Phase
Regimen
Patient population
Results
I
Pembrolizumab (anti-PD-1 antibody)
Advanced solid tumours, including oesophageal cancer
ORR: 30.4 % (29.4 % in squamous cell carcinoma vs. 40 % in adenocarcinoma); 12-month PFS: 21.7 %
II
Nivolumab (anti-PD-1 antibody)
Advanced oesophageal squamous cell carcinoma
ORR: 17.2 %; median PFS: 1.5 months; median OS:10.8 months
Nivolumab, a fully human monoclonal IgG4 antibody specific for PD-1, was tested in a phase II trial for patients with advanced oesophageal cancer [93]. In this study, patients with advanced oesophageal squamous cell carcinoma who had failed standard therapies were treated with nivolumab 3 mg/kg every 2 weeks. Of 65 patients, 44 (66.7 %) had at least 3 prior lines of therapy. In this heavily pretreated cohort, 11 (17.2 %) of 64 evaluable patients experienced an objective response [1 complete response (CR) and 10 partial responses (PR)], while 25.0 % of patients had stable disease. The median PFS was 1.5 months and the median OS was 10.8 months. As has been observed with PD-1 inhibition in other solid tumours, patients who had CR or PR had durable responses (Table 6.1).
Based on these promising results, two large, randomised phase III trials of PD-1 blockade in oesophageal cancer have been initiated. The first study is evaluating the efficacy of pembrolizumab versus standard chemotherapy in patients with advanced oesophageal cancer who have progressed after first-line therapy (NCT02564263). The second study is designed to compare nivolumab with taxane chemotherapy in patients with unresectable advanced or recurrent oesophageal cancer in the second-line setting (NCT02569242). Several studies are also investigating the efficacy of PD-L1 inhibition in this patient population. In addition to PD-1 monotherapy, combinations of PD-1 and PD-L1 inhibitors with chemotherapy, radiation therapy or targeted therapy in the metastatic setting are being explored. Furthermore, PD-1 monoclonal antibodies are being tested in the adjuvant and neoadjuvant setting.
Gastric Cancer and Gastroesophageal Junction Cancer
Initial results of PD-1 pathway blockade in patients with gastric cancer were disappointing. Of seven patients with metastatic gastric cancer enrolled in the multi-cohort phase I trial of the anti-PD-L1 antibody BMS-936559, no objective responses were observed [12]. However, other PD-L1 inhibitors have shown promising activity in early phase trials, despite the small number of patients enrolled in those trials. In a multi-arm phase I trial of durvalumab or MEDI4736, 1 of 16 patients with advanced gastroesophageal cancer achieved a complete response, while 3 patients had partial responses (ORR 25 %). Eight additional patients had stable disease, resulting in a disease-control rate (DCR) of 75 % [94]. In addition, a phase I trial of atezolizumab (MPDL3280A) included one patient with metastatic gastric adenocarcinoma, who had a durable partial response lasting more than 48 weeks [95]. Based on these encouraging results, larger trials evaluating the efficacy of these inhibitors in advanced gastric and GEJ cancer are currently underway.
Monoclonal antibodies against PD-1 have been more rigorously evaluated in metastatic gastric adenocarcinoma. In KEYNOTE-012, a large multi-arm phase Ib trial investigating the activity of pembrolizumab in advanced solid tumours, 162 patients with previously treated metastatic gastric cancer were screened for PD-L1 positivity (defined as PD-L1 immunohistochemical staining in the stroma or in ≥1 % of tumour cells). Of these patients, 40 % (65) were found to be PD-L1-positive and 39 were enrolled in the trial. At a median follow up of 8.8 months, 13 (33 %) patients remained on therapy and the ORR was 22 % by central review. The median time to response was 8 weeks with a median duration of response of 40 weeks. Median PFS was 1.9 months; however, 26 % of patients remained progression-free at 6 months. Median OS was 11.4 months, with a 6-month OS rate of 66 %. In this preselected PD-L1-positive patient population, there was preliminary evidence that high PD-L1 expression was associated with ORR (1-sided P = 0.1) [96]. Pembrolizumab is currently being evaluated in large phase III trials either in combination with cytotoxic chemotherapy in the first-line setting or as monotherapy in the second-line setting in patients with advanced or metastatic gastric or GEJ adenocarcinoma (NCT02494583, NCT02370498).
The efficacy of nivolumab has also been investigated in metastatic gastric and GEJ cancer. The phase I/II CheckMate-032 study included 59 patients with advanced gastric or GEJ cancer, regardless of PD-L1 status. In this heavily pretreated patient population, the confirmed ORR was 14 % and DCR was 32 %. Patients with PD-L1-positive tumours had an ORR of 27 %, while only 12 % of patients with PD-L1-negative tumours had objective responses. The median duration of response was 7.1 months and the 12-month OS rate for the entire cohort was 36 % [97]. A phase III trial of nivolumab monotherapy after failure of standard therapies is currently ongoing (NCT02267343).
The results of completed trials of PD-1 and PD-L1 inhibitors in gastric and GEJ cancer are summarised in Table 6.2.
