Fig. 17.1
Axial T1 fat-suppressed images before (a) and after (b) the administration of intravenous contrast in a woman with extremely dense fibroglandular tissue and marked background parenchymal enhancement on the first post-contrast image (b)
Computer algorithms are also being developed to provide a quantitative metric of background parenchymal enhancement [12, 13]. From a computing standpoint, this is more complicated then the volumetric assessment of fibroglandular tissue because it involves the evaluation of contrast enhancement, which itself is dependent on a number of different factors. A detailed discussion is beyond the scope of this chapter, but variables include time, type of contrast and dose. Robust and standard quantitative methods are needed but do not currently exist.
There has been current investigation into whether background parenchymal enhancement in the healthy breast can be predictive or prognostic of the breast cancer in the contralateral breast. In a recent study, authors reported that neoadjuvant chemotherapy patients with higher pre-treatment background parenchymal enhancement in the contralateral normal breasts went on to show a significant decrease of background parenchymal enhancement early after neoadjuvant chemotherapy and were more likely to achieve pathologic complete response [14]. Another study evaluated the parenchymal enhancement in the contralateral breast in patients with unilateral breast cancer; the results suggested that parenchymal enhancement was significantly associated with long-term outcome [15].
No studies to date have employed the power of more than a few parameters together to explore background parenchymal enhancement as a predictive biomarker, nor have they tried to quantify it on a voxel-by-voxel basis. Background parenchymal enhancement should be further utilized by exploring its potential as a predictive biomarker through the means of multiparametric MRI and advanced image analysis.
Analysis of information inherent in breast MRI images such as fibroglandular tissue and background parenchymal enhancement may ultimately lead to the development of risk-adjusted screening algorithms. Understanding the impact of these entities for risk has the potential to obviate the current blanket recommendation for a yearly screening mammogram beginning at the age of 40 as recommended by the American College of Radiology [16] and the Society of Breast Imaging [17]. Larger studies are needed to investigate and support this possibility.
17.3 Breast Cancer Genetics
Our current understanding of breast cancer genetics continues to evolve rapidly. As researchers increasingly perform next generation DNA and RNA sequencing, our understanding of breast cancers will continue to grow and the currently defined molecular subtypes described below will likely become further subcategorized in the next few years.
Breast cancer is a heterogeneous disease. The discovery of different molecular subtypes based upon genetic variation has markedly impacted the treatment of breast cancer and helped develop the concept of personalized clinical care [18]. These subtypes have been shown to be predictive of disease-free and overall survival [19] and are used to guide targeted therapy. Full genome sequencing of all diagnosed breast cancers is not yet a clinical reality, although it is possible for a price. Therefore, immunohistochemical surrogates, specifically estrogen receptor (ER), progesterone receptor (PR) and human epidermal growth factor receptor 2 (HER2) status, are used to approximate the following molecular subtypes: 1- luminal A (ER positive (+) and/or PR+, HER2 negative (−)), 2- luminal B (ER+ and/or PR+, HER2 +), 3- HER2-over-expressing (ER-, PR- and HER2+) and 4- basal-like/triple negative (ER-, PR- and HER2-) (Fig. 17.2). The luminal A subtype is the most common and is significantly associated with the best disease-free and overall survival compared to luminal B, HER2-overexpressing and basal-like subtypes. HER2-overexpressing and basal-like subtypes have the worst outcome. However, Herceptin has significantly improved the disease free and overall survival of HER-2-overexpressing cancers [19].


Fig. 17.2
Axial T1 fat-suppressed post-contrast images of (a) Estrogen Receptor positive HER2 negative (b) HER2 overexpressing and (c) Triple negative breast cancers (arrows). Research is currently evaluating if the MR imaging phenotype correlates with the molecular subtype
In addition, within each molecular subtype, it is becoming increasingly clear that there is genotypic variation between tumors (inter-tumoral heterogeneity). This knowledge has resulted in the development and clinical use of predictive and prognostic assays. For example, the American Society of Clinical Oncology has recommended the clinical use of Oncotype Dx (Genomic Health, Redwood City, CA) in early-stage ER+/HER2− invasive breast cancers because it has been shown to be both predictive (likelihood of recurrence) and prognostic (magnitude of chemotherapy benefit). Oncotype Dx is a clinically validated, gene-expression based prognostic and predictive assay that incorporates the mRNA expression of 21 genes, resulting in the so-called Recurrence Score. This Recurrence Score quantifies the magnitude of chemotherapy benefit as well as the 10-year risk of distant recurrence, both increasing with higher Recurrence Scores [20]. This Recurrence Score significantly impacts treatment decisions; women with low recurrence scores can avoid unnecessary chemotherapy [21]. Mammaprint (Agendia) and PAM50 (Prosigna) are other predictive and prognostic genomic assays, which are both clinically available.
