Fig. 4.1
The similarity of the robotic grasper and the human hand (copyright Intuitive Surgical International—reproduced by courtesy of the manufacturer)
Despite these advantages, bariatric surgery performed via robotic technology is still scarce, compared to regular laparoscopy. The relatively high skill set among bariatric surgeons, and the high penetration of minimally invasive surgery, seems to make the advantages of robotic system less compelling, but the growing is evident and the future is promising. Globally, the high costs associated with the purchase of a robotic system, as well as the increased per case costs, remain a key barrier to adoption of this technology. However, with the introduction of new robotic platforms, the costs of this technology will likely be reduced and further facilitate adoption, and the robotic bariatric surgery may become as common as conventional laparoscopic approach within a few years.
4.2 History and Evolution of Robotic Surgery
Since its introduction in the 1980s, several robotic systems have been developed. The first robotic device that was approved by Food and Drug Administration (FDA) for laparoscopic surgery was the AESOP (Automatic Endoscopic System for Optimal Position) by Computer Motion. This company was founded as “Dynamic Microsystems” in 1989, with support from NASA (National Aeronautics and Space Administration) and the National Science Foundation’s Small Business Innovation Research Program.
The primitive AESOP consisted of an operating table-mounted articulating arm that provided seven degrees of freedom and was used to maneuver the camera during laparoscopic surgery. This device received FDA approval in 1993 shortly after the first clinical procedures were reported. Thereafter, Computer Motion acquired additional capital to develop an entire robotic system for laparoscopic surgery, and in the mid-1990, ZEUS, a master–slave robotic surgical system, was created. The most noteworthy project involving the ZEUS robot was a dramatic demonstration of telepresence, by the performance of a transatlantic cholecystectomy with the surgeon in New York, USA, and the patient in Strasbourg, France, in early September 2001 (Marescaux et al. 2001).
At the same time frame, another robotic system was developed in Northern California, resulting in the only available system for laparoscopic surgery up to now, the da Vinci Surgical System (Intuitive Surgical, Inc., Sunnyvale, CA, USA). As its origins were military, many initial efforts on tele-robotic surgery were conducted, with aims of developing a system of remotely providing surgical care to injured soldiers in the field.
Following this initial phase, Intuitive Surgical International was founded to develop this technology, for use in civilian surgical practice. With this endeavor the emphasis shifted from telepresence to delivering a system that could facilitate minimally invasive surgical procedures, by mimicking the intuitive hand-like manipulations of open surgery, while maintaining all the benefits of the laparoscopic approach. The early prototypes were able to demonstrate the ability to develop manipulators with seven degrees of freedom that closely mimicked the movements of the human hand and wrist. This technology was developed as a master–slave system, in which there are three major components: the surgeon console (or the master), the patient cart (or the slave), and the video cart. The surgeon sits at a console remote from the patient (master), usually in the surgical suite, and performs the operation by manipulating the arms and instruments of the patient cart (slave), that is docked to the patient.
The first prototype was tested in April 1997, and in that same month, a cholecystectomy was performed, marking the first robotic surgical procedure. By December 1998, the first commercial version of the current da Vinci Surgical System was released, with intentions for use in cardiac surgery, and the first robot-assisted coronary bypass procedure was achieved shortly thereafter. Less than 2 years later, the first human trial with robotic-assisted surgery, involving 200 patients undergoing cholecystectomy and Nissen fundoplication was completed, and in July 2000, the da Vinci robotic system received FDA approval, for limited surgical use in abdominal surgeries. Further procedures were approved in the following years, for virtually all types of surgeries in different specialties.
Currently it has remained the only FDA approved robotic platform on the market, for minimally invasive abdominal and thoracic surgery. Over the last decade, the system has evolved through four major platform upgrades, from the Standard to the S System, to the Si System, and most recently to the Xi System.
