The significant expansion of the number of PDXs for prostate cancer by these groups was attributed especially to the significant investments and dedication transplanting large numbers of patient samples to compensate for the general low success rate. In order to understand the high success rates experienced in the NMRI nude mice by the Rotterdam Group, the putative role of (nonpathogenic) murine viruses in triggering PDX establishment has been an interesting suggestion. This hypothesis is supported by the later observation that all newly established PDXs were found to contain murine leukemia virus (MLV). Indeed, we reported on highly activated stroma in these PDXs with increased susceptibility to develop murine lymphomas [29]. Later studies indicated that prostate cancer appeared to have a propensity for infection with murine gamma retroviruses [30], although it remains unclear if infection with these viruses is necessary for the establishment of prostate cancer cell lines and PDXs [31, 32].
In line with the initial rather poor successes to establish PDXs of prostate cancer, a similar difficult development was seen when patient material was directly used for cell cultures. Historically, only a few cell lines were successfully derived from human tissue, namely PC3, DU145, and LNCaP [33–35]. Although only LNCaP shows the important feature of androgen responsiveness (driven by a mutated AR), these cell lines are still among the most frequently used in prostate cancer research. Despite major developments in culture techniques and protocols, only two additional prostate cancer cell lines, i.e., MDA PCa 1 and MDA PCa 2a/b, could be established directly from the patient [36]. More successfully, several cell lines, such as PC346C, VCaP, DUCaP, LAPC4, and CWR22Rv1, could be generated from established PDXs [25, 37–40]. As already indicated above, these PDX-derived cell lines are contaminated with MLV virus, as a result of the original passaging in mice [31, 32].
In an effort to improve growth efficiency, the traditional athymic nude mouse as host for PDX engraftment was replaced by mouse strains that were more immune deficient. Since most nude mice are “leaky” and do have a few T-cells, especially as they age, knockout mice with more complete defects in the immune system have been constructed. In 1983, the severe combined immunodeficient (SCID) mouse was reported, lacking both T-and B-cells [41]. Crossbreeding of SCID and the nonobese diabetic (NOD) mouse, which was characterized by an impaired innate immunity, resulted in NOD-SCID mice, with defects in both innate and adaptive immunity [42]. Later, other knockout mouse strains were developed using genetic engineering to induce specific mutations (Rag1 and Rag2) that prevent mature T- and B-cell development and mutations (IL-2rγ) preventing natural killer (NK)-cell development. The crossing of IL-2rγnull mice with Rag1null, Rag2null or NOD/SCID (NSG) mice provided novel mouse strains with even more profound immunological defects, contributing to an increase in the number of PDXs for prostate cancer [25, 43]. Besides the immune deficiency of the host animal, also engraftment site (subcutaneous, orthotopic, or subrenal capsule) may have added to the increased success rates, as was shown by the eminent development of the Vancouver PDX series, using subrenal capsule engraftment [44].
These technological advances are leading the expansion of current PDX collections. In the current PDX series, tumor samples from late-stage disease are overrepresented, showing higher take rates than early well-differentiated, androgen-dependent tumors (Fig. 8.2). Moreover, to cover today’s multitude of treatment options for late-stage disease, it is increasingly relevant to include PDX models representing resistance toward the various (targeted) therapies. Finally, there is a lack of prostate cancer PDXs that spontaneously metastasize to the bone, the preferential metastatic site in prostate cancer. Ongoing efforts are focused on expanding the PDX assortment to cover the phenotypic spectra of the different stages of prostate cancer disease. Clearly, in order to achieve such a well-balanced panel of prostate cancer PDXs that reflect the current patient population, major dedication and coordinated efforts from both the research center and their clinical partners are essential.
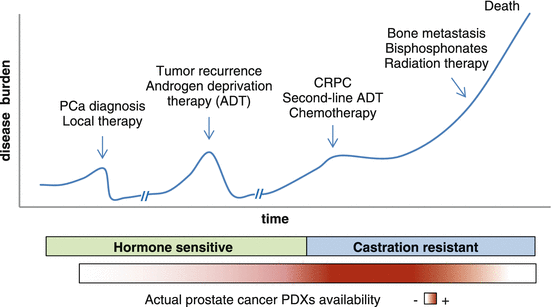
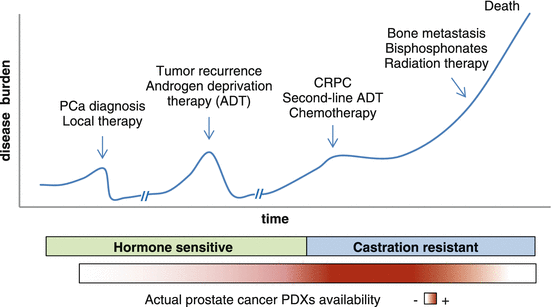
Fig. 8.2
Typical clinical history and standard treatment of progressive prostate cancer. Most prostate cancer PDX models available are representative of the more advanced castration-resistant stage. There is a lack of well-differentiated, hormone-sensitive models, as well as PDXs that spontaneously metastasize to the bone to represent the earlier and later stages of the disease, respectively. ADT androgen deprivation therapy; CRPC castration-resistant prostate cancer
Do PDX Models Recapitulate the Complexity of Human Prostate Cancer?
