Fig. 3.1
18F-FDG-PET (transaxial section) showed increased uptake of tracers in a primary tumor of lung cancer (a) (black arrow). Immunohistochemical analysis of the resected tumor revealed that the immunohistostaining pattern of Glut1 was membranous (b). There was a statistically significant correlation between the expression of Glut1 and the SUVmax of 18F-FDG uptake in lung cancer (c) (Modified from Ref. [5, 14])
Increased glucose uptake is necessary for the survival of cancer cells, and it is thought that glucose transport plays an important role in this process [4, 5, 7, 8]. Currently, there are 14 known glucose transporter protein subtypes, and Glut1 and Glut3 have been demonstrated to be highly expressed in several human cancers [9]. In previous studies, it has been shown that expression levels of Glut1 are closely correlated with the accumulation of 18F-FDG within tumor cells [4, 5]. However, we found no significant relationship between Glut 3 expression and 18F-FDG uptake in cancer cells [4]. Recently, it has been reported that the amount of 18F-FDG uptake is determined by not only glucose metabolism, angiogenesis, and cell proliferation, but also the mammalian target of rapamycin (mTOR) signaling pathway [10]. In some studies, the uptake of 18F-FDG has been shown to be significantly linked with therapeutic response to mTOR inhibitors in cancer patients [11, 12]. Conversely, another report has documented that the amount of 18F-FDG within tumor cells is not a potential predictive marker for the response to mTOR inhibitor therapy, but a pharmacodynamic measurement for Akt activation during mTOR inhibitor treatment [13]. In our previous study, we found that the amount of 18F-FDG uptake was closely correlated with glucose metabolism (Glut1/hexokinase I), hypoxia (HIF-1α), angiogenesis (VEGF and CD34), and the PI3K/Akt/mTOR signaling pathway in patients with lung cancer [5]. Generally, the accumulation of 18F-FDG within cancer cells is known to be markedly higher in non-adenocarcinoma (AC) than in AC, but the correlation with these molecular markers was proven to be stronger in AC compared to non-AC. It has also been reported that the amount of 18F-FDG accumulation and glucose metabolism as determined by Glut1 expression has the potential to be significant prognostic markers for predicting outcome after surgical treatment in AC patients.
In experimental studies, the relationship between 18F-FDG uptake and glucose metabolism has been investigated using tumor cell lines [4, 5]. Our in vitro studies depicted that uptake of 18F-FDG was clearly decreased by inhibition of Glut1 and increased by Glut1 upregulation through the induction of HIF-1α in most cell lines [4, 5]. As it remains unclear whether 18F-FDG uptake within tumor cells is controlled by the mTOR pathway, the relationship between inhibition of the mTOR pathway and 18F-FDG uptake was investigated using lung cancer cell lines. Uptake of 18F-FDG was reduced by mTOR inhibitors in a dose-dependent manner, and the inhibition of mTORC1 alone decreased 18F-FDG accumulation in tumor cell lines. Therefore, these results suggest that the mTORC1 signaling pathway is associated with a biological mechanism for the uptake of 18F-FDG in lung cancer cells.
3.4 Clinical Role of 18F-FDG-PET as a Predictor of Outcome After Chemotherapy
18F-FDG-PET is useful not only for the diagnosis and staging of lung cancer but also for the prediction of therapeutic outcome [1, 2]. A recent meta-analysis showed that the primary tumor-standardized uptake values (SUVs) measured on 18F-FDG have prognostic value in lung cancer [3]. Many reports have investigated whether the value of maximal SUV (SUVmax) is best for use as a prognostic predictive marker after the treatment of lung cancer; however, SUV varies with several factors such as blood glucose levels and the interval between tracer injection and scanning time. Therefore, the amount of background 18F-FDG uptake is thought to be affected by significant statistical error. Recent studies have shown that it is not the value of SUVmax but rather the ratio of the SUVmax of the tumor to the mean SUV of the mediastinum (T/M ratio) that is better for measurement of the accumulation of 18F-FDG [14, 15]. However, we cannot currently conclude whether SUVmax or the T/M ratio is more appropriate to predict prognosis after chemotherapy against advanced lung cancer. Further investigation is warranted in order to discover new measurements of 18F-FDG uptake that are useful for the prediction of outcome after treatment.