These changes have resulted in improved visualization, an expansion of the operative field facilitating multi-quadrant surgery, improved ergonomics, and the addition of an array of specialized instruments for different surgical specialties, including an articulating vessel sealer and a robotically controlled stapler. In addition, starting with the Si system, other capabilities have been added in modular fashion, to support single incision surgery with the VeSPA™ surgical instruments, fluorescence imaging with the Firefly™, and data integration at the console, with a 3-dimensional surgical navigation model with TilePro™ display. Currently fluorescence imaging and use of 3-dimensional reconstruction of computed tomography images are already being used in limited settings, to facilitate identification of anatomical structures and to “preplan” surgical approaches. Nevertheless, the potential of the digital interface between surgeon and patient is still in its infancy (Kim et al. 2013).
4.3 The Da Vinci Surgical System
The da Vinci robot is a master–slave system, whereby the surgeon sits at the console (master) and performs the procedure by manipulating controllers that translate the movement into tissue manipulation through the surgical cart (slave), that is docked to the patient. The system has three major components: the surgeon console, the surgical cart, and the vision cart (Fig. 4.2).
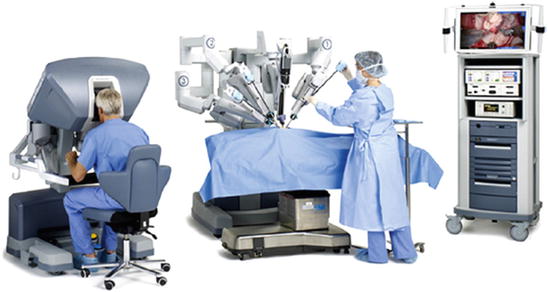
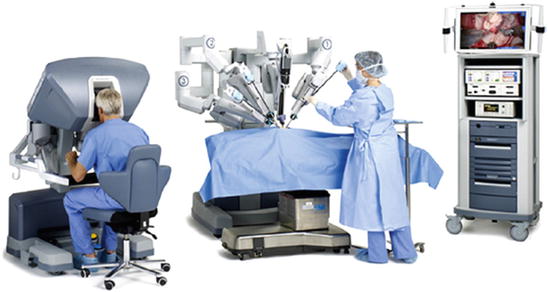
Fig. 4.2
Da Vinci robotic systems have three major components: the surgeon console, the surgical cart, and the vision cart (copyright Intuitive Surgical International—reproduced by courtesy of the manufacturer)
The shared core technology of the four versions offers the following distinguished features: physical separation of the surgeon from the patient through telepresence; a 3-dimensional stereoscopic image (HD for the S and Si model), with up to ten times magnification; wristed movements of the robotic instruments providing seven degrees of freedom (compared with five degrees of freedom for standard laparoscopic instruments); anthropomorphic design of the surgical arm and instruments for intuitive control; and software features such as tremor filtration and optional motion scaling up to 3:1.
The surgeon console allows the surgeon to control up to four robotic arms, including the videoscope, in a very ergonomically friendly manner (Figs. 4.3, 4.4, and 4.5). The system is designed to offer hand–eye alignment, which means that the endowrist instruments move in the same way with respect to the camera, as the hands of the surgeon move with respect to the surgeon’s eye. The instrument tips automatically align with the controllers at the surgeon console, and their movements mimic the movements of surgeon’s hand. These features establish a strong sense of hand–eye coordination, and natural intuitive motion, promulgating the illusion that the robotic instruments are his/her fingers. These advantages, together with the 3D vision, mimic the feel of open surgery, with the sense of actually being within the operative field.