Prostate cancer is a heterogeneous and often multifocal disease [45]. This heterogeneity manifests itself not only in the variability between different patients but also within one patient’s tumor, where multiple cancer foci in the prostate may differ in histological grade and/or expression of molecular markers [46–49]. Recent next-generation sequencing studies revealed the presence of multiple clonal populations within a patient’s tumor, marked by spatial heterogeneity across different foci and a dynamic clonal composition, that evolves during disease progression and therapy [50–53]. In addition to clonal composition, tumor complexity is also defined by micro-environmental factors, such as extracellular matrix, stroma, and immune cells. Both clonal heterogeneity and complexity are not accurately represented in the conventional in vitro cell lines. PDX models are derived directly from patient tumors, without the selective pressure of prior in vitro expansion, thereby thought to be a better representation of the original tumor in terms of tissue complexity and clonal heterogeneity.
Overall, we and others have shown that prostate cancer PDXs largely recapitulate the original morphology, androgen sensitivity, and expression of the major biomarkers, such as AR and PSA [22, 23, 54, 55]. Genetic and genomic profiling studies have shown that these prostate cancer PDXs preserve major genetic alterations (e.g., AR, TMPRSS2-ERG, PTEN, and TP53) and global gene expression of the original tumor samples [54–57]. Furthermore, prostate cancer PDX models, depending on their disease stage, reflect the response to conventional systemic therapies, such as androgen deprivation therapy (ADT) and taxane chemotherapy [54, 58, 59]. Knowledge of these phenotypic and genomic characteristics of the xenografts is crucial when choosing the most appropriate preclinical model for the particular research question, and distinctive characteristics should be regularly checked to ensure that the reliability of the model is maintained during extended passaging. In general, prostate cancer PDX characteristics remain relatively stable during serial passage in mice [22, 54, 55].
Inter-patient and intra-tumoral heterogeneity in prostate cancer highlights the need for a broad collection of models to represent the genetic diversity of these tumors at different stages of the disease. Spatial heterogeneity and temporal evolution of clonal composition may be accounted for by taking multiple samples of a patient’s tumor, from different foci within the prostate or from different metastatic lesions or at different time points during the course of disease. Kohli et al. developed a series of PDX models from needle biopsies of a rib metastasis from a prostate cancer patient collected before and after treatment with enzalutamide and found that the PDXs preserved with high fidelity the patient’s genomic and transcriptomic alterations [56]. Despite the obvious ethical and practical hurdles, in collecting multiple samples from metastatic lesions at different time points during treatment and establishing prostate cancer PDXs from needle biopsies, this encouraging report demonstrates that modeling clonal evolution during disease progression is feasible using PDXs.
In summary, prostate cancer PDX models reproduce the main characteristics of patient tumors with regard to tissue architecture, genetic alterations, biomarker expression, as well as response to androgen deprivation. Major biomarkers like AR and PSA are essential characteristics that need to be maintained also in late-stage disease to adequately reflect AR-positive progressive castration-resistant prostate cancer (CRPC).
Tumor Micro-environment in Prostate Cancer PDX Models
It is well-recognized that the tumor micro-environment plays a crucial role in regulating tumor growth and metastatic potential of cancer cells. The tumor micro-environment involves the extracellular matrix (ECM) and includes fibroblasts, endothelial cells, mesenchymal stem cells (MSCs), macrophages, and other inflammatory cells to form a highly dynamic heterogeneous cell population with distinct functions. Cancer-associated fibroblasts (CAFs), originating from stromal fibroblasts, local progenitors, and infiltrating bone marrow-derived MSCs, support tumor progression by expressing growth factors and promoting epithelial-mesenchymal transition (EMT) [60]. The ability to adapt the stromal compartment and reprogram fibroblasts into CAFs is vital for the tumor cell to influence and modulate its micro-environment, making it permissive to tumor growth, survival, invasion, and metastasis [61–64]. In PDX models, human stromal cells are lost shortly after subcutaneous tumor engraftment in athymic mice, being replaced within the first mouse passages by murine stroma [65]. The loss of human stroma is considered a major limitation of the PDX model. However, our current understanding of EMT dynamics and tumor-stroma interplay questions the need for human stroma and whether murine fibroblasts cannot be educated to become CAFs with similar properties and function. Indeed, murine fibroblasts and vasculature can efficiently support the overall structure and growth of the engrafted tumor, and it seems that stably established prostate cancer PDXs contain CAFs that are well capable of reprogramming the host (subcutaneous) stroma (van Weerden, unpublished data). However, a consequence of the replacement of human by murine stroma in PDX models is that interspecies incompatibility may compromise the physiological cross talk between some stromal factors and respective receptors on the cancer cells (and vice versa). Although mice and men share ~83% of homology, some relevant pathways that constitute the intricate tumor-stroma interplay are not reflected in PDX models. For example, species differences have been demonstrated for HGF/cMET and IL6/IL6R, two very relevant pathways implicated in prostate cancer growth and metastasis [66–69].