For the prediction of therapeutic outcome using 18F-FDG-PET, appropriate timing of PET imaging after treatment is problematic. Many studies regarding PET in the assessment of chemotherapy focus on the early prediction of response by measuring differences between SUVmax before and after treatment. However, the relationship between initial SUV and subsequent response to chemotherapy remains unclear.
Previously, we have reported the prognostic significance of SUVmax, the T/M ratio, and the ratio of the SUVmax of the metastatic site to the SUVmax of the primary site (M/P ratio) measured on 18F-FDG-PET in patients with advanced NSCLC who had received chemotherapy [16]. Comparison of the prognostic significance of SUVmax, T/M ratio, and M/P ratio showed that high M/P ratio was a significant independent factor for predicting a shorter prognosis after chemotherapy in patients with advanced NSCLC. Although SUVmax and T/M ratio were significantly related to a worse outcome by univariate analysis, the prognosis of non-AC was unaffected by the value of SUVmax and the T/M ratio. Additionally, a high M/P ratio indicated a poor response to initial chemotherapy, but this was not true for SUVmax and T/M ratio.
It has been shown that the accumulation of 18F-FDG in preoperative primary tumors is a useful prognostic factor for predicting negative prognosis in NSCLC patients with postoperative recurrence who have received platinum-based chemotherapy [17]. The uptake of 18F-FDG within tumor cells was significantly higher in the preoperative primary site than in the recurrence site, and low 18F-FDG accumulation within the preoperative primary tumor correlated closely with the presence of epidermal growth factor receptor (EGFR) mutation. The amount of 18F-FDG uptake in preoperative primary tumors may be important for predicting outcome after chemotherapy against recurrent NSCLC as compared to that in recurrent sites. Further investigation in a large prospective study is needed to confirm this result.
In order to evaluate the early prediction of therapeutic response to EGFR-tyrosine kinase inhibitor (TKI), the usefulness of 18F-FDG-PET was investigated in advanced NSCLC harboring EGFR mutation [18]. In this preliminary study, 5 NSCLC patients with EGFR mutation underwent 18F-FDG-PET to evaluate changes in 18F-FDG accumulation at 2 days and 4 weeks after the initiation of gefitinib in comparison to 18F-FDG-PET imaging prior to treatment. The SUVmax of 18F-FDG uptake significantly decreased on day 2 after the initiation of gefitinib in patients with any response. On the other hand, the SUVmax of 18F-FDG uptake markedly increased in patients with progressive disease. This preliminary data suggests that 18F-FDG-PET could predict therapeutic response to EGFR-TKI in the early phase of therapy in patients with advanced NSCLC harboring EGFR mutation. Zander et al. compared changes in 18F-FDG uptake after 1 (early) and 6 (late) weeks of erlotinib treatment in patients with advanced NSCLC [19]. Their study stated that patients with an early metabolic 18F-FDG response showed significantly longer survival, but those with a late metabolic 18F-FDG response did not. Therefore, early response monitoring using 18F-FDG-PET may be predictive for outcome after EGFR-TKI treatment.
18F-FDG-PET has also been shown to be useful for NSCLC patients who have received concurrent platinum-based chemoradiotherapy [20]. One hundred seventy-three subjects underwent posttreatment SUV analyses, and posttreatment 18F-FDG-PET was performed at about 14 weeks after radiotherapy. High posttreatment SUV within tumor cells was related to negative survival in stage III NSCLC. Therefore, the appropriate timing of 18F-FDG-PET monitoring remains controversial, even though there are several reports on this subject.
Currently, we cannot conclude whether SUVmax as a measurement of 18F-FDG uptake is appropriate for predicting outcome after any treatment in patients with lung cancer. A recent meta-analysis reported the prognostic value of metabolic tumor volume (MTV) and total lesion glycolysis (TLG) in patients with lung cancer [21]. In 13 studies including 1581 patients, patients with high MTV or high TLG had a worse prognosis for adverse events and death, and MTV and TLG were considered to be significant prognostic factors in patients with early-stage (stage I/II) and advanced-stage (stage III/IV) NSCLC.
