The prevalence and incidence of diabetes mellitus (DM), especially type 2 DM (T2D), is increasing worldwide. DM contributes to a high burden of disease-associated complications and comorbidities, with vascular complications representing the leading cause of morbidity and mortality in patients with diabetes. These affect both small and large blood vessels, that is, microvascular and macrovascular beds, respectively.
The microcirculation is the terminal vascular network of systemic circulation consisting of microvessels with diameters <150 μm (arterioles, capillaries, and venules) [ ]. Vessels of the microcirculation are almost entirely lined by the endothelium, a cellular monolayer of endothelial cells (EC) lining the blood vessel. The endothelium is the orchestral conductor of blood vessel function. Thus, EC in symbiosis with smooth muscle cells regulates the microvascular blood flow predominantly by regulation of the tone of arterioles guy [ ] Fig. 10.1 . Additionally, the endothelium plays a critical role in maintaining multiorgan health and homeostasis. Endothelial functions include the regulation of vascular tone by the production of vasodilators, including nitric oxide (NO) and prostacyclin (PGI2), and vasoconstrictors like endothelin-1 (ET-1), angiotensin II, and reactive oxidative species (ROS) [ ]. Other functions involve angiogenesis (neovascularization of hypoxic tissue), hemostasis, and the provision of an antioxidant, anti-inflammatory, antithrombotic interface, nutrient, and electrolyte uptake, to name only a few [ ]. The inability of EC to perform their physiological function (a condition known as EC dysfunction) presents with impaired endothelium-dependent vasodilation, heightened oxidative stress, chronic inflammation, leukocyte adhesion, hyperpermeability, and EC senescence. Angiogenesis and EC dysfunction are defining features of various diseases such as DM, hypertension, obesity, and dyslipidemia [ ].

Microvascular complications in diabetes are mostly determined by hyperglycemia, although significant roles are also played by high blood pressure and hyperlipidemia, especially in T2D [ ]. Indeed, blood glucose control is tightly associated with the risk of developing microangiopathy, since several clinical trials have clearly shown that chronic elevation of blood glucose, as measured by HbA1c levels, is associated with microvascular complications. Moreover, the United Kingdom Prospective Diabetes Study (UKPDS) trial and other prospective studies with a 10-year follow-up have shown that intensive glucose therapy is associated with a reduced risk of clinically evident microvascular complications and death from any cause [ , ]. Results from the Diabetes Control and Complications Trial (DCCT) and the UKPDS trials have clearly established that hyperglycemic injury is the primary causal factor behind microvascular damage. Hyperglycemia promotes microvascular damage through at least five main mechanisms elegantly reviewed by Michael Brownlee [ ]: (1) an increased glucose flux and other sugars through the polyol/aldose reductase pathway; (2) an increased intracellular advanced glycation end products (AGEs) formation; (3) interaction between AGEs and their receptors (termed RAGEs), leading to intracellular signaling which disrupts cell function [ ]; (4) a persistent activation of protein kinase C (PKC) isoforms [ ]; and (5) an increased hexosamine pathway activity [ ]. According to Brownlee, this single hyperglycemia-induced process involves the overproduction of superoxide by the mitochondrial electron transport chain. Production of ROS has been discovered as a common feature of all cell types damaged by hyperglycemia. In this sense, the increased production of superoxide by the mitochondrial electron transport chain, which decreases a key glycolytic enzyme termed glyceraldehyde-3 phosphate dehydrogenase (GAPDH), has been proposed as a causal link between elevated glucose and each of the previously mentioned pathways responsible for hyperglycemic damage [ ]. Although in recent years the mitochondrial superoxide generation hypothesis in diabetes complications has been questioned, there is evidence to show that mitochondrial function and excess cellular energy production are critical mediators of diabetes complications [ ].
Diabetes-induced endothelial dysfunction is a critical and initiating factor in the genesis of diabetic vascular complications. All these altered molecular pathways in endothelial dysfunction are related to excesses or defects in angiogenic processes. The effects of hyperglycemia on the microvasculature can lead to excessive neovascularization, as in proliferative diabetic retinopathy [ ] and nephropathy [ ] or attenuated neovascularization, contributing to impaired coronary collateral vessel development [ ], impaired wound healing [ ], and transplant rejection in diabetic recipients [ ]. The concurrent existence of pro- and antineovascularization responses in diabetes is known as the “angiogenesis paradox.” The name “angiogenesis” (or neoangiogenesis) refers to the growth of new vessels from existing microvasculature [ ] caused by changes in the growth state of EC and an imbalance between pro- and antiangiogenic factors, with the proangiogenic factors as the predominance. Angiogenesis is a tightly orchestrated process, which involves EC proliferation, migration, invasion, and new tube formation, proangiogenic molecules, for example, tumor necrosis factor-alpha (TNF-α), interleukin-6 (IL-6), or vascular endothelial growth factor (VEGF), and antiangiogenic factors, for example, serpins. If the proangiogenic molecules dominate, the angiogenesis is dysregulated, and the endothelium becomes dysfunctional. The alterations of angiogenesis associated with diabetes are extensive and given that diabetes is a multisystem and a multiorgan disease, we might expect that angiogenesis abnormalities are present in essentially all organs and tissues of patients with diabetes.