Clearly, another limitation of PDX models is the requirement for immunodeficient hosts. The lack of a functional immune system has a significant impact on the tumor micro-environment and, obviously, limits the use of PDX models for studies on tumor immunity and immune-modulating therapies. Later in this chapter, we will discuss strategies to tackle this deficiency.
Another determinant aspect in the interaction between tumor and micro-environment is the tissue-specific composition of the stroma and extracellular matrix. Hence, the site of engraftment may influence tumor growth, metastasis, and treatment responses, particularly for drugs directed against tissue-specific targets of the tumor micro-environment. Depending on the research question, orthotopic engraftment into the murine prostate or intraosseous transplantation may be preferable over subcutaneous transplantation. For prostate cancer, the orthotopic model has been especially challenging, not so much because of the small size of the mouse prostate, requiring dedicated microsurgery, but also because of the selection which prostate lobe, dorsolateral or ventral, would be the most appropriate to reflect human prostate tissue. While some research groups inject cells in the ventral prostate for convenience, others argue that based on genomic profiles, the dorsolateral lobe seems to better reflect the human prostate micro-environment [70, 71]. The use of cell line suspensions rather than PDX fragments has also changed the nature of these models into cell line-derived xenograft (CDX) models rather than true PDXs. Although the small size of the mouse prostate makes tumor fragment transplantation troublesome, surgical orthotopic implantation (SOI) was reported to be feasible [72, 73]. Orthotopic models of prostate cancer also demand for alternative methods to accurately monitor tumor-burden plasma PSA may be used as an indicator of tumor burden, although this approach is obviously restricted to PSA-producing xenografts. Transrectal ultrasonography (TRUS) has been established for visualization of the murine prostate [74] (Fig. 8.3). The application of TRUS monitoring of orthotopic prostate cancer has shown to be an excellent noninvasive, reliable, and fast method allowing for intensive monitoring of treatment responses of orthotopic prostate cancer PDXs [75]. New developments in ultrasound imaging include 3D ultrasound combined with photoacoustic imaging that offer longitudinal monitoring of tumor burden as well as displaying tumor vasculature and angiogenesis [76, 77]. Alternative approaches to monitor tumor growth and its micro-environment have been greatly extended by highly dedicated, multimodality small animal imaging applications, including optical imaging using fluorescence and/or bioluminescence and in vivo imaging systems (IVIS), MRI, and PET/SPECT [78]. The transfection of cancer cells with multicolor, more intense fluorophores and the establishment of fluorescently tagged transgenic mice to also visualize the murine environment have significantly contributed to our knowledge of tumor behavior and the cross talk with its micro-environment [79, 80].
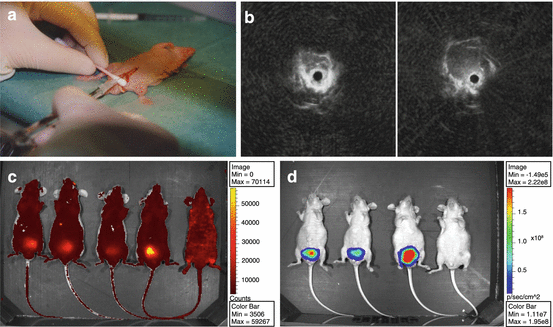
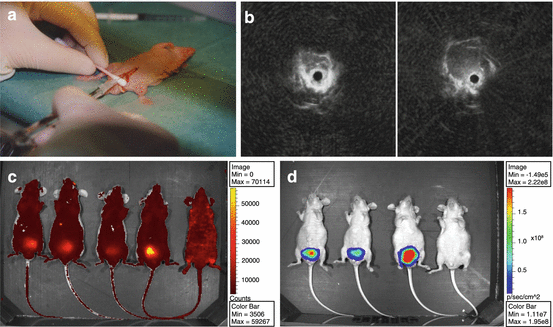
Fig. 8.3
Prostate cancer orthotopic PDX model. (a) Cell injection into the dorsal mouse prostate. Orthotopic tumor growth can be monitored by (b) rectal ultrasonography, using a dedicated mouse rectal ultrasound probe (adapted from Kraaij et al. 2002 [74]); (c) katushka-fluorescence; or (d) Luc2 bioluminescence
Despite some limitations, PDX models of prostate cancer have shown to recapitulate the complexity of the human disease rather well, providing a substantial contribution to basic and translational research. Techniques to reconstitute human stroma, humanize the immune system, and adapt the hormonal status of the host animal are emerging. These and other innovative strategies in the development of prostate cancer PDX models will be discussed in the last section of this chapter.