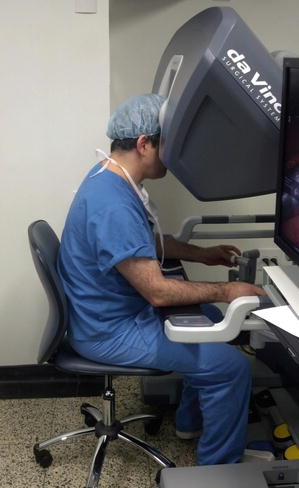
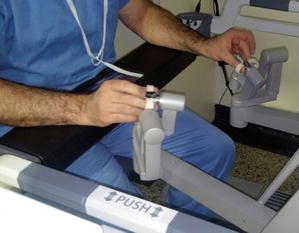
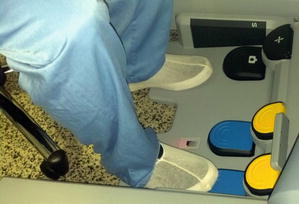
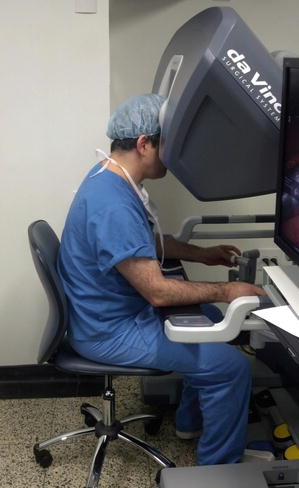
Fig. 4.3
Ergonomic advantages of robotic surgery
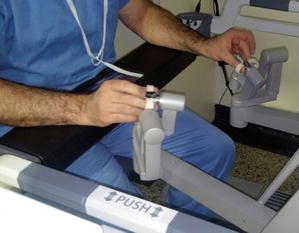
Fig. 4.4
The surgeon controlling the robotic arms through the surgeon console
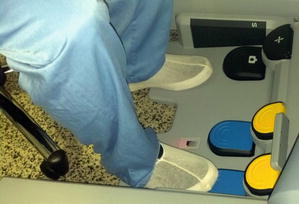
Fig. 4.5
Control by pedals at the surgeon robotic console
As was intended, these key features of the robotic system significantly enhance dexterity, when compared to traditional laparoscopy, and enable minimally invasive surgery. This impact was quickly recognized in urology. Although cardiac surgery was the first intended target for introduction of the robotic system, urologic surgery, and specifically prostatectomy, was the first area that saw widespread adoption of the robotic technology. It quickly evolved from a predominantly open procedure to a minimally invasive robotic procedure.
4.4 The Learning Curve and Training Program
Advances in surgical technology are often associated with a learning curve, before the surgeon becomes proficient, and truly learns how to use the innovation. This is certainly the case with the robotic platform, with the caveats that the learning curve also involves the surgical team, instead of just the surgeon. In many instances the surgeon is learning a new technique, concurrently with learning to use the robot.
The surgeons to be trained can be divided into three groups: those who are really novices in surgical practice; those who are surgeons, but do not master the laparoscopic approach; and finally those who already have training in laparoscopy. Each in their own way, all of these “levels” of surgeons have to go through the robotic learning curve.
The learning curve is typically defined by stabilization of operative times, and/or the achievement of certain clinical benchmarks. It can be described as having three components: the starting point, the slope of the curve, and the plateau of the curve. The starting point may be variable, since each physician has a previous individual experience that conducts to different initial levels of expertise. The slope of the curve represents how fast the surgeon learns the needed new skills, and varies by procedure, by surgeon, and by how many and how often the new procedure is performed. Finally, the plateau is when the changes in the outcomes being measured are not significant, the surgeon is usually deemed trained, and he may reach the end of the learning curve [18].
The appropriate way of analyzing the learning curve is an important topic of debate, and there has been a wide variation in the endpoints used; however, a comprehensive review of the literature indicated that the “duration of the procedure” is the most commonly used method, for establishing the learning curve in robotic surgery (Harrysson et al. 2014). Meanwhile, it seems that the most appropriate way of defining the learning curve process is the association of the operative time, number of conversions, nature of the results, and complication rate.
Based just on operative time, the initial studies suggested the learning curve of the robotic RYGB to be around 15–30 cases. Additionally, stabilization of operative time appears to occur at a lower number of cases, in centers focusing the use of the robotic platform, with high volume and a dedicated robotic team and program (Mohr et al. 2005; Ayloo et al. 2011, 2014; Vilallonga et al. 2012). This represents a significant decrease when compared to the learning curve of laparoscopic RYGB, which is believed to be around 100 procedures (Oliak et al. 2003; Schauer et al. 2003; Zevin et al. 2012).