Table 6.2
Completed trials of PD-1/PD-L1 inhibitors in gastric and GEJ cancer
Phase | Regimen | Patient population | Results |
---|---|---|---|
I | Pembrolizumab (anti-PD-1 antibody) | Advanced solid tumours, including gastric or GEJ adenocarcinoma | ORR 22 % |
I | Durvalumab (MEDI4736, anti-PD-L1 antibody) | Advanced solid tumours, including gastroesophageal adenocarcinoma | Of 16 patients, 4 had objective responses, including one complete response |
I/II | Nivolumab (anti-PD-1 antibody) | Advanced solid tumours, including gastric or GEJ adenocarcinoma | ORR 14 % (ORR 27 % in PD-L1 positive patients vs. 12 % in PD-L1 negative patients) |
I/II | Nivolumab 1 mg/kg + Ipilimumab 3 mg/kg (N1 + I3) vs. Nivolumab 3 mg/kg + Ipilimumab 1 mg/kg (N3 + I1) vs. Nivolumab monotherapy (N3) | Advanced solid tumours, including gastric or GEJ adenocarcinoma | ORR: N1 + I3 26 % vs. N3 + I1 10 % vs. N3 14 %; OS: N1 + I3 6.9 months vs. N3 + I1 4.8 months vs. N3 5.0 months |
Colorectal Cancer
Preclinical studies of PD-1 inhibition in colorectal cancer generated great enthusiasm for the use of these novel therapeutic approaches in this disease. Iwai and colleagues intravenously injected wildtype and PD-1 knockout mice (PD-1−/−) with murine colon cancer cells, establishing a syngeneic mouse model of colon cancer. Dissemination of tumour cells to the lung was significantly decreased in the PD-1−/− mice. Similar results were observed when mice were treated with PD-1 monoclonal antibodies [98]. In a more recent study utilising the same syngeneic mouse models, response rates to PD-1, PD-L1 and CTLA-4 monotherapy were 25 %, 33 % and 50 % respectively. Combinations of PD-1 or PD-L1 inhibitors with CTLA-4 blockade had an additive effect with a response rate of 75 %. Further addition of the GVAX cancer vaccine to dual checkpoint inhibition led to complete eradication of the murine tumours [99].
Despite these promising preclinical data, the initial results of early phase trials of PD-1 and PD-L1 inhibition in colorectal cancer were disappointing. In the phase I trial of nivolumab in solid tumours, 19 patients with heavily pretreated metastatic colorectal cancer were included. However, no objective responses were observed in this cohort [11]. Additionally, no clinical activity was observed with the anti-PD-L1 monoclonal antibody BMS-936559 in a phase I trial, which included 18 patients with metastatic colorectal cancer [12]. Interestingly, in another phase I study of the PD-L1 antibody atezolizumab, one of four patients with metastatic colorectal cancer achieved a partial response [95]. Furthermore, the combination of atezolizumab with the anti-VEGF-A monoclonal antibody bevacizumab with or without cytotoxic chemotherapy was explored in a phase Ib study. Patients with refractory metastatic colorectal cancer were treated with atezolizumab and bevacizumab, while chemotherapy naïve patients were treated with atezolizumab, bevacizumab and cytotoxic chemotherapy (5-fluorouracil, leucovorin, oxaliplatin [FOLFOX]). Preliminary results from this trial showed an unconfirmed ORR of 7 % (1 of 14 patients) in the refractory cohort, and 48 % (11 of 23 patients) in the chemotherapy naïve cohort [100], which is similar to previously reported response rates with the combination of FOLFOX and bevacizumab in the first-line setting (ORR 45 %) [101].
Despite the disappointing results of PD-1 and PD-L1 blockade in unselected patients with colorectal cancer, a small subset seem to have better responses. Interestingly, among 33 patients with colorectal cancer that were enrolled in the early phase trials of PD-1 inhibitors, only one patient had an objective response. This patient, whose tumour was later found to be mismatch-repair deficient, achieved a complete response and remained disease-free for 3 years [102]. Based on this anecdotal case, a phase II clinical trial evaluating the effect of pembrolizumab in patients whose tumours had or did not have MMR-deficiency was initiated [103]. This study included 41 patients; 20 patients with dMMR colorectal and noncolorectal cancers and 21 patients with pMMR colorectal cancers. Among 10 patients with dMMR colorectal cancer, 4 (40 %) patients achieved objective responses and 5 (50 %) patients had stable disease. In contrast, no patient with pMMR colorectal cancer had an objective response and only 2 patients (11 %) experienced disease stabilisation. PFS at 20 weeks was 78 % in the dMMR colorectal cancer cohort and 11 % in the pMMR colorectal cancer group. Notably, patients with dMMR non-colorectal cancer had responses similar to those of patients with dMMR colorectal cancer (71 % [5 of 7 patients]). Based on these results, the clinical activity of pembrolizumab is currently being evaluated in a larger, single-arm phase II (NCT02460198) and a randomised phase III trial (NCT02563002) for patients with metastatic dMMR colorectal cancer. In addition, a phase II trial of durvalumab in dMMR colorectal cancer is currently enrolling patients (NCT02227667). Further research is needed to understand why immune checkpoint inhibition has largely failed in pMMR colorectal cancer and to develop strategies that will enhance the anti-tumour effect of immunotherapeutic approaches in this disease.