Besides EC, there is another group of cells called pericytes that are part of blood vessels and are also important in the physiopathology of microvascular damage. Pericytes are contractile mural cells that play a main role in the normal structure and function of microvessels by supporting and stabilizing the microvascular structures that are present in all vascular beds [ ]. Pericyte-EC crosstalk is essential in both remodeling and quiescent vasculature. Their detachment from EC causes vascular instability. Pericyte loss is believed to be an early hallmark of diabetes-associated microvascular disease, including retinopathy and nephropathy [ ]. In microvascular diabetic complications, such as retinopathy and diabetic nephropathy, apoptosis of pericytes as well as migration of these cells away from the capillary have been reported [ ].
On the other hand, there is clear evidence of a clinical relationship between micro- and macrovascular complications, which has led to the hypothesis of a possible pathogenic link between them [ ]. Accumulating data from epidemiological and pathophysiological studies suggest that these vascular problems are, in fact, closely interrelated. In this sense, randomized, cross-sectional, and epidemiological prospective studies have demonstrated that microvascular complications in patients with diabetes independently predict cardiovascular complications. Having microvascular complications increases by 18% the risk of a composite endpoint of cardiovascular death or heart failure hospitalization [ ] and also increases the risk from 32% as compared to none to 99%, respectively [ ]. The presence and degree of microvascular complications have also been found to be associated with subclinical atherosclerosis [ ]. A “common soil” for the development of micro- and macroangiopathy diabetic complications has been proposed [ ].
The most prevalent and studied microvascular complications in subjects with DM include eye disease or “retinopathy,” kidney disease termed “nephropathy,” and neural damage or “neuropathy.” Besides these classical target organs of microvascular disease, in recent years, other unforeseen target organs of diabetes-induced microvascular damage have been reported such as the arterial wall, the brain, the skin, the bone, the heart, and the lung Fig. 10.2 .

1
Microangiopathy in nonclassical target organs and tissues in subjects with DM
1.1
Arterial wall of large arteries
The walls of the large arteries receive their nutrient and oxygen supply from the lumen and also from small vessels located in the adventitia called vasa vasorum (VV), whose main stimuli for proliferating are hypoxia and ischemia [ ]. Several authors have hypothesized that microvessels of large arteries are an important target site for diabetic microangiopathy, similar to what is observed in other classical organs, supporting the concept of a generalized microangiopathy [ , ]. In both subjects with type 1 diabetes (T1D) and T2D without clinical cardiovascular disease, using contrast-enhanced carotid ultrasound imaging, increased angiogenesis of the VV in the common carotid artery has been described [ , ]. In subjects with T2D, this increased signal of VV is further increased in subjects with retinopathy [ , ]. On the other hand, this microangiopathy of the large arteries has been suggested to be a substrate of the diabetic atherosclerotic process [ ].
It is known that atherosclerotic plaque rupture with luminal thrombosis is the most common mechanism responsible for the majority of acute coronary syndromes and sudden death. Intraplaque hemorrhage is considered a critical factor in atherosclerotic plaque growth and unstabilization. It is believed that this occurs from leaky VV that invades the intima from the adventitia as the intima enlarges [ ]. In this sense, plaque neovascularization and adventitial VV are reported to be associated with plaque severity and cardiovascular events [ ]. Histological examinations of coronary arteries and aorta suggest that atherosclerotic plaques show a higher density of microvessels in patients with diabetes than in those without this condition [ , ]. On the other hand, other authors have reported reduced density of VV in the arterial wall in a hyperglycemic mouse model of atherosclerosis [ ], as well as in coronary arteries of deceased individuals in which VV reduced density has been described to be lower in those with diabetes with higher HbA1c levels [ ]. Differences concerning the density of VV in previously mentioned studies might be explained by the fact that the effect of hyperglycemia on the vasculature may be dependent upon the tissue/organ being considered as well as the duration of the hyperglycemia reflecting different evolutionary stages of diabetes. For example, an early complication of diabetes is preproliferative diabetic retinopathy, involving pericyte and EC death leading to retinal ischemia, whereas excessive retinal neovascularization or proliferative diabetic retinopathy is a common complication in more advanced diabetes [ ].