A prospectively randomized trial, which compared fellows naive to both robotic and laparoscopic RYGB, found significantly shorter operating times per BMI during the robotic learning curve, when compared to the laparoscopic procedure (Sanchez et al. 2005). On the other hand, when taking into account clinical variables such as conversions, postoperative morbidity, and length of hospital stay, the learning curve for a totally robotic RYGB approaches more closely that of the laparoscopic RYGB (Renaud et al. 2013).
After undertaking the required training, it would be wise to arrange any proof or certification. Currently there is no surgical society mandated or even suggested criteria, for privileging or certifying the use of the robotic platform, and most hospital systems have adopted their own criteria. In the coming years, it would be desirable to establish global parameters for certification, based on scientific and practical qualifications of surgeons.
4.5 MIMIC Simulator and Da Vinci Technology Training Path
Classically, the surgical training begins with theoretical knowledge, goes to dry training models in basic or electronic simulators, reaches animal models and assisted-training field, and proctored surgeries, with the tutor observing the first sequence of operations. Finally the surgeon could be considered able to start his/her own series, based on the good performance in the previous steps. The robotic platform training phase in animal models was replaced by simulators, allowing the creation of specific situations of surgical reality, which may accelerate the training and acquisition of surgical skills, using models quite similar to airline pilot graduation programs.
Nowadays, simulators are very requested in robotic surgery training. The Mimic® robotic simulator was created to introduce virtual surgery, as a valid tool for medical training. This organization has been working with Intuitive Surgical to integrate simulation software with the da Vinci Si surgeon console (dV-Trainer, Mimic Technologies, Seattle, WA) (Mimic Technologies 2014). The quality of surgical training is improved with the program, by the simulation of surgical reality in a progressively difficult, step-by-step planed strategy of surgical training.
The da Vinci System manufacturer provides an oriented program, the “da Vinci Technology Training Pathway”, which intends to train, and not to substitute formal medical surgical training or certification. The goal of this team-oriented program is to help surgeons and staff to develop the knowledge and skills needed to use the technology safely and efficiently. It focuses several steps: system knowledge/skill development and peer-to-peer education.
The “system knowledge and skill development” consists in understanding the da Vinci Surgical System and learning technical skills that will be used in the operating room. The “peer-to-peer education” is a surgeon-to-surgeon instruction and mentorship program that is conducted by qualified, independent medical professionals. Both are fundamental to better practice in robotic surgery.
The first phase (Introduction) is designed to give a foundational understanding of the system. The second one (Technology training) introduces surgeons to the core of the technology, where the surgical teams complete online product training modules, view full-length procedure videos, attend hands on training sessions at a local hospital, and a full day of training at a da Vinci Training Center. The third phase consists in initial case series plan (Skills application). The surgeons are assisted by experienced proctoring surgeons during their first procedures, providing direct support and help in ensuring proper technique, showing the best way for doing the different steps of the procedures, and resolving eventual difficulties in order to keep the safety for the patient. The last phase is a continuing development (also Skills application) conducted by experienced, independent surgeons, who contract with Intuitive Surgical to offer multiple specialties training through select centers. The surgeon should be supervised until he is deemed competent.
Following the initial case series, post-case debriefing is held for the entire team. The team reviews the results with an eye toward benchmarking and improving clinical performance. Surgeons are very encouraged to engage in additional skills simulator and skills drill practice, which are designed to optimize intraoperative dexterity, and to revise the videos of the surgeries, trying to understand what could be done better in the next ones (Intuitive Surgical 2014).
4.6 Robotics in Bariatric Surgery
The bariatric surgery population presents unique anatomical challenges that influence the complexity of the surgical procedure, including a thick abdominal wall, larger liver, and excessive intra-abdominal fat. Additionally RYGB, the most commonly performed bariatric procedure in the United States, involves two bowel anastomoses, requiring intracorporeal suturing.
The robotic system, with high definition, magnified, 3-dimensional vision, seven degrees of freedom, scaling of motion and tremor filtration, ergonomic advantages, and, most importantly, anthropomorphic design of the system intended to mimic those of the human hand, helps overcome the added challenges of performing these procedures in a minimally invasive fashion, and presumably facilitates a more predictable and safer outcome.