Applications of Prostate Cancer PDX Models
PDXs in Prostate Cancer Biomarker Research
The introduction of the serum PSA test in the mid-1980s has changed the management of prostate cancer, allowing for early detection when the disease is still curable [81]. A drawback is that physicians are now detecting, and possibly overtreating, insignificant tumors. Furthermore, benign conditions of the prostate may also cause elevated PSA levels, prompting the search for alternative diagnostic biomarkers. Next to screening and diagnostic purposes, serum PSA is being used as a biomarker for monitoring disease progression and response to therapy. However, in the clinical situation, PSA is a modest surrogate of treatment response. Indeed, preclinical studies with the small molecule suramin showed, in the prostate cancer LNCaP CDX model, the inhibition of PSA production without affecting tumor growth, hence illustrating the limited value of PSA as a response biomarker [82, 83]. PDX studies can be applied to directly correlate tumor growth effects to PSA response and assess whether treatments may directly interfere with PSA production and/or release. Hence, PDX-based studies may provide preclinical validation for the use of PSA as a treatment response biomarker in subsequent clinical trials. Indeed, such PDX studies have been used successfully showing the value of its concept [75, 84, 85].
In the last decade, advances in high-throughput genomic and proteomic profiling led to the discovery of novel prostate cancer biomarkers, including PCA3, TMPRSS2:ERG fusion, and AR splice variant 7 (AR-V7) [86]. Other biomarkers emerging in prostate cancer research include circulating tumor cells (CTCs), microRNAs, and exosomes [87, 88]. These novel biomarkers are still under development and need further validation before being accepted and fully implemented in the clinic. PDXs are particularly suitable preclinical models for biomarker discovery and validation because they constitute a pure source of human tumor tissue that is not contaminated with normal cells. Thus, all alterations in transcripts or proteins detected by genomics or proteomics analyses to be human specific are derived from the tumor and are by definition tumor specific. Genome-wide expression analysis of prostate cancer PDX models has led to the identification of diagnostic and prognostic biomarkers/signatures for prostate cancer. For example, Hendriksen et al. used microarrays to analyze genes affected by castration on a panel of 13 prostate cancer PDXs and identified multiple candidate biomarkers for prognosis. The validation in a small cohort of patient samples showed that low mRNA expression of HERPUD1, STK39, DHCR24, and SOC2 in primary tumors was strongly correlated with the development of metastases after radical prostatectomy [89]. Other studies used next-generation RNA sequencing on paired metastatic/nonmetastatic prostate cancer PDXs to identify microRNAs and long noncoding RNAs, as novel biomarkers associated with metastasis [90, 91]. PDXs are also particularly powerful tools for studies of serum biomarkers, since also here it applies that all human proteins or transcripts detected in the serum of the tumor-bearing mouse are derived from the tumor. Prostate cancer PDXs have been used in combination with proteomics techniques to identify human prostate cancer-secreted proteins and exosomes in the serum of xenograft-bearing mice, as potential diagnostic and prognostic serum markers [92, 93]. In another example, Jansen et al. developed an ingenious PDX-based biomarker discovery method to detect low abundant prostate cancer-derived serum proteins and circumvent the dynamic range limitations of standard patient cohort proteomics comparisons [94]. The authors injected serum from PDX-bearing nude (nu/nu) mice in immune-competent (-/+) mice to elicit an antibody response against PDX-derived antigens. These proteins were then identified by probing protein microarrays with serum from the immunized mice and a subset of these potential biomarkers was subsequently validated in serum samples from prostate cancer patients [94].
Predictive biomarkers of treatment response are a developing field in prostate cancer research and becoming increasingly important to identify patients who are most likely to benefit from emerging targeted therapies. A recent study by Beltran et al. illustrated the use of PDX models in the validation of treatment response biomarkers in combination with targeted therapies [95]. Using whole-exome sequencing, the authors detected a novel alteration involving the DNA repair gene FANCA in a patient with aggressive neuroendocrine prostate cancer, who showed a remarkable clinical response to cisplatinum chemotherapy. The authors subsequently established a PDX from a metastatic lesion of this patient, which contained the same gene alteration, allowing a validation of the predictive value of the mutation for cisplatinum response [95].