A summary of the completed trials of PD-1 and PD-L1 inhibitors is provided in Table 6.3.
Table 6.3
Completed trials of PD-1/PD-L1 inhibitors in colorectal cancer
Phase | Regimen | Patient population | Results |
---|---|---|---|
I | Nivolumab (anti-PD-1 antibody) | Advanced solid tumours, including CRC | Of 19 patients, no objective responses were observed |
I | BMS-936559 (anti-PD-L1 antibody) | Advanced solid tumours, including CRC | Of 18 patients, no objective responses were observed |
I | Atezolizumab (anti-PD-L1 antibody) | Advanced solid tumours, including CRC | Of 4 patients, one achieved a partial response |
Ib | Atezolizumab (anti-PD-L1 antibody) + Bevacizumab +/− FOLFOX | Metastatic CRC (chemotherapy naïve and refractory) | ORR: 7 % in the refractory cohort (atezolizumab + bevacizumab), 48 % in the chemotherapy naïve cohort (atezolizumab + bevacizumab + FOLFOX) |
II | Pembrolizumab (anti-PD-1 antibody) | Metastatic CRC, dMMR vs. pMMR | ORR: 40 % in the dMMR group vs. 0 in the pMMR group; PFS at 20 weeks: 78 % in the dMMR group vs. 11 % in the pMMR group |
Pancreatic Cancer
Pancreatic cancer is considered a non-immunogenic tumour due to the paucity of T cell infiltrates in the tumour microenvironment. Therefore, immune checkpoint inhibitors that restore T cell function are unlikely to be effective in such an environment. The first trials of PD-1 and PD-L1 blockade were disappointing. In the multi-cohort phase I trial of the PD-L1 antibody BMS-936559, no objective responses were seen in patients with pancreatic cancer [12]. The only immune checkpoint inhibitor that has shown some activity in pancreatic cancer is the anti-PD-L1 monoclonal antibody durvalumab. Preliminary results of the multi-cohort phase Ib trial of durvalumab demonstrated an ORR of 7 % (2 of 29 patients) and a DCR of 21 % (6 of 29 patients) [104] (Table 6.4).
Table 6.4
Completed trials of PD-1/PD-L1 inhibitors in pancreatic ductal adenocarcinoma and hepatocellular carcinoma
Phase | Regimen | Patient population | Results |
---|---|---|---|
Ib | Durvalumab (anti-PD-L1 antibody) | Metastatic pancreatic cancer | ORR: 7 %; DCR: 21 % |
I/II | Nivolumab (anti-PD-1 antibody) | Advanced HCC | ORR: 19 %; ORR: 10 % in HBV group vs. 36 % in HCV group vs. 14 % in the uninfected group; OS at 12 months: 62 % |
Hepatocellular Carcinoma
Following the encouraging results of CTLA-4 blockade in HCC [105], PD-1 pathway inhibition was tested in this disease. Clinical activity of PD-L1 blockade was initially observed in the multi-cohort phase I dose-expansion study of durvalumab. Of 21 HCC patients enrolled in the trial, 5 had HBV-associated disease and 4 had HCV-associated HCC. Of 20 evaluable patients, one patient achieved an objective response, while the DCR at 12 weeks was 25 % (5 of 20 patients) [104].
Further evidence of the efficacy of PD-1 blockade was seen in the phase I/II trial of nivolumab in patients with advanced HCC [106]. The study included 47 patients with advanced HCC with Child-Pugh score ≤B7 and progressive disease on, or intolerant of sorafenib. Patients were divided into three cohorts according to their hepatitis viral status. Of all patients, 11 (23 %) were HBV-positive, 12 (26 %) were HCV-positive and 24 (51 %) were not infected by either virus. The majority of patients had extrahepatic metastases (33 of 47, 70 %) and had received prior sorafenib (32 of 47, 68 %). Of 42 evaluable patients, 8 achieved an objective response (2 CR and 6 PR), with responses extending beyond 12 months in 4 out of 8 responders. In addition, 20 patients (48 %) had disease stabilisation. Notably, the OS rate at 9 and 12 months were 70 % and 62 %, respectively. Objective responses were seen in all three cohorts with 1 responder (10 %) in the HBV group, 4 responders (36 %) in the HCV group and 3 responders (14 %) in the uninfected group (Table 6.4). The study has been expanded to include two additional cohorts, one comparing the efficacy of nivolumab with sorafenib, and the other one exploring the combination of nivolumab and ipilimumab for the treatment of patients with advanced HCC (NCT01658878). An additional phase III trial comparing the efficacy of nivolumab with sorafenib as first-line therapy for advanced HCC is currently recruiting patients (NCT02576509).
Biomarkers of Response to PD-1 Pathway Inhibition
It has become clear that only a subset of patients with gastrointestinal malignancies respond to PD-1/PD-L1 inhibitors. Therefore, to improve patient outcomes we need to optimise patient selection. Development of biomarkers that can predict which patients will derive benefit from these agents is an area of active investigation.