3.5 False-Positive Findings in 18F-FDG-PET
18F-FDG-PET can differentiate malignant tumors from benign lesions. Generally, physicians utilize 18F-FDG-PET scans to diagnose malignant disease and perform disease staging for human cancers. However, false-positive findings on 18F-FDG-PET are observed when inflammatory and granulomatous lesions such as pneumonia, pulmonary tuberculosis, aspergillus, and sarcoidosis are present [22, 23]. To overcome the disadvantage of false-positive 18F-FDG uptake in benign lesions, the optimal cutoff point of SUV has been discussed. Although an SUVmax of 2.5 has been identified as an optimal cutoff point, malignant disease cannot be diagnosed according to the value of SUVmax on 18F-FDG-PET [24].
In our institution, we developed L-[3-18F]-α-methyltyrosine (18F-FAMT) as an amino acid PET tracer [25]. 18F-FAMT-PET is useful for differentiating malignant from benign lesions, as 18F-FAMT is transported into tumor cells via L-type amino acid transporter 1 (LAT1) [26]. The LAT family consists of four subtypes: LAT1, LAT2, LAT3, and LAT4 [27, 28]. In particular, LAT1 is expressed extensively in many human neoplasms. There is no evidence of LAT1 expression in normal tissue or benign lesions. Since the uptake of 18F-FAMT is correlated closely with the expression of LAT1, 18F-FAMT is considered to be a specific PET tracer for malignant disease (Fig. 3.2). In previous studies, 18F-FAMT-PET has been described to be able to differentiate malignant nodules from sarcoid lesions [23].


Fig. 3.2
18F-FAMT-PET (transaxial section) showed increased uptake of tracers in a primary tumor of lung cancer (a) (black arrow). Immunohistochemical analysis of the resected tumor revealed that the immunohistostaining pattern of LAT1 was membranous (b). A statistically significant correlation was observed between the expression of LAT1 and the SUVmax of 18F-FAMT uptake in lung cancer (c) (Ref. [24, 26])
3.6 Development of Tumor-Specific PET Tracers
Generally, 18F-FDG-PET is used as a tool for oncologic diagnosis, but there is debate as to whether it is the best tumor-specific PET tracer since it can show false-positive findings in inflammatory and granulomatous diseases. Therefore, the development of tumor-specific PET tracers that do not show false-positive PET findings in nonmalignant lesions is important. Currently, several types of tumor PET tracers in addition to 18F-FDG-PET exist, which utilize amino acid metabolism, hypoxia, and DNA synthesis. In this section, we introduce the clinical significance of these new PET tracers.
- 1.
Amino Acid Metabolism
Tumor cells require nutrients such as glucose, amino acids, fatty acids, and vitamins in order to grow and survive. This demand is met by the availability of nutrients achieved through vascular formation and upregulation of specific transporters. Amino acids are needed not only for protein synthesis but also as a source of nitrogen and carbon for the synthesis of purine and pyrimidine nucleotides, glutathione, and amino sugars. Amino acid transporters are thought to play a crucial role in tumor cell proliferation and development. Among the several different types of amino acid transporters that exist, system L is a Na+-independent large and neutral amino acid transporter [27]. LAT1 transports large neutral amino acids such as leucine, isoleucine, valine, phenylalanine, tyrosine, tryptophan, methionine, and histidine [28]. As described above, 18F-FAMT is transported into cells by LAT1, so we believe that 18F-FAMT-PET represents an alternative method for the molecular imaging of LAT1.