In summary, PDXs are becoming increasingly important in prostate cancer biomarker research. While reflecting the molecular alterations and phenotypic characteristics of human tumors, prostate cancer PDXs provide representative and versatile models for the discovery and validation of diagnostic, prognostic, and therapy response biomarkers.
PDXs to Investigate Novel Therapies for Prostate Cancer
Until recently, options for medical management of metastatic prostate cancer patients were limited, but the last decade has seen significant advances in the treatment of late-stage prostate cancer with the approval of eight new drugs. Next to the traditional androgen deprivation therapies (ADT), these include the androgen pathway-targeting agents enzalutamide and abiraterone acetate, chemotherapeutics docetaxel and cabazitaxel, bone-targeting agents denosumab and radium-223, and the immunotherapeutic sipuleucel-T [96]. Besides symptom palliation, these novel agents have shown to improve survival in metastatic patients, although resistance to these therapies inevitably develops.
PDX models are valuable tools to test novel drugs for their efficacy, to assess potential interfering pathways, to identify and validate putative tumor biomarkers for response, and to optimize treatment strategies, information that cannot be obtained from in vitro studies. Multiple studies have shown that PDX models may predict drug activity in patients remarkably well and are thus useful to generate confirmation and additional information (see above) before or in parallel to clinical trials [97–101]. For prostate cancer, PDX models have shown to recapitulate the clinical response to androgen-targeting agents and docetaxel and are being used increasingly to test novel-targeted agents in combination therapies, particularly with ADT or docetaxel [22, 54, 56, 59]. For example, the combination of PI3K/AKT-targeting drugs with ADT induced durable tumor regression in PTEN-negative prostate cancer PDXs, as compared to either therapy alone, supporting previous reports of a cross talk between PI3K and AR signaling [85, 102, 103]. These promising PDX-based results have paved the way for multiple clinical trials testing combination therapies that target both these pathways simultaneously (Clinicaltrials.gov: NCT02407054, NCT01251861, NCT02525068, NCT01485861). Similarly, PDX models of prostate cancer are being used to test co-treatment options in order to improve docetaxel efficacy and delay disease progression. Such studies have tested combinations of docetaxel with various compounds, among which are estramustine (chemotherapeutic), trastuzumab (anti-Her2 antibody), or zoledronic acid (bone-directed agent) [104–106]. Another interesting application of PDX models is the optimization of the timing and sequence of the different therapies, in order to delay disease progression, as demonstrated in a study by Dahmani et al. that compared four different sequencing schedules of docetaxel and estramustine in a series of prostate cancer PDXs [104]. Finally, prostate cancer PDX models have also been used to evaluate dietary intervention to attempt to delay prostate cancer progression. For example, dietary polyunsaturated fatty acids, protein restriction, lycopene, and vitamin E have been shown to inhibit tumor growth in the CWR22, LuCaP23.1, and PC346C models, respectively [75, 107, 108].
The currently available sets of PDXs for prostate cancer show significant predictive power for clinical response. In the advent of precision medicine, with a multitude of novel-targeted drugs in the pipeline, PDX models will take an important role in the research and development of personalized therapies.
PDXs to Understand Mechanisms of Therapy Resistance
Despite major advances in treatment, metastatic prostate cancer remains a lethal disease, as resistance emerges inevitably to the therapies being currently offered. Knowledge of the mechanisms driving tumor growth and resistance is crucial for designing rational strategies to delay the onset of resistance and for the development of therapies targeting these resistance pathways. While often cell lines are used to establish the resistance phenotype because of convenience, PDX models may be more relevant to mimic the clinical progression and development of treatment resistance. One strategy is to collect tumor biopsies before the initiation of therapy and, again at the time of treatment resistance, to generate pre- and post-therapy PDX models. This approach heavily relies on the successful establishment of such PDXs, which has been shown to be rather challenging for prostate cancer. Also, post-treatment biopsies are not always easy to obtain or readily accessible. An alternative approach is to use treatment-naïve prostate cancer PDXs and establish drug resistance in vivo by exposing the host animal to a clinical relevant drug scheme. This approach has the advantage of generating paired treatment-naïve and treatment-resistant models, with the same genetic background [59]. Such PDX pairs are very helpful for molecular-profiling studies, to identify mechanisms and markers of resistance, and for subsequent functional studies, to validate these mechanisms and evaluate treatment options for resistance.