PD-L1 expression as a potential biomarker has been studied extensively in several malignancies. In the phase I study of nivolumab, PD-L1 expression on tumour cells was assessed by immunohistochemistry in 42 tumours. There was a significant correlation between PD-L1 expression and response to PD-1 therapy across multiple tumour types, including colorectal cancer. Of 25 patients with PD-L1–positive tumours (defined as ≥5 % of tumour cells expressing PD-L1), 9 (36 %) responded to nivolumab, whereas no patients with PD-L1–negative tumours responded to therapy [11]. Herbst and colleagues reported a significant correlation between PD-L1 expression in the tumour-infiltrating immune cells and response to anti-PD-L1 therapy in several tumour types, including gastric cancer [95]. In this study, patients whose tumours expressed PD-L1 in ≥5 % of infiltrating immune cells had an ORR of 34 %, whereas 16 % of patients whose tumours expressed PD-L1 in ≤5 % of immune cells responded to anti-PD-L1 therapy. Similar findings were reported in melanoma with PD-L1 expression in the tumour cells being predictive of response to pembrolizumab, as well as prolonged PFS. However, 9–20 % of patients with PD-L1–negative tumours had a durable response to pembrolizumab, and median OS did not differ significantly between patients with PD-L1-positive and negative tumours [107]. These results demonstrate that, although PD-L1 expression correlates with higher response rates to PD-1 and PD-L1 inhibitors, patients without PD-L1 expression may also benefit from these therapies.
Few biomarkers studies in gastrointestinal malignancies have been reported. In the KEYNOTE-012 trial of pembrolizumab in metastatic gastric cancer, there was a trend towards an association between high PD-L1 expression and ORR (1-sided P = 0.10) [96]. However, all patients in this study had PD-L1-positive tumours (immunohistochemical staining in the stroma or in ≥1 % of tumour cells). In contrast, in the CheckMate-032 study, which included patients with gastric tumours regardless of PD-L1 status, PD-L1 expression was associated with higher ORR to nivolumab (27 % vs. 12 %) [97].
Several technical and biological issues currently preclude the use of PD-L1 expression as a robust predictive biomarker. These include: (1) differing definitions of PD-L1 positivity threshold by immunohistochemistry, (2) variable detection antibodies, (3) use of archival versus fresh biopsies, (4) heterogeneous expression of PD-L1 in the primary tumour and metastatic sites, (5) evaluation of staining in the tumour cells or the infiltrating immune cells [108]. Further standardisation of PD-L1 immunohistochemical assays will be essential for the development of useful predictive models.
In addition to PD-L1, efforts to identify other biomarkers are currently ongoing. Herbst and colleagues utilised gene expression profiling to predict responses to anti-PD-L1 therapy. Tumours in responders to PD-L1 blockade demonstrated elevated expression of IFN-γ and IFN-γ-inducible genes (e.g. IDO1 and CXCL9) [95]. Similar analysis was performed in gastric tumours from patients enrolled in KEYNOTE-012. Patients with low expression of IFN-γ and TCR-signalling signatures did not respond to pembrolizumab [109]. Furthermore, as noted above, high mutational burden is associated with better responses to immune checkpoint blockade [32, 33, 103]. However, further work is needed to determine which mutations are more likely to generate neoantigens that can induce a robust anti-tumour response. Lastly, establishing predictive biomarkers of resistance to PD-1 pathway blockade is also essential. Recent studies have explored the role of the WNT/β-catenin pathway in resistance to immune checkpoint inhibitors. Gene expression profiling across tumour types included in TCGA was used to classify tumours as T-cell inflamed or non-inflamed. Genomic sequencing revealed that tumours lacking a T cell infiltrate had activation of the WNT/β-catenin pathway, by activating mutations in CTNNB1, inactivating mutations in APC or overexpression of other components of the pathway [110]. As the WNT/β-catenin pathway is commonly activated in colon cancer, these data may explain the lack of response of these tumours to immune checkpoint inhibition. These and other assays will be critical in our efforts to identify patients who will benefit most from immune checkpoint blockade.
Combination Approaches Involving PD-1/PD-L1 Blockade
Inhibitors of PD-1 or PD-L1 have achieved durable responses in some patients with gastrointestinal cancers. In order to enhance the anti-tumour benefit of these immune checkpoint inhibitors, we need to understand the mechanisms of immune escape and identify other therapies that may synergise with these agents. Rational combinations of PD-1 and PD-L1 inhibitors with other immunotherapies or conventional therapies are currently being explored in clinical trials (Fig. 6.1).
