoxifen. Large individual variation has been demonstrated in the activity of SULT1A1 in humans. This variability has been attributed to a single-nucleotide polymorphism (G638A) in the coding region of the gene, to single-nucleotide polymorphisms in the 3-untranslated region and in the 3-flanking region of the gene, to gene deletion, and to gene duplication. The association of SULT1A1 polymorphisms with tamoxifen metabolism or with treatment outcome of tamoxifen therapy has been investigated, but the findings are discordant [67, 68].
Transporters can be classified as influx or efflux transporters, which are located either at the basolateral or apical membrane in polarized cells [69]. Efflux transporters include ATP-binding cassette transporter family (ABC) and multidrug toxin extrusion proteins (MATES) [70]. Influx transporters are the organic anion transporters (OATs and OATPs), organic cation transporters (OATPs), and oligopeptide transporters [56].
ABC transporters are classified into seven families (ABCA to ABCG) based on the nucleotide-binding domain and the transmembrane domains. There are at least 49 ABC transporter genes, but mainly ABCB1, ABCC1, ABCC2, ABCC3, and ABCG2 are involved in drug transport [71]. More than 50 SNPs and other polymorphisms affect the function of the well-characterized ABCB1 gene encoding the transporter P-glycoprotein (P-gp) [56]. Data from P-glycoprotein knockout mice have suggested that P-glycoprotein is involved in the transport of tamoxifen metabolites endoxifen and 4-hydroxytamoxifen [72]. ABCB1 polymorphisms have been reported to correlate with the treatment outcome of tamoxifen therapy [73, 74]. In addition, Kiyotani et al. [75] demonstrated that the allele of ABCC2rs3740065 has an additive effect on recurrence-free survival outcome of adjuvant tamoxifen therapy for breast cancer patients, although no association was found between this polymorphism and plasma concentrations of tamoxifen metabolites [75].
While the majority of studies focus on the effect of CYP2D6 polymorphisms on the outcome of adjuvant tamoxifen treatment, CYP2D6 polymorphisms could also affect the outcome of chemoprophylaxis with tamoxifen. In that context, in a very recently published study, a nested case control study in the context of NSABP-1 and NSABP-2 was performed to investigate the impact of CYP2D6 genotype and CYP2D6 inhibitor use, as well as metabolizer status (CYP2D6 genotype combined with CYP2D6 use), on breast cancer events. Cases were women who developed breast cancer while on tamoxifen or raloxifene chemoprophylaxis for at least 5 years and controls were women free of breast cancer. CYP2D6 genotyping was performed for alleles associated with reduced, absent, or increased enzyme activity. The authors concluded that there was no effect of CYP2D6 metabolism on the efficacy of tamoxifen or raloxifene in breast cancer chemoprophylaxis [34].
Undoubtedly, further research is needed for predicting clinical outcome to tamoxifen treatment based on pharmacogenomics. Further research efforts should focus on prediction based on genetic variations of a number of genes, breast tumor genomics, epigenetics, and micro-RNA pharmacogenomics.
Pharmacogenomics and Aromatase Inhibitors
Exemestane is a noncompetitive third-generation aromatase inhibitor used in the treatment of breast cancer in postmenopausal women. A major pathway for exemestane metabolism is the reduction of the 17-keto group by aldo-keto reductase to form 17-dihydroexemestane [76]. Existing limited data suggest that 17-dihydroxyexemestane exhibits similar anti-aromatase activity with exemestane, implying that 17-dihydroxyexemestane may significantly contribute to the anti-aromatase activity of exemestane in vivo [77]. In this regard, it has been shown that the absolute concentration of 17-dihydroxyexemestane accounts for about 10–15 % of total exemestane concentration in the plasma of patients treated with exemestane [76, 78]. According to another study, the level of 17-hydroxyexemestane accounts for 35–40 % of exemestane in the plasma of patients treated with exemestane [79].
