© Springer International Publishing AG 2017
Yuzhuo Wang, Dong Lin and Peter W. Gout (eds.)Patient-Derived Xenograft Models of Human Cancer Molecular and Translational Medicine10.1007/978-3-319-55825-7_11. Patient-Derived Tumor Xenografts: Historical Background
(1)
Vancouver Prostate Centre, Vancouver, BC, Canada
(2)
Department of Urologic Sciences, Faculty of Medicine, University of British Columbia, Vancouver, BC, Canada
(3)
Department of Experimental Therapeutics, BC Cancer Research Centre, Vancouver, BC, Canada
(4)
Living Tumor Laboratory, BC Cancer Agency/Vancouver Prostate Centre, Vancouver, BC, Canada
Keywords
Patient-derived xenograftTumor graftImmune-deficient miceAbbreviations
NOD
Non-obese diabetic
PDX
Patient-derived xenograft
SCID
Severe combined immunodeficiency
A Point on Terminology
Given the extensive history of cancer, the history of patient-derived xenograft (PDX) models is also difficult to fully recapitulate. In particular, this task is complicated by the irregularity with which PDX models were designated. The current use of “PDX cancer models” is a relatively recent addition to the lexicon. However, the general concept of PDX models—i.e., the transplantation of human cancers into animal models—can be found throughout the chronicles of cancer research. However, it wasn’t until the discovery of host immunity and its crucial role in graft survival that the idea of serial transplantation could be realized. Therefore, in order to better reflect the nature of its history, the term “human tumor models” will be used in place of “PDX models,” except when appropriate. Finally, it is important to also mention the existence of the other labels that have been used, including, but not limited to, “human tumor xenografts,” “xenopatients,” “heterotransplant tumor models,” “heterotransplanted human tumors,” and “transplantable tumor models.”
A Brief Historical Context
Concurrent with the initial observation of cancer emerged a need to recapitulate tumors for further observation and experimentation. The idea of what is now known to be a PDX tumor model is composed of two general concepts: “xenotransplantation” (i.e., the transplantation of tissue into a foreign species) and the general use of animals for medical research. Although PDX technology may seem to be a more recent innovation, recapitulating a human cancer within an animal model has been a long-standing goal of cancer research. Such attempts at producing a human cancer model have been underway for centuries, beginning with the first recorded experiment in 1775 by the French surgeon Peyrilhe, who injected extracts of a human breast cancer into a dog [1]. Although Peyrilhe’s experiment ultimately failed (“At length my maid, disgusted by the stench of the ulcer, and softened by the cries of the animal, put an end to his life, and thus prevented my observing the ultimate effects of this disease”), similar efforts to transplant human tumors into various animal models have since been continuously in progress.
Early Attempts
In an effort to learn about the origin of cancer, early scientists attempted to induce spontaneous tumor formation by a method termed “cancer genesis.” They employed a variety of methods to “irritate” or “misplace” cells, with the ultimate goal of generating cancers in otherwise healthy tissue [1]. Although the majority of these experiments ended in failure, these attempts eventually culminated in the successful transplantation of human cancer into animals [2].
Following Peyrilhe’s attempt in 1775, pioneering oncologists began to conduct experiments in order to determine the transmissibility of cancer. These experiments were largely motivated by a sense of curiosity, in that scientists were initially interested in transplanting cancers into animals for purposes of observing the pathology of this otherwise unknown disease. However, more than a century was to pass before a consistent method of tumor transplantation could be achieved. Among the many initial attempts were those made by Dupuytren, Langenbeck, and Lebert and Follin. In 1807, Dupuytren attempted a variety of methods in order to transplant a human cancer into an animal, including “[feeding] animals with cancerous material, [introducing] it into the abdomen, [injecting] cancer juice into the peritoneal cavity and the veins, and [inoculating] the pus of an ulcerated cancer” [1]. In 1840, Langenbeck attempted a similar method by injecting a dog with fluid from a human medullary carcinoma of the humerus. However, in Langenbeck’s case, several round nodules were found in the lung 2 months following the inoculation [1]. Then, in 1851, Lebert and Follin injected emulsified mammary cancer into the jugular vein of a dog. Although the animal died 15 days later, the autopsy found nodules along the wall of the heart [1]. Unfortunately, in both cases, it was unknown whether the observed nodules had occurred as a direct result of successful tumor engraftment or was due to spontaneous tumor formation. With regard to Langenbeck’s experiment, the pathologist Virchow had observed the nodules to more closely resemble spontaneous cancer of dog rather than human origin [1, 3]. From there, scientists began to slowly make incremental advancements toward the generation of human cancer models. Of particular note, in 1938, Greene reported the successful engraftment of human uterine adenoma and adenocarcinoma in rabbits, which was then serially transplanted for several subsequent generations [4]. Furthermore, Greene later followed with the successful transplantation of human tumors into the eye of a guinea pig, rabbit, and mouse [4–8].