It has been suggested that the VV present evolutionary changes similar to those observed in the retina: an initial stage in which endothelial dysfunction and loss of capillaries predominate [ ], and more advanced stages in which ischemia plays a key role, leading to angiogenesis and inflammation in response to the progressive enlargement of the necrotic core within the plaque [ ]. This change in plaque phenotype results in a more inflamed and unstable plaque, favoring plaque rupture and a poor outcome of cardiovascular events.
1.2
The brain
Subjects with diabetes show an increased risk of ischemic stroke. This is observed both in subjects with T1D [ ] and T2D [ ] and can be attributed to both large and small vessel involvement. Importantly, cerebral small vessel disease (SVD), which includes lacunar infarctions, white matter lesions, and cerebral microbleeds, has been reported to be associated with stroke incidence and with the development and progression of dementia [ ]. This disease is usually assessed and characterized by MRI. In subjects with T2D, an increased prevalence of lacunar infarcts has been described compared with subjects without DM [ , ]. Among them, those with diabetic retinopathy have an increased burden of SVD compared with those without retinopathy [ ]. In subjects with T1D, the prevalence of SVD, in particular cerebral microbleeds, has also been reported to be increased compared with healthy control subjects [ ]. Similar to what has been described in subjects with T2D, in subjects with T1D, diabetic retinopathy, more specifically in its proliferative stage, has been reported to be associated with cerebral microbleeds [ , ]. In these subjects, the presence of proliferative diabetic retinopathy together with SVD is associated with a reduced skin capillary density, which points to a process of a generalized microangiopathy.
The data obtained in the previously mentioned studies suggest that retinal microvascular abnormalities might be pathophysiologically linked to ischemic cerebral SVD supported by the knowledge that the microvascular bed of the retina mirrors the small cerebral vessels concerning their embryological origin, anatomical features, and physiological properties [ ].
1.3
The skin
The skin microvasculature plays a crucial physiological role in the body’s defenses to protect the keratinized epithelium of the epidermis. The thickening of the basement membrane (BM) and loss of pericyte coverage caused by diabetes can compromise the skin barrier and allow microbe migration [ ]. In addition to structural changes, vascular dysfunction due to changes in the microvascular perfusion as the result of diabetes, hyperglycemia, and/or insulin resistance has also been described [ ]. Studies in diabetic foot patients showed an impaired vascular hyperemic response to skin heating or minor trauma and a reduced reaction to hypoxia. These alterations were not only present in the foot skin but also in forearm skin, an area that is not usually affected by diabetes neuropathy. Moreover, in diabetes, lower extremity skin has lower tissue oxygen saturation and high-energy phosphate reserves compared with controls, and this worsens if neuropathy is present [ ].
Skin microvascular damage in diabetes can also lead to deficiency in wound healing, in both acute and chronic wounds. Experimental models suggest that diabetes impairs the endothelial progenitor cell proliferation and subsequent recruitment to injury sites by inhibiting nitric oxide generation [ ].
To evaluate dermal capillaries and thus microvascular damage in the skin, the most used technique is the nail-fold video-capillaroscopy. This noninvasive method evaluates finger capillary abnormalities that are considered surrogate markers for generalized microvascular function [ ]. It is also used for the identification of microvascular patterns in many rheumatic diseases, particularly systemic sclerosis [ ]. Finger capillary abnormalities have been described in subjects with T1D and T2D and reported to directly correlate with the presence of diabetic retinopathy [ ] and neuropathy [ ]. Moreover, in subjects with T1D and proliferative retinopathy, lower skin capillary perfusion is associated with lower cognitive performance over time [ ].
1.4
The bone
The structural integrity of bone marrow has been recognized to be altered in diabetic conditions. In individuals with T2D, bone marrow microangiopathy is associated with inflamed fat accumulation and apoptotic reduction of CD34+ hematopoietic stem/progenitor cells [ ]. It is now accepted that bone marrow plays a fundamental role in the vascular regenerative process. The reason is that endothelial progenitor cells derived from bone marrow have proangiogenic activity. These cells are mobilized following a gradient of growth factors and chemokines released by ischemic or injured tissues and differentiate into ECs. They support vascular growth mainly by secreting proangiogenic factors [ , ].