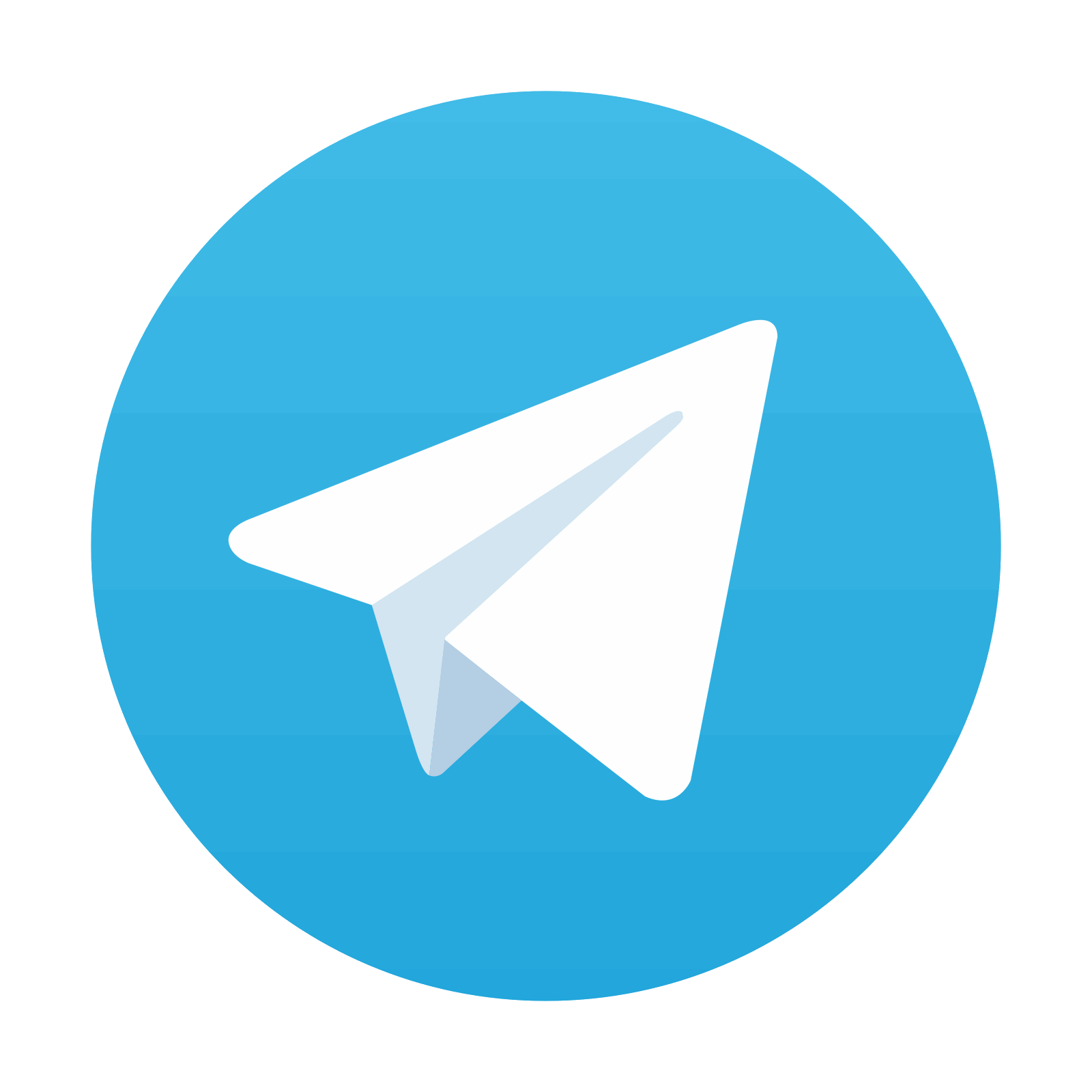
Stay updated, free articles. Join our Telegram channel

Full access? Get Clinical Tree
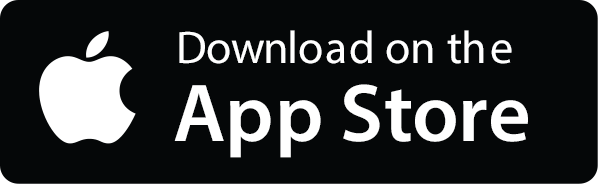
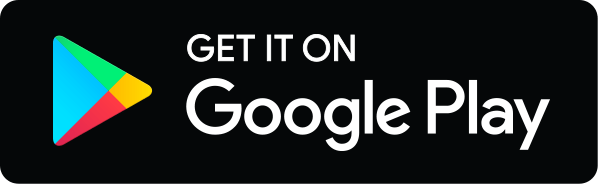