Concurrent with attempts to transplant human cancers, scientists also began to examine the morphology of the graft and surrounding tissue. In 1890, Klebs studied fragments of a human carcinoma engrafted into the peritoneal cavities of white rats and found the epithelial constituents of the original graft to have disappeared by the third day following engraftment [1, 3]. Jensen also monitored the outcome of transplanted tumors and observed stromal changes and the disappearance of connective tissue cells within the central region of the original graft [1]. Additionally, Jensen reported the formation of many blood vessels and the appearance of fibroblasts around the tumor and within the margin [1].
Finally, scientists looked toward refining the transplantation technique and streamlining the procedure. In order to do so, a variety of factors were taken into evaluation, including engraftment technique, dosage (e.g., type and origin of the inoculate, frequency, and location), site of engraftment, and environmental conditions (e.g., temperature and environment). Furthermore, many experiments were also conducted in order to examine transplantation efficiency in different hosts, by implanting tumors into rats, the cheek pouch of hamsters, dogs, and chicken embryos [1, 9–13].
In 1912, Murphy reported the successful serial growth of the Jensen rat sarcoma in a chicken embryo [5, 14, 15]. However, much to his surprise, attempts to further propagate the tumor into adult chickens were not found to be similarly successful. With regard to this differential transplantation success between the chick embryo and the adult chicken, Murphy hypothesized that “[the embryo] either provides a food substance utilizable by these tissues, which is lacking in the adult, or else lacks a defensive mechanism against such an invasion, which is possessed by the adult” [16]. Although we now know the reason to be the latter (i.e., host immunity), this experiment (alongside several others—including another of Murphy’s involving the transplant of Rous chicken sarcoma into a chicken embryo) had several important implications [16]. Most importantly, these observations helped to guide researchers toward careful considerations of the animal host. Namely, questions arose that would later crystallize into (and/or were resolved by) theories regarding host immunity and graft rejection.
One scientist in particular, who made significant contributions toward the development of human tumor models (and ultimately PDX models), was Dr. Helene Wallace Toolan. From the Sloan-Kettering Institute for Cancer Research, Toolan was highly prolific during the 1950s in her research into the transplantation of human tumors into laboratory animals, particularly with regards to methodology and technique. Most significantly, in 1951, on the basis of the results from Murphy [16], Toolan devised a protocol for the irradiation of rats and mice prior to transplantation [17]. Observing positive evidence of successful engraftment, Toolan proceeded to successfully transfer the tumors for several generations using X-irradiated and/or cortisone-treated rats and hamsters [18]. Ninety out of the 101 human tumors were reported to successfully survive and proliferate in the treated hosts, thus demonstrating the feasibility and efficacy of Toolan’s method [14, 18, 19].
By addressing the observations and speculations of Murphy, Toolan had effectively demonstrated the essential role of host immunity in transplantation success. Accordingly, the use of chicken embryos and the cheek pouch of hamsters (an immunologically privileged site) was beneficial in the sense that the pretreatment of conditioning agents was not necessary [2]. However, a comparative study examining the transplantation of human tumors into animals and eggs and as a tissue culture demonstrated the conditioned animal to be the most effective host for establishing and maintaining tumor growth [20]. According to the study, certain characteristics (such as the rate of growth) can vary depending on the medium in which the human tumors are grown. For example, the human epidermoid carcinoma designated H. Ep. #3 was reported to be highly virulent and metastatic when implanted into the animal or onto the egg but was observed to be “delicate” in tissue culture [20]. Conversely, H. Ep. #1 and H. Ep. #2 were found to grow relatively slowly within the conditioned hamster or rat, or on the egg, but grew in “gallon lots” as a tissue culture. However, when transplanted back from the tissue culture into an animal host, the cancers were found to revert back to a slower and more indolent pace of proliferation [20].