Another amino acid PET tracer, 11C-labeled methionine (MET), has been used in the imaging of various human cancers [29–31] and is better for the detection of malignant tumors than 18F-FDG-PET because of its higher specificity, which improves the differentiation between malignant and benign processes. Ishimoto, et al. investigated the feasibility of 18F-FDG-PET and 11C-MET-PET for the evaluation of treatment response after stereotactic radiotherapy (SRT) in lung cancer [32]. 18F-FDG-PET and 11C-MET-PET were performed in 9 patients 1 week before and up to 8 months after SRT. The results showed that the SUV of 18F-FDG and 11C-MET uptake after SRT changed concordantly and the addition of 11C-MET-PET provided no additional information over 18F-FDG-PET. To our knowledge, there are no reports on prognosis and response after chemotherapy using 11C-MET-PET in patients with lung cancer. Additionally, PET tracers using tyrosine derivatives such as 2-18F-fluoro-L-tyrosine (18F-FET) and 3-123I-iodo-α-methyl-L-tyrosine (123I-IMT) have also been investigated [33–35]. 18F-FET-PET has been examined for its diagnostic performance in lung cancer, and the level of 18F-FET accumulation was shown to be markedly different according to histology [33, 34]. It was found that all patients with squamous cell carcinoma (SQC) showed positive 18F-FET findings, but negative 18F-FET uptake was observed in most AC patients. Therefore, 18F-FET-PET is thought to be useful in the differentiation between malignant and benign lesions in patients with SQC; however, it does not seem to be a meaningful method in AC patients. 123I-IMT single-photon emission tomography (SPECT) shows a high sensitivity for detecting primary lung cancer lesions (94 %), but for small tumors (of less than 20 mm), its sensitivity is too low to be helpful [35]. In an experimental study, 123I-IMT was found to be transported selectively via human LAT1 [36], while it has also been considered that 18F-FET may be selectively transported via LAT1, which is expressed in normal cells [34]. In human cancers, however, it remains unclear whether 11C-MET, 18F-FET, and 123I-IMT correlate closely with the expression of amino acid transporters such as LAT1. Currently, the uptake of 18F-FAMT alone has been proven to be significantly correlated with the expression level of LAT1 in patients with lung cancer.
- 2.
Hypoxic Imaging
Tumor hypoxia is associated with resistance to chemotherapy and radiotherapy and induces angiogenesis, metastasis, and tumor aggressiveness, resulting in poor prognosis. Therefore, it has a significant impact on the biological activity of various malignancies and acts as a target for tumor imaging [37, 38]. Recently, hypoxia-targeting radiopharmaceuticals have been developed for the diagnosis of malignancy and assessment of therapeutic response. 18F-fluoromisonidazole (FMISO) and 60Cu or 64Cu-diacetyl-bis (4-(N)-methylthiosemicarbazone) (ASTM) are clinically available as the principal forms of such PET tracers [39, 40]. 60Cu-ASTM-PET and 18F-FDG-PET were compared to evaluate their usefulness for therapeutic monitoring in patients with NSCLC [41]. In 14 patients, both forms of PET imaging were performed to evaluate therapeutic response. The mean T/M ratio for 60Cu-ASTM was significantly lower in responders than in nonresponders, but the mean SUV for 60Cu-ASTM was not different between responders and nonresponders. In addition, the uptake of 60Cu-ASTM did not correlate with that of 18F-FDG. This preliminary study suggests that PET imaging with 60Cu-ASTM may be effective for patients who are less likely to respond to any treatment. FMISO is a hypoxic PET tracer that is taken up selectively by hypoxic cells and shows a slower washout from normoxic cells than 60Cu-ASTM. In eight patients with advanced NSCLC, FMISO-PET and 18F-FDG-PET were assessed before and 2 weeks after chemotherapy [42]. This study suggested that changes in FMISO uptake within tumor cells measured the early response to chemotherapy and may be useful as a predictive marker related to survival. Cherk et al. described the evaluation of 17 patients with resectable NSCLC using FMISO-PET and 18F-FDG-PET [43]. In this study, the accumulation level of FMISO was significantly lower than that of 18F-FDG, and there was no correlation between either FMISO or 18F-FDG uptake and microvessel density (MVD) and HIF-1α, VEGF, or Glut1 expression, but there was a weakly positive correlation between both FMISO and 18F-FDG uptake and the proliferative marker Ki-67. However, this preliminary study had a small sample size that may have caused bias in the results. A recent meta-analysis showed that hypoxia modification could improve local control and survival after treatment [44]. Sachpekidis et al. assessed 13 patients with stage III NSCLC by PET imaging with FMISO and 18F-FDG, and their results indicated that only one subject showed increased FMISO uptake within the tumor site. A lack of correlation between FMISO and 18F-FDG implied a discordance between increased hypoxia and glycolysis in this type of cancer [45]. Currently, it seems to be difficult to utilize FMISO or 60Cu-ASTM-PET imaging in order to assess therapeutic response and prognosis after chemotherapy in NSCLC patients.