Breast MRI is the most sensitive imaging modality for tumor characterization and accurate size measurement at present. There have been major technological advances in MRI which complement the above-described discoveries of the genetic heterogeneity of breast cancer. Advances in MRI include the now clinically available 3-Tesla MRI scanners as well as 8 and 16-channel breast coils. High temporal and spatial resolution imaging is also now a clinical reality. Isotropic MR imaging, which means the voxel is equal in three dimensions allows for multiplanar reconstruction. Clinical indications for breast MRI include pre-operative evaluation to define extent of disease in newly diagnosed breast cancer [5, 22]. Clinically, tumors are still characterized by using the American College of Radiology Breast Imaging-Reporting and Data System (BI-RADS) Lexicon. Besides tumor size measurement, BI-RADS characteristics are qualitative assessments of tumor features or semi-quantitative analyses of contrast enhancement kinetics.
One consequence of technological advances in imaging is that a large volume of data exists. Data mining (also referred to in the literature as data or knowledge discovery) involves computer extraction and analysis of data with the goal of discovering useful information. As it pertains to the field of radiology, this type of computer analytics is referred to as ‘radiomics’. Radiomics involves the computer extraction and analysis of quantitative imaging features [23]. One example of this is in the characterization of tumor morphology, texture and enhancement kinetics [24]. This data can be used to build models, which could be used to predict tumor type, behavior or response. Radiomics enables the correlation of imaging phenotypes with genomic information; this is called radiogenomics [25].
Currently the benefit of radiomics and radiogenomics is the identification of tumor imaging biomarkers. As explained earlier in this chapter, an imaging biomarker is a broad term implying either a biologic feature or indicator of biologic behavior that is detectable on an image. Biomarkers are either qualitative or quantitative. A quantifiable imaging biomarker is one that can be objectively measured, which is preferable because it eliminates inter-reader variability and allows change to be assessed on follow-up scans. This is particularly relevant to those patients undergoing treatment. A simple example of a quantifiable imaging biomarker is tumor size. The types of biomarkers that are being researched are broad but include subtle imaging changes, often imperceptible to the eye (features that can be generated by sophisticated computer algorithms) as they pertain to biology, physiology and treatment response, functioning as surrogate markers of tumor behavior and/or endpoints.
Research in this area includes the development of predictive models that correlate breast cancer MRI features with breast cancer molecular subtypes [26–28]. For example, machine-learning-based (support vector machines) models using leave-one-out cross validation have been used to identify significant MR image features associated with three different invasive ductal carcinoma molecular subtypes (ERPR+ (n = 95, 53.4 %); ERPR−/HER2+ (n = 35, 19.6 %); triple negative (n = 48, 27.0 %)). This study developed a predictive model that could distinguish the breast cancer (n = 178) with significant predictive power. When combining the top nine imaging features, the model distinguished the subtypes with an overall accuracy of 71.2 % [26].
Breast cancer MRI features are also being correlated with clinically available genomic assays [29, 30]. MR morphologic and texture-based image features of ER+, PR+ and HER2− invasive ductal carcinomas have been extracted to investigate their association with Oncotype Dx. Ninty-five patients were included with a median Oncotype Dx Recurrence Score of 16 (range: 0–45). Using stepwise multiple linear regression, a model was developed using imaging and pathology information that correlated with the Oncotype Dx recurrence score [30]. Additionally, MR imaging radiomics of 108 breast cancers from The Cancer Imaging Archive (TCIA; (http://www.cancerimagingarchive.net)) have been correlated with their respective genomic analyses in The Cancer Genome Atlas (TCGA)—both of which are open-source and open-access information resources [31]. This study also demonstrated significant associations between breast cancer MR radiomics signatures and multigene assay recurrence scores, specifically Mammaprint (Agendia), Oncotype Dx and PAM50 risk of relapse based on subtype (Prosigna) [29].