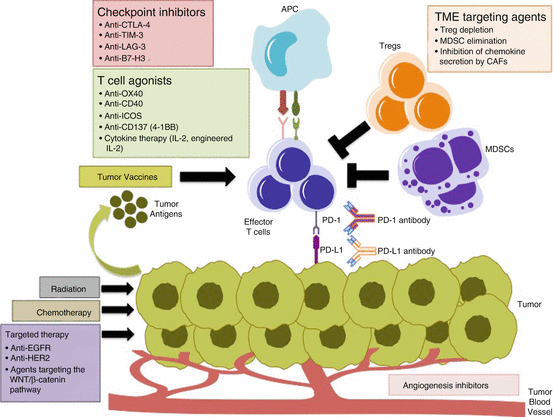
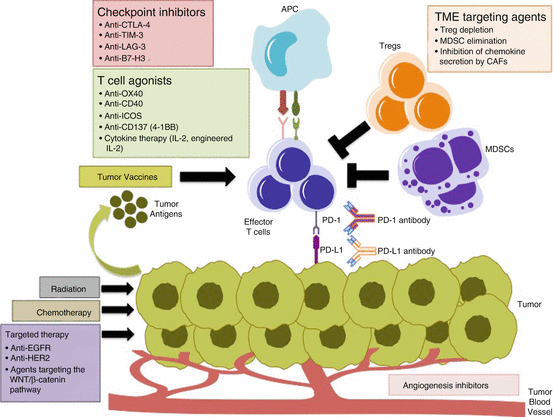
Fig. 6.1
Combination strategies with PD-1/PD-L1 inhibitors. Inhibitors of other immune checkpoints and T cell agonists can further increase T cell activity when combined with PD-1/PD-L1 inhibitors. Elimination of the immunosuppressive components of the tumour microenvironment (Tregs, MDSCs, VEGF) can enhance the anti-tumour effects of PD-1 blockade. Direct targeting of the tumour with radiation, cytotoxic chemotherapy or targeted therapy induces cancer cell death, increases tumour antigen presentation and promotes activation of effector T cells. Similarly, vaccines against tumour antigens can initiate an anti-tumour immune response by enhancing the influx of T cells into the tumour, and synergise with PD-1 pathway blockade. APC antigen-presenting cell, Tregs regulatory T cells, MDSCs myeloid derived suppressor cells, TME tumour microenvironment
Combinations of PD-1/PD-L1 Blockade and Other Immune Checkpoint Inhibitors
Combinations of anti-PD-1/PD-L1 monoclonal antibodies and other immune checkpoint inhibitors may lead to more robust antitumour effects in gastrointestinal cancers. Indeed, in advanced melanoma, the combination of PD-1 and CTLA-4 blockade demonstrated a synergistic effect compared to either inhibitor alone [111]. The combination of PD-1 and CTLA-4 inhibition is currently being explored in gastric, colorectal, hepatocellular and pancreatic cancer. Preliminary analyses of the combination of nivolumab and ipilimumab in gastric, GEJ and dMMR colorectal cancer have been reported. In CheckMate-032, 160 patients with metastatic gastric or GEJ cancer were treated with nivolumab alone (3 mg/kg) or in combination with ipilimumab (two cohorts; nivolumab 1 mg/kg + ipilimumab 3 mg/kg or nivolumab 3 mg/kg + ipilimumab 1 mg/kg). The ORR was 14 % for the nivolumab alone group (N3), 26 % for the nivolumab 1 mg/kg + ipilimumab 3 mg/kg group (N1 + I3) and 10 % for the nivolumab 3 mg/kg + ipilimumab 1 mg/kg group (N3 + I1). Median OS was 5.0 months (95 % CI, 3.4–12.4 months) for the N3 group, 6.9 months (95 % CI, 3.6 months to NR) for the N1 + I3 group and 4.8 months (95 % CI, 3.0–9.1 months) for the N3 + I1 group [112]. The interim analysis of CheckMate-132 included 56 patients with dMMR metastatic colorectal cancer, treated with nivolumab 3 mg/kg (N3) or nivolumab 3 mg/kg and ipilimumab 1 mg/kg (N3 + I1). The ORR was 27 % for the N3 group and 15 % for the N3 + I1. Despite the higher response rate in the monotherapy group, the combination was superior with regard to PFS and OS. The 4-month PFS was 55 % for the N3 group and 80 % for the N3 + I1 group, while the 5-month OS was 75 % for the N3 group and 100 % for the N3 + I1 group [113]. These preliminary results demonstrate a promising clinical activity of immune checkpoint inhibitor combinations in gastrointestinal malignancies. Further research is needed to identify patients who are more likely to respond to these combinations compared to PD-1 monotherapy.
As noted above, several other co-inhibitory receptors, such as TIM-3, LAG-3, B7-H3, are expressed in gastrointestinal tumours and are associated with poor prognosis [54, 66, 68, 89, 90]. Preclinical studies in murine models of colon cancer have demonstrated synergy between PD-1 and LAG-3 blockade, leading to complete regression of the tumours in the majority of mice treated with this combination [114]. A large number of early phase trials are currently investigating the safety and efficacy of inhibitors targeting LAG-3 and other immune checkpoints in combination with PD-1 blockade in advanced solid tumours, including gastrointestinal malignancies (Table 6.5).