Reduced circulating endothelial progenitor cell levels have been described in diabetes and are related to the incompetence of the bone marrow of patients with diabetes to efficiently respond to ischemic stimuli. This is phenomena is called “diabetic mobilopathy” [ ]. In this sense, experimental studies found important structural alterations in the bone marrow of T1D mice, with fatty degeneration and depletion of the hematopoietic component. Similar to clinical findings, endothelial progenitor cell depletion was also present, and it was associated with increased oxidative stress, DNA damage, and activation of apoptosis [ ]. On the other hand, Fadini and colleagues demonstrated that levels of circulating bone marrow–derived proangiogenic cells inversely correlate with complications in the coronary, peripheral, and cerebrovascular regions [ , ].
1.5
The heart
The coronary microvasculature performs a dynamic role to meet the oxygen and nutrient demands of the myocardium. About 90% of the myocardial blood volume is exclusively located within the myocardium, while the remaining 10% is composed of arteries and veins on the epicardial surface [ ].
Diabetic cardiomyopathy (DCM) is a heart condition defined as ventricular dysfunction in the absence of coronary atherosclerosis and hypertension [ ]. Numerous morphological alterations of microvasculature, such as periarterial fibrosis, arteriolar thickening, focal constrictions, microvascular tortuosity, capillary BM thickening, capillary microaneurisms, and decreased capillary density, are seen in diabetic myocardium. Diabetes also causes functional deficits in the coronary microvasculature, like reduced blood flow and endothelium-dependent coronary vasodilation. Both structural and functional alterations along with an altered myocardial metabolism (e.g., hyperglycemia, and lipids toxicity), inflammation imbalances, myocardial fibrosis and peripheral neuropathy, represent the pathogenesis of DCM [ , ].
A connection has been described between microangiopathy and neuropathy that may contribute to the pathogenesis and progression of coronary artery disease. This includes impairment of skin microvascular reactivity, measured as a proxy of microvascular dysfunction, which is more pronounced in T2D patients with myocardial ischemia than in patients without diabetes or coronary artery disease [ ]. Also, nephropathy has been linked to myocardial microvascular dysfunction. A study using cardiac positron emission tomography with computed tomography (PET/CT), which allows measurements of myocardial flow reserve to estimate the microvascular function of the heart, found an impaired function in T1D and T2D patients. In T1D, a reduced myocardial flow reserve was observed in the presence of macroalbuminuria, and in T2D, this occurred even with normoalbuminuria, but it was more prevalent in the presence of albuminuria [ ].
To diagnose DCM, noninvasive tests such as electrocardiography (ventricular overload), echocardiography (myocardium structural and functional abnormalities), and natriuretic peptides plasma levels (diagnosis of heart failure) can be helpful [ ].
1.6
The lung
There is evidence that the lung is another target organ of diabetes. Several abnormalities of respiratory function have been described in patients with T1D and T2D [ ]. Postmortem studies have reported microvascular abnormalities such as thickened alveolar, epithelial, and pulmonary capillary basal laminas that are significantly thicker in diabetics than in age-matched controls. These alterations are frequently linked to histological abnormalities in the lung parenchyma like nodular fibrosis [ ]. Moreover, these microvascular injuries also result in a reduced pulmonary diffusing capacity for carbon monoxide (DLCO), reduced lung volumes, and reduced pulmonary elastic recoil [ ]. Microangiopathy alterations along with chronic low-grade tissue inflammation and/or accumulation of advanced glycation could explain the lung restriction in diabetes [ ].
Pulmonary microvascular disease in DM has been assessed through echocardiography quantifying pulmonary transit of agitated contrast bubbles (PTAC). A reduced PTAC during exercise has been found in diabetes patients, especially if microvascular complications were presented, compared with those without complications and control subjects. This suggests that PTAC may be a meaningful indicator of pulmonary microvascular disease [ ].
2
Conclusions
Diabetes is a multisystem disease that affects multiple tissues and organs. Besides classical target organs of microvascular disease, in recent years, other unforeseen target organs of diabetes-induced microvascular damage have been reported such as the arterial wall, the brain, the heart, the bone, lungs, skeletal muscle, and the skin. Subjects with diabetes and microvascular disease in a specific organ or tissue have a higher risk of presenting microvascular involvement in other organ(s) or tissues. These data suggest a common microvascular impairment occurring in multiple microvascular beds. Finally, there is clear evidence of a clinical relationship between micro- and macrovascular complications, which has led to the hypothesis of a possible pathogenic link between them.