Currently, researchers are using different computer algorithms to extract MR imaging phenotypes. Large scale datasets are necessary to validate all observations are needed and this will be facilitated by multi-institutional collaboration. This will allow researchers to test their results models on MR images acquired by different vendors, magnet strength and imaging protocols.
17.4 Therapy
As stated above, breast cancer encompasses a heterogeneous group of tumors; this heterogeneity results in very different outcomes. Targeted systemic therapy, based upon a tumor’s molecular subtype, epitomizes the concept of precision medicine. Breast cancer systemic recurrence remains a major cause of morbidity and mortality [32]. Historically, chemotherapy was administered after tumor surgical resection and was termed adjuvant chemotherapy. Recently, research has demonstrated equivalent success of adjuvant and neoadjuvant chemotherapy in preventing breast cancer local recurrence. Neoadjuvant chemotherapy (NAC) is used to treat breast cancer pre-operatively and historically it was used for patients with inflammatory or inoperable locally advanced breast cancer. A major shift in breast cancer treatment is currently taking place whereby NAC is being increasingly used because it enables more patients to be treated with breast-conserving surgery whereas traditionally they would have required a mastectomy [32, 33]. NAC is now being increasingly used to establish tumor chemosensitivity in vivo with the goal of a pathologic complete response [34]. Pathologic complete response, an intermediate endpoint, serves as a biomarker for improved disease free and overall survival [34]. The focus in this section will be on the use of NAC since MRI plays a critical role in operable breast cancer. Discussion of all oncologic treatments exceeds the breadth of this chapter.
A study in 2006 reported that approximately 70 % of patients responded to NAC, with pathologic complete response (pCR) in 13–26 % [35]; however, more recent studies suggest the pCR rate is higher [36, 37]. Breast cancer genetics plays a significant role: it has been shown that the likelihood of a tumor responding to NAC is based upon its specific subtype, with triple negative and HER2 over-expressing tumors having a higher likelihood of complete pathologic response (pCR), of up to 38.2 % and 45.4 %, respectively (Fig. 17.3, 17.4, and 17.5) [36, 37]. Currently, no imaging biomarker has been validated to detect a pathologic complete response post NAC preoperatively. Therefore, all patients still undergo breast surgery post NAC.




Fig. 17.3
HER2 overexpressing right breast cancer axial T1 fat-suppressed post-contrast image pre (a) and post (b) neoadjuvant chemotherapy (arrows). This case was interpreted as a complete radiologic response because of the absence of any residual enhancement on the post-neoadjuvant chemotherapy images (b). Surgical excision confirmed a complete pathologic response

Fig. 17.4
Triple negative right breast right breast cancer axial T1 fat-suppressed post-contrast image pre (a) and post (b) neoadjuvant chemotherapy (arrows). This case was interpreted as a partial radiologic response because there has been a decrease in size of the persistently enhancing mass on the post-neoadjuvant chemotherapy images (b). Surgical excision confirmed a partial pathologic response

Fig. 17.5
Estrogen receptor positive HER2 negative left breast cancer axial T1 fat-suppressed post-contrast image pre (a) and post (b) neoadjuvant chemotherapy (arrows). This case was interpreted as a partial radiologic response because there has been a decrease in size of the persistently enhancing mass on the post-neoadjuvant chemotherapy images (b). Surgical excision confirmed a partial pathologic response
As our understanding of breast cancer genetic subtypes expands, so too the availability of novel therapies will increase. The National Comprehensive Cancer Network guidelines currently recommend a breast MRI before and after NAC. Clinical breast MRI detects breast cancer by contrast enhancement related to tumor angiogenesis; quantitative guidelines to determine complete pCR are lacking. Better methods are needed to detect complete pCR. The definition of a pCR varies with some groups defining it as the absence of residual invasive breast cancer (ductal carcinoma can be present) while others define it as the absence of residual invasive and in situ breast cancer [38].