Table 6.5
Selected ongoing trials of therapeutic combinations with PD-1/PD-L1 blockade
Phase | Agents | Patient population | NCT identifier |
A. Other immune check point inhibitors | |||
Ib/II | Durvalumab + Tremelimumab (anti-CTLA-4) | Recurrent or metastatic gastric and GEJ cancer | NCT02340975 |
II | Durvalumab + Tremelimumab (anti-CTLA-4) | Unresectable HCC | NCT02519348 |
I-Ib/II | PDR001 (PD-1 antibody) + MBG453 (anti-TIM-3 antibody) | Advanced or metastatic solid tumours | NCT02608268 |
I/IIa | Nivolumab + BMS-986016 (anti-LAG-3 antibody) | Advanced or metastatic gastric cancer, HCC, CRC | NCT01968109 |
I | Pembrolizumab + MGA271 (anti-B7-H3 antibody) | Advanced pancreatic cancer and B7-H3 expressing CRC | NCT02475213 |
B. T cell agonists | |||
I | Durvalumab + MEDI6383 (OX40 agonist) | Advanced solid tumours | NCT02221960 |
I | Durvalumab + Tremelimumab + MEDI0562 (OX40 agonist) | Advanced solid tumours | NCT02705482 |
I | Pembrolizumab + GSK3359609 (ICOS agonist) | Advanced solid tumours, including oesophageal cancer and CRC | NCT02723955 |
I | Nivolumab + Urelumab (CD137 agonist) | Advanced solid tumours | NCT02534506 |
I | Nivolumab + Varilumab (CD27 agonist) | Advanced solid tumours, including CRC | NCT02335918 |
I | Atezolizumab + RO7009789 (CD40 agonist) | Advanced solid tumours | NCT02304393 |
C. Targeted therapy | |||
Ib/II | Pembrolizumab + Trastuzumab (anti-HER2 antibody) or Cetuximab (anti-EGFR antibody) | HER2 overexpressing unresectable gastric and GEJ cancer, unresectable KRAS, NRAS and BRAF wildtype CRC | NCT02318901 |
Ib/II | Pembrolizumab + Margetuximab (anti-HER2 antibody) | Advanced or metastatic HER2+ gastric and GEJ cancer | NCT02689284 |
Ib/II | Pembrolizumab + Cetuximab (anti-EGFR antibody) | Metastatic KRAS and NRAS wildtype CRC | NCT02713373 |
D. Agents targeting the tumour microenvironment | |||
I | Pembrolizumab + Ramucirumab (anti-VEGFR2 antibody) | Advanced or metastatic gastric and GEJ cancer | NCT02443324 |
I | Durvalumab + Ramucirumab (anti-VEGFR2 antibody) | Advanced gastric and GEJ cancer, HCC | NCT02572687 |
I | Atezolizumab + Bevacizumab (anti-VEGF antibody) + chemotherapy | Advanced or metastatic gastric and GEJ cancer, metastatic pancreatic cancer, HCC | NCT02715531 |
I | Durvalumab + Mogamulizumab (anti-CCR4 antibody) | Advanced solid tumours, including pancreatic cancer | NCT02301130 |
I/IIa | Pembrolizumab + PLX3397 (CSF-1R inhibitor) | Advanced solid tumours, including pancreatic cancer | NCT02452424 |
I/II | Durvalumab + Epacadostat (IDO inhibitor) | Advanced solid tumours, including gastric and GEJ cancer | NCT02318277 |
I/II | Nivolumab + Epacadostat (IDO inhibitor) | Advanced solid tumours, including CRC | NCT02327078 |
I/II | Nivolumab + Ulocuplumab (anti-CXCR4 antibody) | Advanced pancreatic cancer | NCT02472977 |
E. Cytotoxic chemotherapy | |||
II | Pembrolizumab + cisplatin/5-FU | Recurrent or metastatic gastric and GEJ cancer | NCT02335411 |
I/IIA | Pembrolizumab + mFOLFOX6 | Advanced or metastatic gastric and GEJ, CRC, biliary tract and pancreatic cancer | NCT02268825 |
Ib/II | Nivolumab + irinotecan/capecitabine | Advanced solid tumours, including CRC and pancreatic cancer | NCT02423954 |
Ib/II | Pembrolizumab + gemcitabine/nab-paclitaxel | Metastatic pancreatic cancer | NCT02331251 |
F. Radiation therapy | |||
Ib/II | Neoadjuvant Pembrolizumab + chemoradiotherapy | Locally advanced GEJ or gastric cardia cancer that can be surgically removed | NCT02730546 |
Ib/II | Neoadjuvant Pembrolizumab + chemoradiotherapy | Resectable or borderline resectable pancreatic cancer | NCT02305186 |
II | Pembrolizumab + radiotherapy or ablation | Metastatic CRC | NCT02437071 |
II | Neoadjuvant Pembrolizumab + chemoradiotherapy | Stage II-III rectal cancer | NCT02586610 |
G. Vaccines | |||
II | Nivolumab + GVAX + CRS-207 | Metastatic pancreatic cancer | NCT02243371 |
II | Adjuvant or Neoadjuvant Nivolumab + GVAX | Resectable pancreatic cancer | NCT02451982 |
II | Pembrolizumab + GVAX + stereotactic radiotherapy | Locally advanced pancreatic cancer | NCT02648282 |
Combinations of PD-1/PD-L1 Blockade and T-Cell Agonists
An alternative strategy to augment the efficacy of PD-1 inhibition is to increase T cell activity. Several co-stimulatory molecules, including members of the TNF receptor family (OX40, CD137 [4-1BB] CD40 and CD27) and members of the B7-CD28 family members (ICOS and B7-H3), lead to enhanced memory and effector T cell responses, increased T cell survival and regression of tumours in murine models of solid tumours [115–119].