References
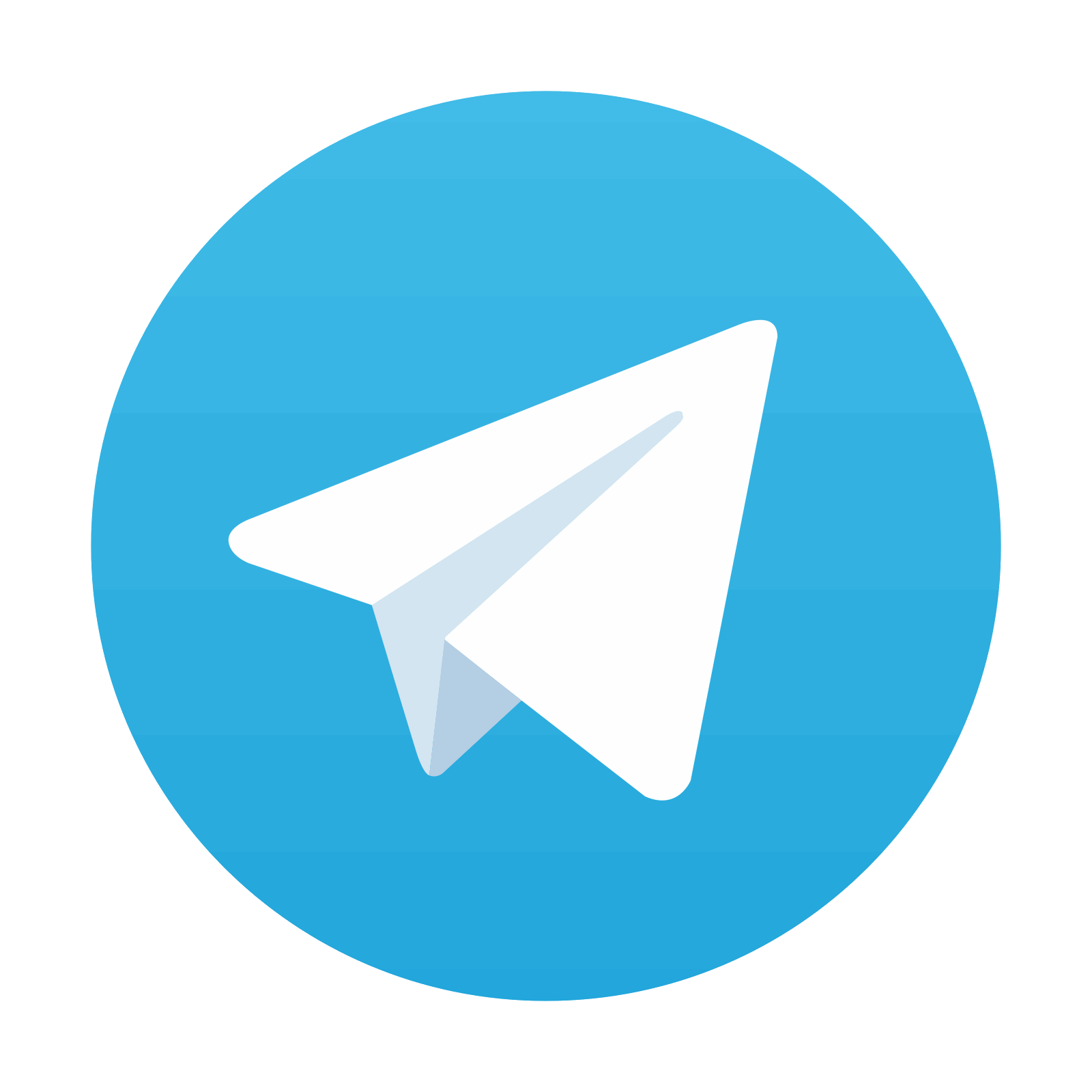
Stay updated, free articles. Join our Telegram channel

Full access? Get Clinical Tree
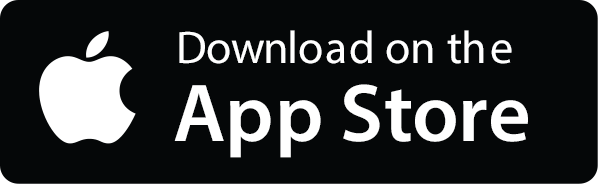
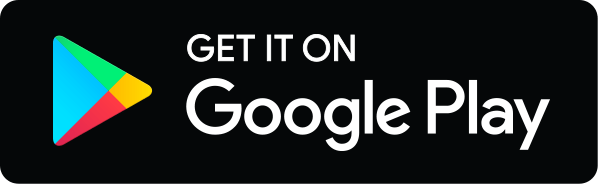