One of the most significant differences between robotic surgery and conventional laparoscopy is that with robotic surgery a computer, or digital interface, exists between the surgeon and the patient. This allows a limitless potential for data use, particularly imaging, that can be collected both preoperatively and in real time, and manipulated and integrated with the surgical anatomy, to help identify critical anatomical structures.
Since the first robotic bariatric procedure, placement of an adjustable gastric band was performed in 1998 (Cadiere et al. 1999) by using the Mona, an early version of the current da Vinci System; almost all bariatric surgical procedures have been performed to varying degrees using the robotic system. Systematic reviews had already established the safety and feasible of the robotic approach in all of them (Gill et al. 2011; Cirocchi et al. 2013).
RYGB is considered in many countries the gold standard of surgical treatment of morbid obesity. The introduction of robotics technology in RYGB began with a hybrid method (Horgan and Vanuno 2001; Talamini et al. 2003), called “robotic-assisted.” In this technique the operation starts as a conventional laparoscopy, and the robot is docked to the patient only to perform a specific step, usually the handsewn gastrojejunostomy, followed by the same conventional laparoscopy to finish the procedure.
After the initial experience, the robot was docked to the patient from beginning to end of the procedure, featuring the totally robotic bariatric surgery (Mohr et al. 2005, 2006; Sanchez et al. 2005). Although this is called “totally robotic,” still today there is need for an assistant surgeon to fire the stapler by an auxiliary trocar. The recent FDA approval for the staplers coupled to robotic arms could make the procedure totally robotic soon.
In some cases, the hybrid technique could represent a preliminary step toward performing a totally robotic RYGB, a sort of “staff training” in managing the technology, especially the time when the robot is attached and unattached to the patient, called “docking and undocking time.” Although these may seem responsible for greater operative time observed in robotic RYGB, they have a tendency to become shorter along with experience, not more than 5–10 min for skilled groups.
Although it is difficult to completely capture the whole financial impact of one technique versus the other, cost is perhaps the greatest barrier to the adoption of robotic technology over conventional laparoscopy. The capital cost of the robotic system (approximately U$ 2,000,000) has to be added to an annual maintenance fee of about 10 % of the purchase price and to the costs of disposable items. Both the procedural cost and the total cost of performing robotic RYGB have been found to be greater than for the laparoscopic technique (Hubens et al. 2008; Curet et al. 2009; Scozzari et al. 2011).
On the order hand when the costs of supplies, hospital stay, and complications for the robotic approach were compared to open and laparoscopic technique, the total cost could be similar or even less. Factors that contributed to increased costs for the open and even laparoscopic techniques were length of hospital stay, time in the intensive care unit, and complication rates including anastomotic leaks. In addition, the robotic RYGB was able to report significant savings with regard to instrumentation costs by avoiding the use of expensive laparoscopic staplers and replacing by laparoscopic suturing (Hagen et al. 2012).
Thus, it is expected that in a short period of time, the cost will be no longer a barrier to the widespread adoption of robotic technology in bariatric surgery.
4.7 The Robotic Roux-en-Y Gastric Bypass
While worldwide experience in robotic RYGB has involved short follow-up to date, results about weight loss and complication rates are similar to laparoscopic RYGB (Ayloo et al. 2011), even in a large series with over a thousand patients (Tieu et al. 2013). On the other hand, robotic RYGB has shown higher total operative time (Myers et al. 2013), and a greater overall cost of the procedure. With increasing experience of the groups, both cost and surgical time tend to decrease, and reach the laparoscopic results.