Specifically, in preclinical models of colorectal cancer, activation of CD137 with an agonistic monoclonal antibody enhanced NK cell degranulation and cytotoxicity. Combined anti-EGFR and anti-CD137 therapy was synergistic and resulted in complete tumour resolution and prolonged survival [120]. In addition, the clinical impact of a CD40 agonist monoclonal antibody in combination with gemcitabine chemotherapy was evaluated in a phase I clinical trial for patients with chemotherapy-naïve advanced or metastatic pancreatic cancer. Of 21 patients enrolled in the trial, 4 patients (19 %) achieved PR and 11 patients had stable disease. Median PFS was 5.6 months and median OS was 7.4 months. Gemcitabine monotherapy historically achieves an ORR of 5.4 % with median PFS of 2.3 months and median OS of 5.7 months. Therefore, the combination of anti-CD40 and gemcitabine showed promising efficacy in patients with metastatic pancreatic cancer [121].
Based on these encouraging results, combinations of PD-1/PD-L1 inhibitors and T-cell agonists are currently being explored in early phase trials (Table 6.5).
Combinations of PD-1/PD-L1 Blockade and Targeted Therapies
Monoclonal antibodies targeting the human epidermal growth factor (HER) family receptors have been in clinical use in gastrointestinal malignancies for many years. Specifically, the anti-EGFR monoclonal antibodies, cetuximab and panitumumab, are approved for the treatment of KRAS wild-type advanced or metastatic colorectal cancer, while the anti-HER2 monoclonal antibody, trastuzumab, is approved as first-line therapy of metastatic, HER2-positive gastroesophageal cancer. In addition to blocking the EGFR/HER2 signalling pathway, these therapies have a significant impact on the tumour immune microenvironment by promoting antibody-dependent cellular cytotoxicity. Preclinical studies have demonstrated that the therapeutic effect of anti-EGFR and anti-HER2 monoclonal antibodies is dependent on CD8+ and NK cells [122, 123]. In addition, studies in immunocompetent mouse models of HER2-positive breast cancer suggest that PD-1 blockade can significantly improve the therapeutic efficacy of anti-HER2 monoclonal antibody therapy [123]. This potential synergistic effect of PD-1 inhibition and anti-EGFR or anti-HER2 therapy is currently being investigated in KRAS wild-type colorectal cancer and HER2-positive gastric and oesophageal cancer, respectively (Table 6.5).
Combinations of PD-1/PD-L1 Blockade and Agents Targeting the Tumour Microenvironment
Another approach to enhance the efficacy of PD-1/PD-L1 inhibitors is to combine them with agents targeting other immunosuppressive components of the tumour microenvironment.
Emerging data show that VEGF plays an important role in tumour immune evasion. In addition to promoting tumour angiogenesis, VEGF blocks the maturation of dendritic cells and therefore impairs antigen presentation, inhibits lymphocyte trafficking through the vasculature into tumours and enhances T cell exhaustion by upregulating immune checkpoint expression [124–128]. Angiogenensis inhibitors, particularly anti-VEGFA monoclonal antibodies, have been shown to augment the anti-tumour immune response and synergise with PD-1 blockade in both preclinical and early clinical studies [128, 129]. In a mouse model of colorectal cancer expressing high levels of VEGF-A, combined therapy with anti-PD-1 and anti-VEGF-A monoclonal antibodies led to a significant decrease in tumour volume, compared to either agent alone. Of note, anti-VEGF-A therapy decreased the proportion of tumour-infiltrating CD8+ T cells expressing immune checkpoints [128]. In patients with metastatic melanoma, combination therapy with bevacizumab and ipilimumab enhanced lymphocyte and macrophage trafficking leading to a more robust immune cell infiltration into the tumour, compared to ipilimumab monotherapy [129]. These studies provide the mechanistic foundation for combining antiangiogenic therapy with immune checkpoint blockade. Clinical trials evaluating the efficacy of combinatorial treatment with PD-1 blockade and monoclonal antibodies targeting VEGF-A or its receptor are currently ongoing in a variety of gastrointestinal malignancies (Table 6.5).
An additional approach to augment anti-PD-1 therapy is the depletion of Tregs. Chemokine receptor 4 (CCR4) is expressed selectively in effector Tregs, which promote immunosuppression. Preclinical in vitro studies demonstrated that CCR4 blockade led to the elimination of Tregs and the induction of T-cell responses specific to human tumour antigens. In addition, administration of the anti-CCR4 monoclonal antibody mogamulizumab to patients with adult T-cell leukaemia-lymphoma selectively depleted effector Tregs, enhanced immune responses and significantly reduced tumour cells [130]. Combination of mogamulizumab with either PD-L1 or CTLA-4 inhibitors is currently being tested in patients with metastatic pancreatic cancer (Table 6.5).
Critical drivers of the immune escape in gastrointestinal malignancies include tumour-associated macrophages (TAMs) and myeloid derived suppressor cells (MDSCs). These cells not only contribute to immune suppression, but also promote cancer cell proliferation, mediate resistance to cytotoxic chemotherapy and augment the metastatic potential of solid tumours [131, 132]. Colony stimulating factor 1 receptor (CSF1R) is expressed on TAMs and MDSCs and can be targeted with monoclonal antibodies. In preclinical models of pancreatic cancer, CSF1/CSF1R blockade was shown to decrease the number of TAMs and reprogram remaining TAMs to support antigen presentation and bolster T cell activation within the tumour microenvironment. This in-turn led to reduced immune suppression and elevated interferon responses, which restrained tumour progression. However, inhibition of CSF1R also increased the levels of PD-L1 and CTLA-4 in these tumours, potentially limiting its long-term efficacy [133]. One rational strategy to overcome immune evasion is to combine PD-1/PD-L1 or CTLA-4 inhibitors with CSF1R blockade. This approach is under investigation in several solid tumours, including pancreatic and gastric cancer (Table 6.5).