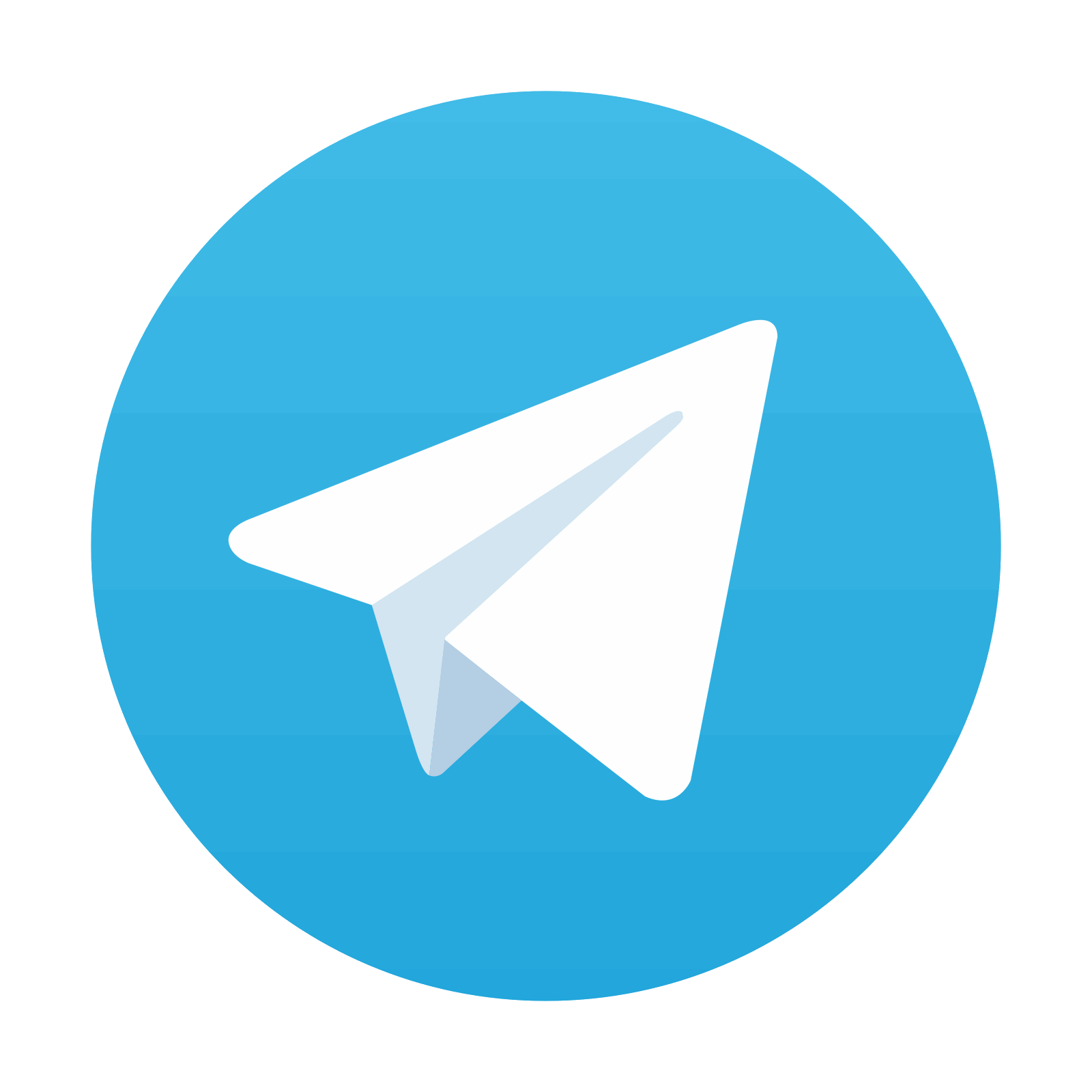
Stay updated, free articles. Join our Telegram channel

Full access? Get Clinical Tree
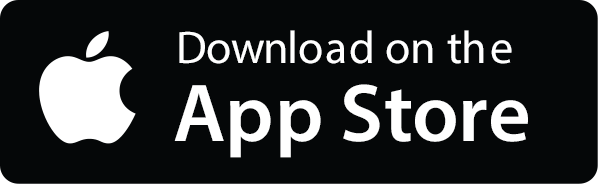
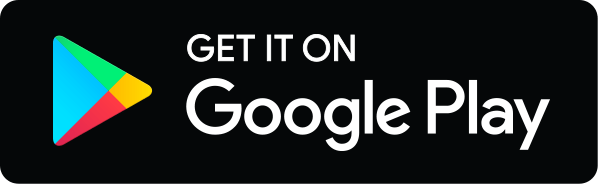