- 3.
DNA Synthesis
3′-Deoxy-3′-[18F] fluorothymidine (18F-FLT) is a thymidine analog that was developed to measure cell proliferation [46]. 18F-FLT is phosphorylated by thymidine kinase and enters the salvage signaling pathway without incorporation into DNA molecules. According to recent studies, we know that the uptake level of 18F-FLT clearly reflects tumor cell proliferation as determined by Ki-67 labeling index [47, 48]. Therefore, 18F-FLT is considered to be a more tumor-specific PET imaging marker as compared to 18F-FDG. However, during 18F-FLT-PET, this tracer normally accumulates in the liver and bone marrow; thus, malignant and normal lesions in these organs cannot be differentiated using 18F-FLT-PET. Recently, the relationship between 18F-FLT uptake and Ki-67 labeling index was investigated in 18 NSCLC patients [47]. This study showed that there was a statistically significant correlation between 18F-FLT uptake and Ki-67 labeling index (γ = 0.77; p < 0.0002) as well as between 18F-FLT uptake and 18F-FDG uptake (γ = 0.81; p < 0.0001). With regard to diagnostic performance, the sensitivity for detection of malignant lesions was 72 % for 18F-FLT-PET and 89 % for 18F-FDG-PET. Therefore, while 18F-FLT-PET may be less sensitive for disease staging compared to 18F-FDG-PET, the uptake of 18F-FLT is better for measurement of tumor proliferative activity than 18F-FDG. Other studies also support this evidence [49, 50]. Everitt et al. reported the usefulness of 18F-FLT-PET for the assessment of therapeutic monitoring after concurrent radical chemoradiotherapy in 20 patients with NSCLC [51]. 18F-FLT-PET and 18F-FDG-PET were performed at baseline, week 2, and week 4, and the SUVmax of tumor PET uptake was measured. SUVmax on 18F-FDG-PET was 14 at baseline, 10 at 2 weeks, and 10 at 4 weeks. SUVmax on 18F-FLT-PET was 6 at baseline, 3 at 2 weeks, and 2 at 4 weeks. Therefore, this study suggests that 18F-FLT-PET is a more sensitive tracer of early therapeutic response than 18F-FDG-PET. Although 18F-FLT-PET reflects different proliferative responses after treatment in NSCLC patients, it remains unclear whether it can predict outcome after therapy. Zander et al. also described that early 18F-FLT response (1 week after treatment) could predict significantly longer progression-free survival after erlotinib therapy, but 18F-FLT was not predictive for response after 6 weeks of therapy [19]. Based on these studies, 18F-FLT-PET may be a better monitoring marker for predicting early response after therapy. Further investigation in patients with lung cancer is warranted for the confirmation of these results.