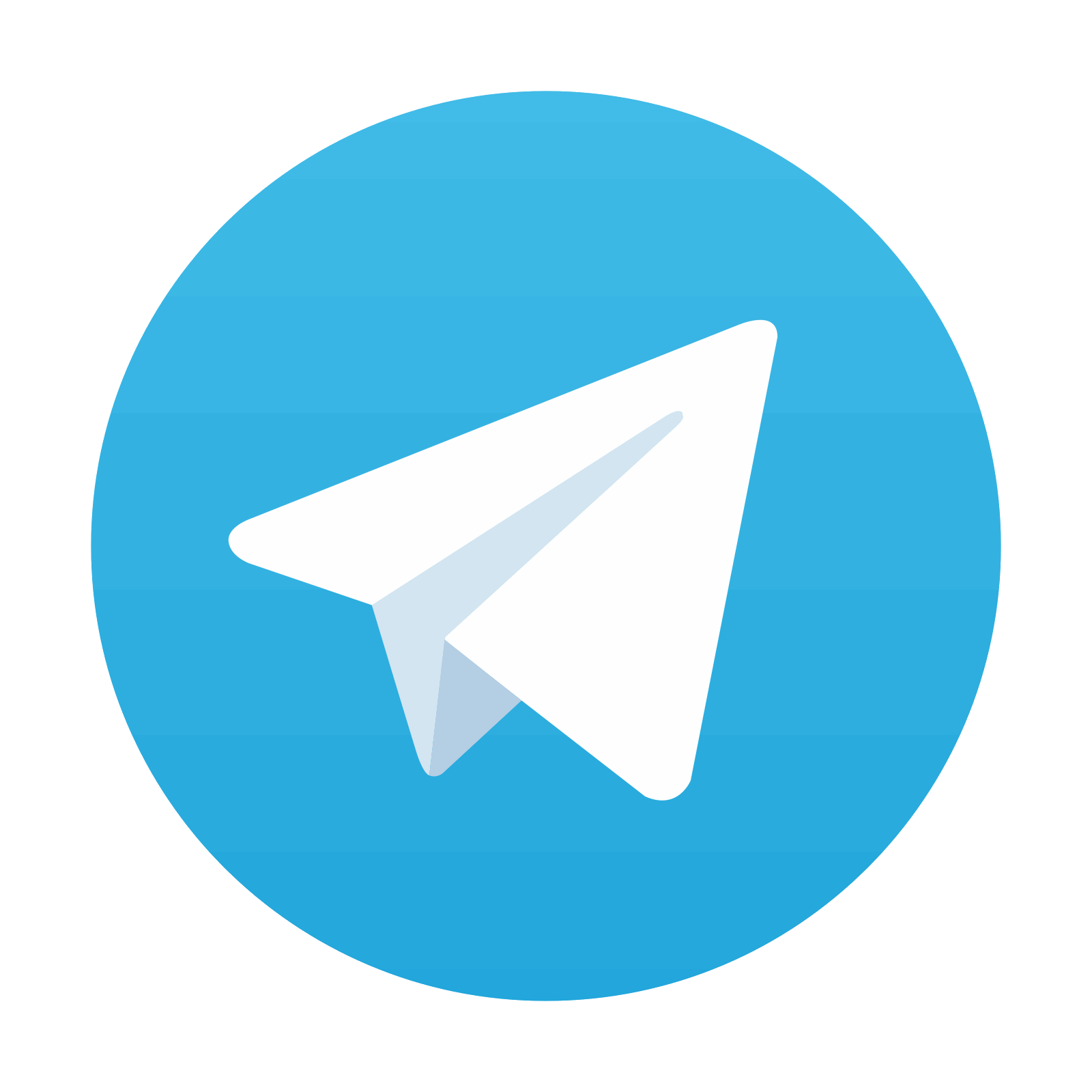
Stay updated, free articles. Join our Telegram channel

Full access? Get Clinical Tree
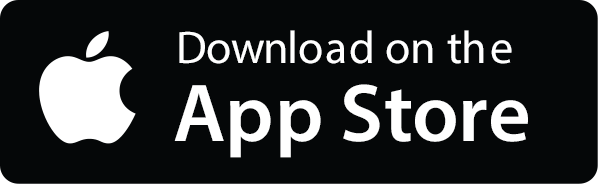
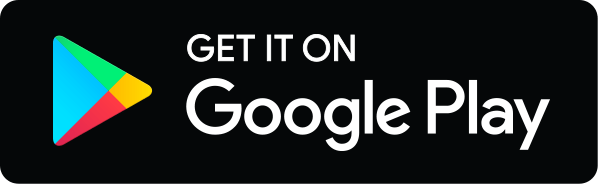