Despite the documented success of using a conditioned animal host for the transplantation of human tumors, there were several caveats to preclude their continued use. For the most part, this was due to a shift in the intended application of human tumor models. As society’s knowledge and understanding of cancer progressed, scientists began to consider the possibility of using human tumor models as a platform for screening possible anticancer agents. As a result, the preconditioning of animal hosts did not provide the ideal experimental conditions. Palm et al. emphasized the importance of minimizing the influence of conditioning agents from the experimental design and cautioned against possible “ambiguous and/or misleading information” resulting from the conditioning agents rather than the experimental agent [21]. Furthermore, Palm et al. expressed concerns with regards to “spurious antitumor effects caused by less than optimum host conditioning,” which he thought would conceal and/or dilute the effects of the potential therapeutic agents. Thus, it became imperative to find an alternate method of subduing host immunity.
For the most part, in the initial years following the first successful transplantation of human tumors into laboratory animals, mice were largely neglected as hosts in favor of rats and hamsters. However, the rationale for the use of mice as experimental models had existed since the 1900s. According to Woglom, the use of mice was motivated by “the ease with which [mice] can be procured and the cheapness and facility with which they can be can kept under observation.” Furthermore, Woglom asserted that the “subcutaneous location of its tumors [makes] for early and ready recognition” [1]. Therefore, by acknowledging and recognizing the benefits associated with mouse models, Gallily and Woolley looked toward the production of mouse-grown human tumor models for the evaluation of “anticancer drugs, chemicals, and regimes” [4]. These models were produced via the serial transfer of H. Ep. #3 into treated and/or conditioned mice. Additionally, Gallily and Woolley investigated the heterotransplantability of the H. Ep. #3 tumor by testing different inoculation sites, which included subcutaneous, intramuscular, and intraperitoneal sites. As a result, the experiments conducted by Gallily and Woolley formed an important component of early efforts toward producing what would eventually be recognized as a PDX collection.
Establishing PDX Cancer Models Using Immune-Deficient Mice
However, the early success rate of PDX establishment is extremely low, which is largely due to the rejection of grafts by the host immune system. Although some methods such as X-ray irradiation and thymectomy have been used to suppress the host immune system [22–24], advances in the development of PDXs largely benefited from the generation of immune-deficient hosts (mice).
The first breakthrough was the development of nude mice. In 1962, Isaacson and Cattanach were the first to report a mutant mouse (BALB/c nu/nu) suitable for xenografting of human cancer tissue [25]. These mice have the Foxn1 mutation, are athymic, and therefore lack the functional T cells [26]. However, natural killer (NK) cell activity is slightly increased, and the humoral antibody response system is only partially impaired in these mice. A number of PDX cancer models were established by grafting various types of cancer tissues into nude mice [24, 27, 28]. Nude mice are still commonly used as hosts for xenotransplantation of human tumors. Although the take rate of immortalized cell lines in nude mice ranges from 50 to 100%, the take rate of tumor tissue implants is generally low and varies largely among tumors of different origins.