For prostate cancer, the research focus has for long been dedicated toward investigating the mechanisms of resistance to ADT. Hendriksen et al. compared androgen-sensitive with castration-resistant PDX models to characterize the adaptation of the androgen receptor pathway during prostate cancer progression [89]. Other studies in prostate cancer PDX models revealed a novel AR mutation and PI3K/AKT activation as mechanisms of resistance to the anti-androgen bicalutamide [109, 110]. Prostate cancer PDXs have also been used to understand the relevance of the significant intra-tumoral testosterone and dihydrotestosterone (DHT) levels that are maintained in castration-resistant tumors and their potential role in castration-resistant growth. Based on these observations, it was hypothesized that castration-resistant tumors might be able to produce their own androgens (de novo steroidogenesis) [111] or to maintain intra-tumoral androgen levels by active conversion of adrenal androgens [112, 113]. These potential resistance mechanisms to ADT motivated the development of inhibitors of CYP17A1, a key enzyme in the steroidogenic synthesis, such as abiraterone and ortenorel. Additional studies in castration-resistant PDXs revealed that resistance to abiraterone was associated with the upregulation of CYP17A1 and AR expression, including constitutively active AR splice variants, suggestive of potential mechanisms of abiraterone resistance [114].
For prostate cancer, chemotherapy is almost exclusively dominated by the successful taxane-based therapies. To allow the investigation of mechanisms of taxane-resistance, the discovery of predictive biomarkers of taxane response, and to fill the lack of in vivo models for taxane-resistant prostate cancer, novel PDX models of docetaxel resistance have been generated. De Morrée et al. established two docetaxel-resistant PDXs from two independent docetaxel-naïve PDXs, by repeated biweekly administration of docetaxel to tumor-bearing mice [59]. Studies of these PDXs revealed that taxane efficacy was determined by the capacity to accumulate sufficient intra-tumoral drug levels and that resistance was directly related to the inability to achieve this [59]. Other PDX studies were applied to understand the reduced efficacy of docetaxel observed in enzalutamide-resistant patients. These studies demonstrated cross-resistance between these two agents, as docetaxel directly inhibited AR activation in enzalutamide-naïve tumors, but not in enzalutamide-resistant tumors [115]. At the same time, the expanding knowledge of the interactions between taxanes and (hormonal) agents also underscore the importance of defining the best treatment sequence and optimal timing of the treatment.
Altogether, these studies show that PDX models of prostate cancer have contributed to our understanding of mechanisms of therapy resistance and are relevant tools to identify and validate potential therapy resistance biomarkers.
PDXs as Translational Tools for Precision Medicine
The “omics” era has had a profound impact in our understanding of the molecular alterations in prostate cancer, leading to the identification of new disease markers and potential therapeutic targets [50, 116]. This knowledge fueled the development of a new generation of targeted drugs, giving rise to the concept of precision medicine, whereby patients are offered personalized treatment tailored to the molecular characteristics of their tumors. To achieve this, it is essential to improve integration between laboratory research and the clinic, through the use of relevant models that accurately reflect the genetic alterations, disease characteristics, and therapy response of the human tumors. Research and pharmaceutical communities are increasingly turning to PDX models, as a way to recapitulate the complexity of human cancers and improve the predictive power of preclinical research.
Co-clinical trials are a novel trend in the development of targeted therapies, in which PDX studies are conducted in parallel with Phase I/II clinical trials [117, 118]. This concept makes use of genetically-defined PDX models to evaluate drug efficacy, determine patient-selection strategies, identify possible resistance mechanisms, and test drug combination modalities, using real-time integration of PDX and clinical data. This combined approach is assumed to facilitate the selection of treatment strategies for further assessment and to accelerate clinical translation. Taking this concept a step further, a pilot co-clinical study was performed generating personalized tumor grafts from 14 patients with different types of cancer, to evaluate 63 anticancer drugs and guide the selection of individualized patient treatments [98]. Considering the low take rate, long latency, and slow growth of prostate tumors in the mouse, personalized PDX models are unlikely to become feasible tools to aid real-time therapeutic decisions of prostate cancer patients. There are few reports of co-clinical trials in prostate cancer. One of these studies investigated the clinical activity of cabozantinib, a MET/VEGFR2 inhibitor, in 21 metastatic prostate cancer patients in a Phase II trial in parallel to a similar study in three prostate cancer PDXs [119]. Tumor responses in the PDX models closely mimicked the response observed in the patients. The integration of the functional data from the PDX studies brought novel insights into the mechanism of action of cabozantinib, identified potential mechanisms of therapy resistance, and allowed an investigation of the impact of dosing schedules on cabozantinib efficacy [119]. The previously-mentioned study, by Beltran et al, where a PDX was established from the metastatic lesion of a prostate cancer patient to investigate the biological role and predictive value of FANCA deletion on cisplatinum sensitivity, provides another example on how PDX models may complement clinical data [95].