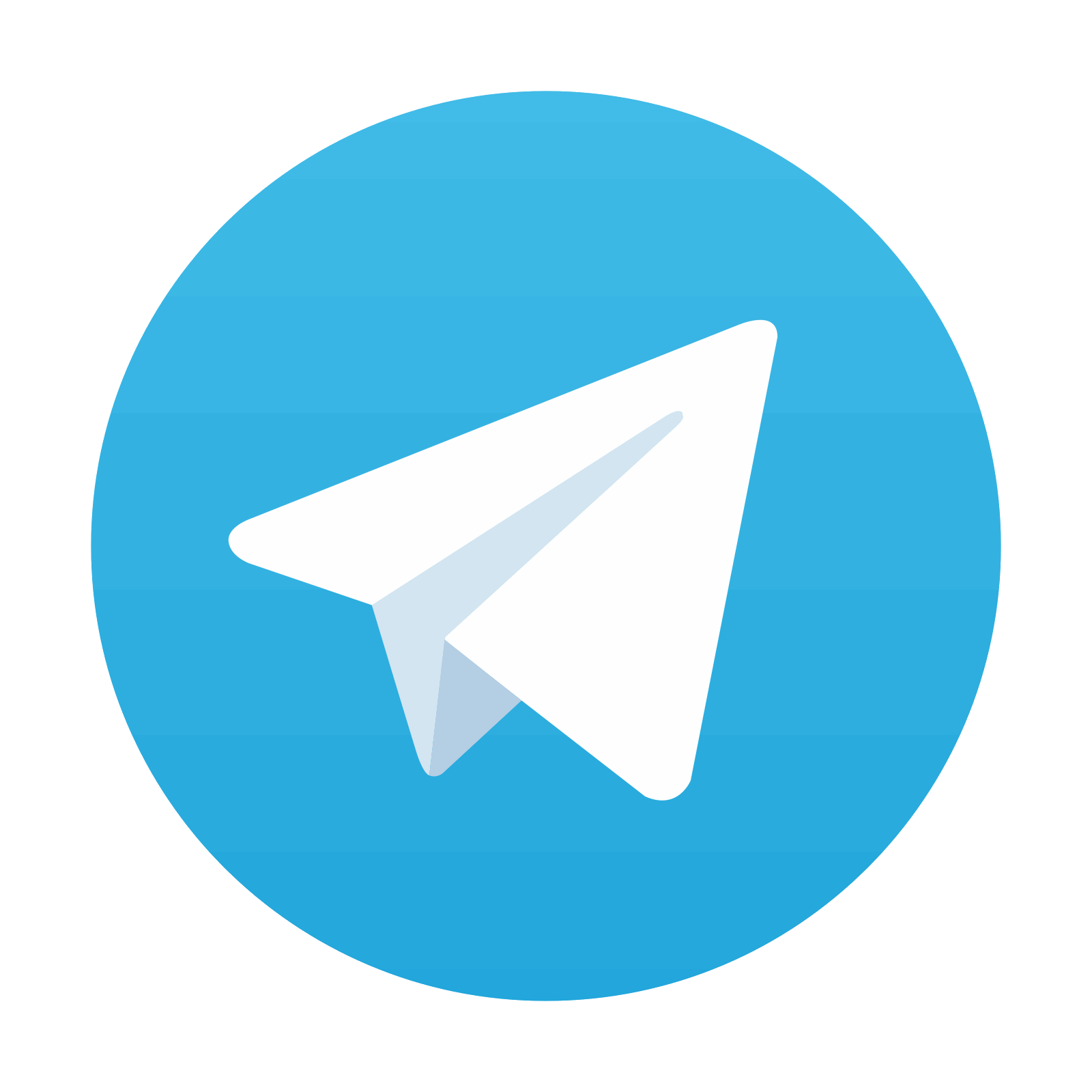
Stay updated, free articles. Join our Telegram channel

Full access? Get Clinical Tree
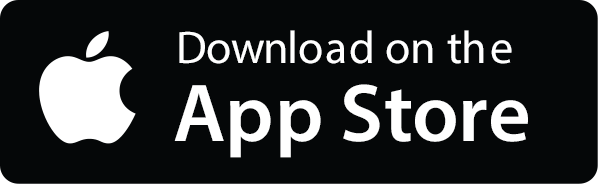
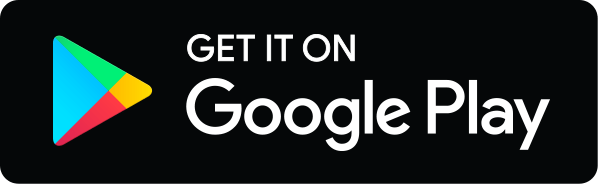