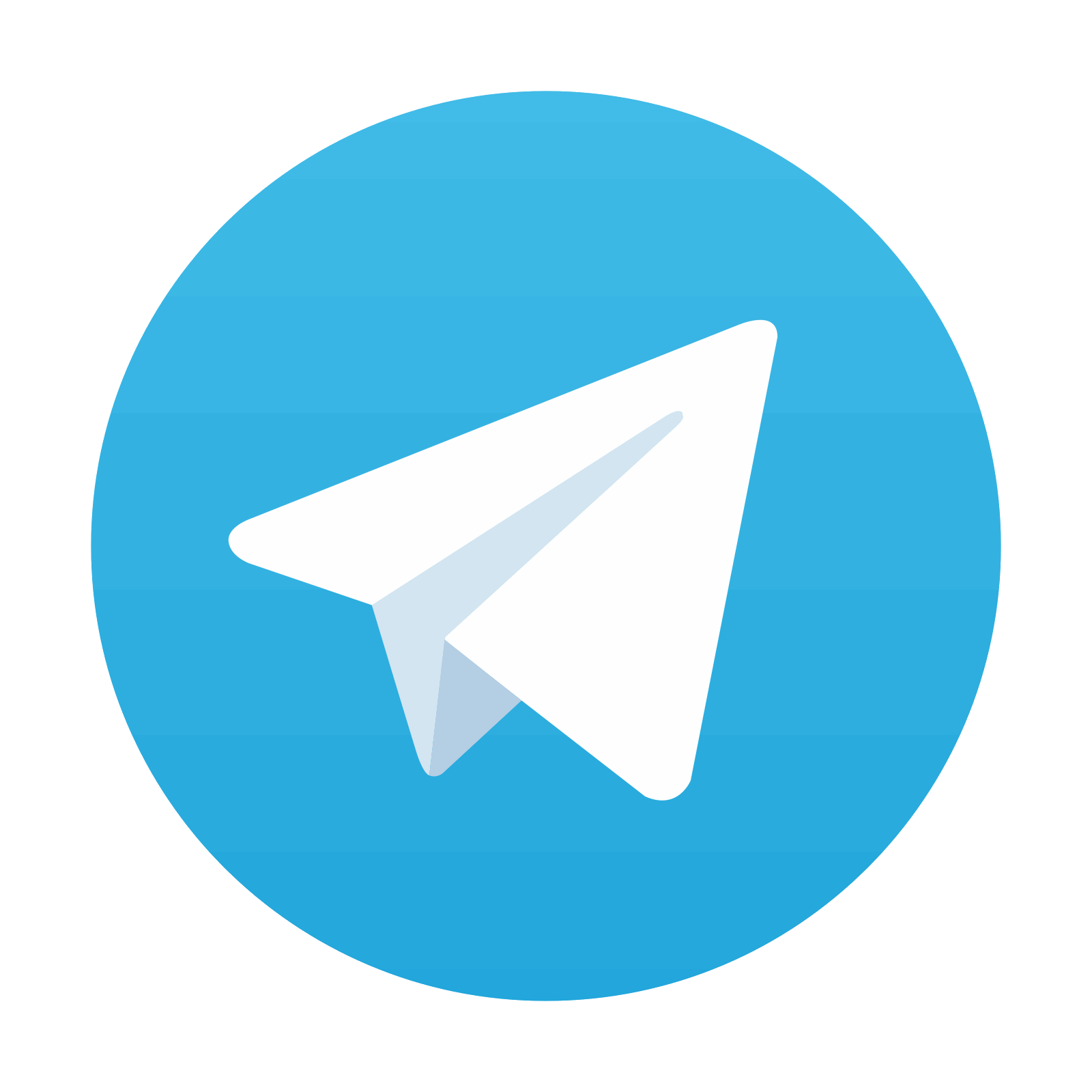
Stay updated, free articles. Join our Telegram channel

Full access? Get Clinical Tree
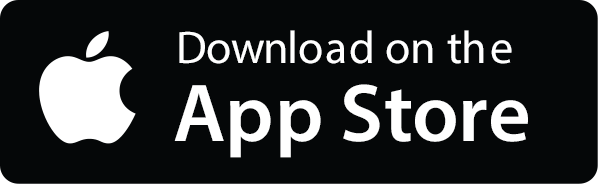
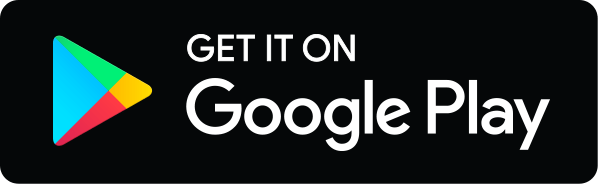