Altogether, these studies highlight the potential of integrating PDX-based studies with clinical trials to predict efficacy of novel-targeted agents, investigate mechanisms of drug sensitivity/resistance, and develop patient-stratification strategies, accelerating clinical translation into personalized therapies. To achieve this, access to a broad panel of PDX models representing the range of molecular alterations occurring in prostate cancer is crucial. Such extensive PDX cohorts are currently being assembled, characterized, and annotated to meet this need.
Challenges and Future Directions
Modeling Inter-Patient Heterogeneity: PDX Clinical Trials
The prediction of clinical efficacy and identification of factors that underlie heterogeneous patient responses are highly relevant for adequate screening and selection of potential candidate therapeutics [120]. To guide screening methods and enhance our ability to predict clinical responses, a novel concept was suggested by Gao et al. [97]. Using an extensive PDX collection, containing ~1000 models with a diverse set of driver mutations, a large-scale in vivo compound screen was conducted. This so-called PDX clinical trial (PCT) approach was conducted based on the “one animal per model per treatment” (1 × 1 × 1) model to reflect inter-patient response heterogeneity and assess the population responses to various treatments. This novel preclinical concept was demonstrated to be reproducible and reflected, retrospectively, clinical translatability by identifying associations between genotype and drug response, as well as with established mechanisms of resistance [97, 100, 121]. Such an approach clearly requires an extensive set of genomically- characterized PDXs to fully capture the diversity of the disease, a demanding task and major challenge to apply for prostate cancer.
Interaction of Tumor Micro-environment: Making the Mouse a Hospitable Host
The lack of human stroma is considered a major limitation of PDX models. While murine stroma quickly infiltrates the tumor graft, taking over the function of its human counterpart in supporting the overall structure and growth of the tumor, the engraftment site and interspecies compatibility may compromise the interaction between tumor and host micro-environment.
As discussed earlier, orthotopic PDX engraftment in the mouse prostate or in the bone can be used to replicate the micro-environment at the natural sites of local and metastatic prostate cancer, respectively. Advancements in small animal imaging techniques provide noninvasive methods for monitoring of tumor growth and spread and for visualization of the micro-environment [72, 78, 79].
Furthermore, innovative strategies are being developed to reconstruct species-specific interactions in PDX models. These include co-engraftment of patient-matched stroma components or in vitro-expanded human CAFs, and the transplantation of tissue-engineered humanized bone constructs, to serve as homing site for human prostate cancer cells [65, 122, 123]. In addition, humanized mice are being engineered to compensate for species differences in relevant paracrine growth factors and cytokines. Although little is known about the factors involved in the cross talk between tumor and micro-environment and the role of each of these interactions on prostate cancer growth and progression, it is realized that species differences on relevant pathways may hamper a true reflection of the physiological epithelial-stromal interaction within a PDX. This is the case for HGF/cMET and IL6/IL6R, two pathways implicated in CRPC growth and metastasis [66–69]. Engineered SCID mice expressing human HGF have been generated, allowing the investigation of the HGF/cMET axis in relevant PDX models [124]. Also, a humanized IL-6 ligand receptor system has been introduced in mice, although it involves C57BL/6J immune-competent mice and the system still needs translation to immune compromised strains in order to be applicable to PDX models [125].
From Immunocompromised Mice to Humanized Immune System
Inherent to the PDX system is the lack of a functional immune system. With the realization of the important role of the immune system in the regulation and complexity of the tumor micro-environment, and hence in tumor growth and progression, efforts were undertaken to develop humanized mouse models with a functional immune system. NSG or Rag2-/-IL2rγ-/- triple-negative immunodeficient mice, characterized by profound immunological defects in both innate and adaptive immunity, have been implanted with human hematopoietic stem cells to create a humanized immune-competent tumor micro-environment. The humanized model is based on engraftment of CD34+ human hematopoietic stem and progenitor cells (HSPCs) isolated from cord blood, bone marrow, or fetal liver and injected intravenously into irradiated immune-deficient mice. Here human T- and B-cells develop from human stem cells engrafted in the mouse, which are tolerant of the mouse host due to negative selection during differentiation into T- and B-cells. An alternative humanization model uses freshly isolated leukocytes, from human peripheral whole blood or spleen, for intravenous or intraperitoneal injection into immune-deficient mice recipient. Because the transferred lymphocytes are functionally mature, this model allows for fast evaluation of immune function, although only for relative short-term (weeks) studies [126, 127]. These humanized models develop a functional human immune system, characterized by T-cell maturation and T-cell-dependent inflammatory responses. NSG mice reconstituted with human immune cells and inoculated with prostate cancer PC-3 cells indeed demonstrated infiltration with tumor-infiltrating lymphocytes (TILs) that were able to affect tumor growth [128]. Since interspecies differences in the specificity of growth factors and cytokines represent a serious hurdle when constructing a human immune system in immunodeficient mice, current developments are directed towards genetically introducing essential human cytokine genes in these mouse models [129].