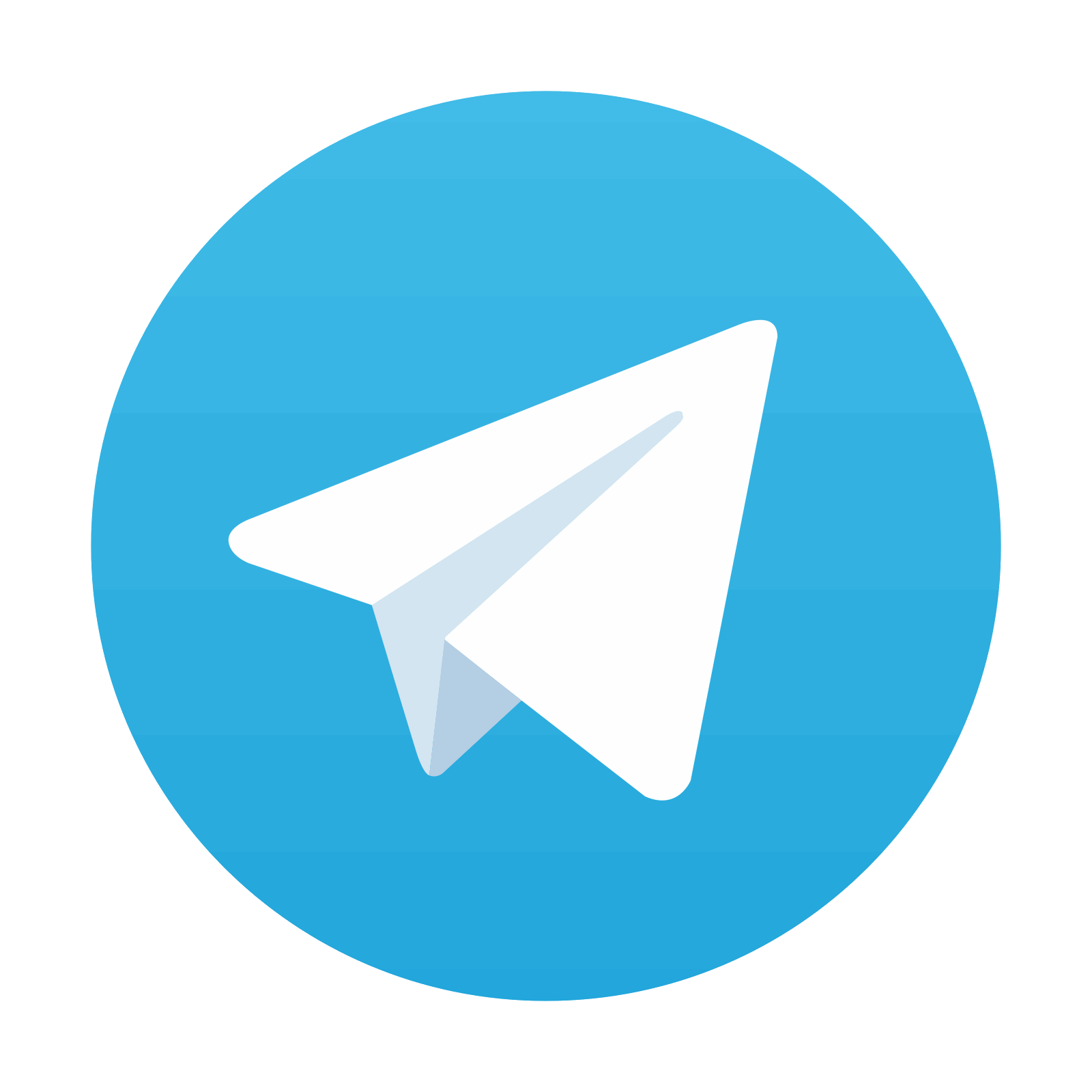
Stay updated, free articles. Join our Telegram channel

Full access? Get Clinical Tree
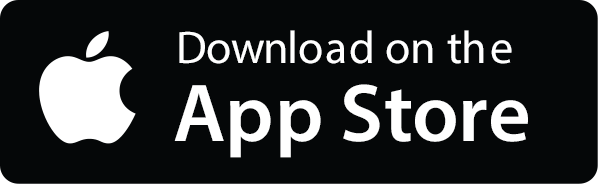
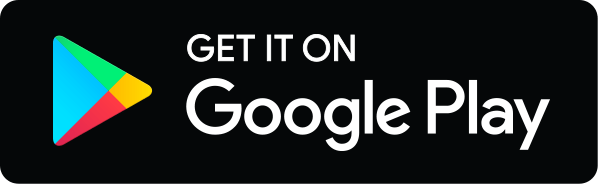