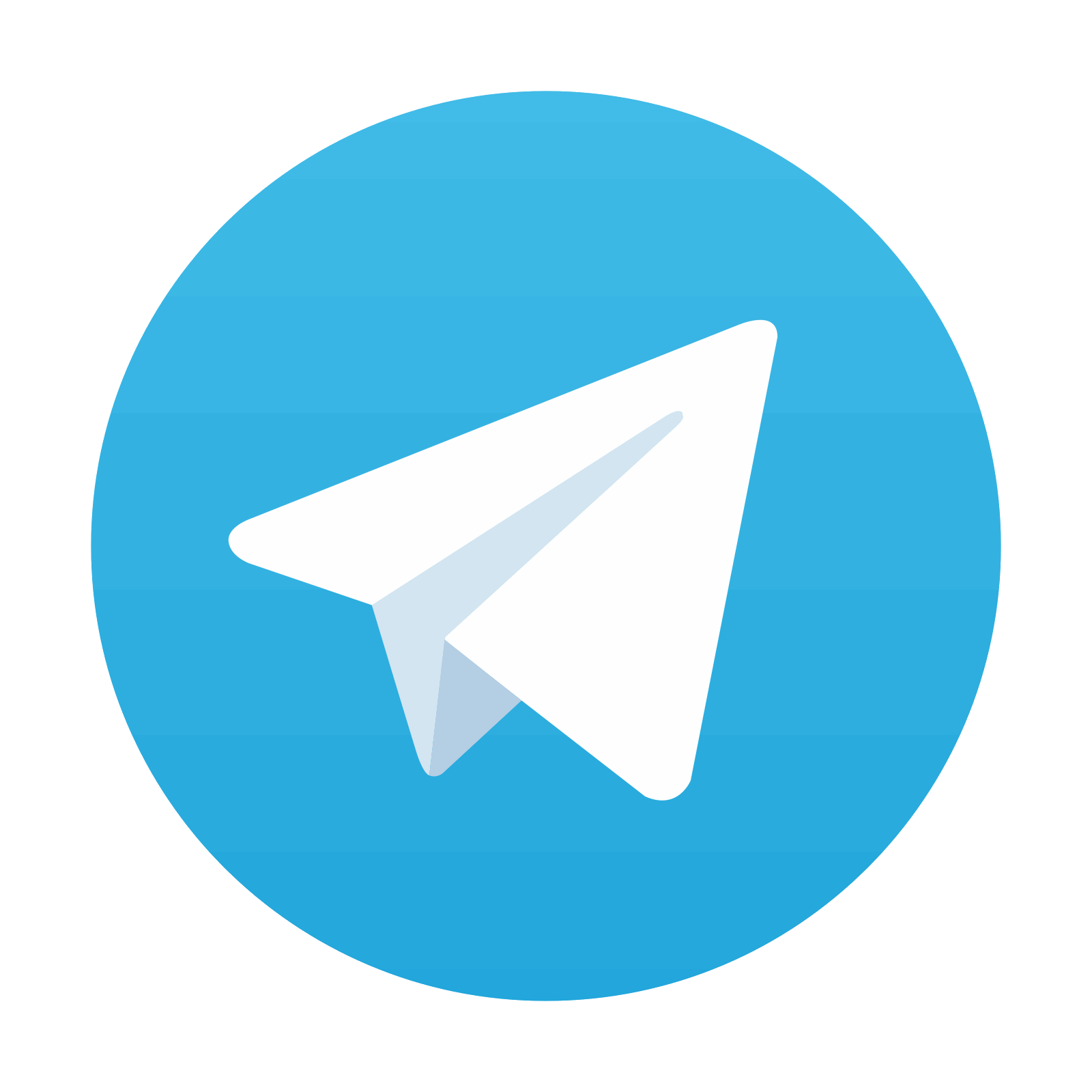
Stay updated, free articles. Join our Telegram channel

Full access? Get Clinical Tree
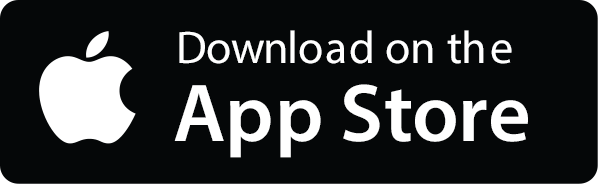
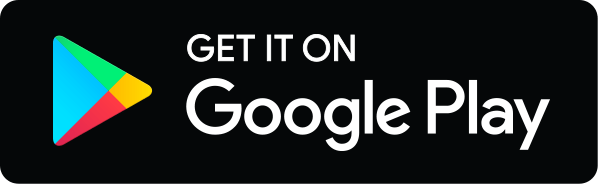