At the core of surgical pathology is a surgeon’s obligation to their patient. In studying the tumor, a surgeon can better care for their patient. Historically, upon removing the tumor, a surgeon would walk with the specimen from the operating room to an adjacent laboratory. There he or she would dissect relevant sections for examination. This exercise paired with the growing academic influence of established schools of anatomy provided the framework for modern surgical pathology.
Johannes Muller’s (1801–1858) careful experimental methods at autopsy laid the groundwork for the young Rudolph Virchow (1821–1902) to form his cell theory “omniscellula ecellua” or “all cells come from cells.” The concept rebuilt the understanding of human disease based on the dynamics of cell interactions. Inflammation and tumor growth were considered in the intercommunication of cellular populations. Virchow’s remarkable teaching methods influenced capable followers like Francis Delafield (1841–1915) and Theophil Mitchell Prudden (1849–1924) to publish their Handbook of Pathological Anatomy and Histology in 1885. They too went on to influence other devotees of the cellular vision. James Ewing (1866–1943) who studied under Delafield and Prudden and William Welch (1850–1934) built on prior intellectual works and observations. They forged a lifework that became American surgical pathology.1
Technical advances in the field continued to sustain and grow this burgeoning discipline. While invented by Zacharias Janssen (1585–1632) and optimized by the lens work of Antoine Leeuwenhoek (1632–1723), it wasn’t until 1673 that the compound microscope made its way into the laboratory. The mid- to late 1800s saw substantial advances in optics to demonstrate its deciding value within the laboratory. Even engineering of the 3″ × 1″ glass slide and coverslip were not trivial achievements. Like any innovation, the microscope had to demonstrate that it was better than benchmark standards at that time. As with many innovations, dissenting opinions existed regarding the microscope’s usefulness. Alfred-Armand-Louis-Marie Velpeau (1795–1867), an anatomist at the University of Paris, commented, “the invention of the microscope is not at all necessary to decide whether such a tumor is of cancerous nature.”2
Another important development in the field was the use of differential staining. Up until 1850, tissue was examined unstained; as early as 1858 pathologists were experimenting with tissue staining. Probably the most noteworthy was a stain from the logwood tree (Haematoxylum campechianum) found in northern Central America, southern Mexico, and Haiti. This pigment was coupled with a mordant that fixed the color to the test tissue and perfected the staining process. Ten years later, pairing this methodology with developing synthetic dyes such as eosin provided a synergistic combination that became and remains one of the most heavily utilized staining method in the surgical pathology laboratory—Hematoxylin and Eosin or the H&E.1
In all likelihood, the most historical and iconic technical advance in the surgical pathology field was the development of the frozen section. This test was conducted within the surgery suite for immediate impact on operative decision-making. Early systems utilized freezing and salt brine solutions before briefly fixing with formalin. One of the most influential supporters of this method began as one of its most staunch critics. Joseph Colt Bloodgood (1867–1935), a prominent surgeon at Johns Hopkins Hospital, became an ardent proponent of the technique and eventually published an editorial in surgery, gynecology, and obstetrics that all small hospitals needed a pathologist with frozen section capabilities within the operative suite.1 The development of the frozen section fostered an emerging and collaborative relationship between the surgeon and the pathologist. While indeed, the first surgical pathologists were surgeons, surgical pathology eventually became a recognized subspecialty of Pathology. The American Board of Pathology was founded in 1936, the year the first board examination in Pathology occurred.
The pathology workflow begins with accessioning. Specimens are assigned an accession number based on their location where the procedure was performed (e.g., inpatient, outpatient, or specialized testing). Specimen accession is an initial step in the analytic pathology process. It ensures documentation of correct patient identification and demographics, insurance information and triage to appropriate testing. Scientific sophistication and analysis is irrelevant if that result is assigned to the incorrect patient. Approximately 55 patient identification errors are made per million specimens tested.3 This error rate costs approximately $721 per incident or $280,000 worth of unnecessary procedures and corrections. The Joint Commission views accessioning errors as a component of overall patient safety and has made improving patient safety as one of its core principles. In accordance with that vision, the commission has produced guidelines for proper documentation in their 2001 revised standards.4 Their guidelines require at least two patient identifiers in accessioned laboratory specimens. These can include a patient’s first name and last name (together), the patient’s date of birth, medical record number, encounter number, or unique identifier.5 Significant reduction in preanalytic errors can be achieved by implementing policies and procedures similar to those used for phlebotomy or transfusing blood. Careful verification of the patient’s identity should be done at the time of the biopsy or procedure. The requisition form and label should be secured and verified on the specimen container against the wrist band of the patient. If the patient is awake, the pathologist should ask to recite their name and date of birth . Specimen containers should never be left unlabeled or be labeled later. This leads to mismatched and unlabeled specimens.
In a study performed by the College of American Pathologists (CAP) analyzing approximately 1,000,000 accessions from 417 participating institutions, there was an accessioning error rate of 6.0% with a median deficiency rate of 3.4%. In those accessioning errors, 77% were due to incorrect or missing information, 9.6% from identification deficiencies, and 3.6% from specimen mishandling. Missing information most often included “No clinical history” (40.4%), “No date of procedure” (14.1%), followed by “No tissue source indicated” (9.4%). Specimen identification deficiencies were attributed to “Illegible patient name or unique identifier” (2.6%), “No label on container”(1.8%), and “No requisition slip”(1.7%). Interestingly, as it pertains to the discussion later in this chapter, the most common specimen mishandling deficiency was “Inadequate fixative in container” (2.6%).6
From accessioning, specimens are sent to the “Gross Room” for macroscopic examination. Patient identifiers are again confirmed and specimens are assessed for fixation. Basic physical characteristics of the specimen are also entered. These include weight and measurements. Notable features are documented, such as lesion size, shape, interface of tumor to surrounding normal tissue (circumscribed or infiltrative), color, texture (indurated, gritty), and distance to surgical margins. Any identifiable markers from the surgeon or radiologists (such as clips) are also recorded. Most of the time these markers are used to orient the surgical margins “long [suture]—lateral and short [suture]—superior,” or “suture at 12:00.” Inks are used to paint the surgical margin and indicate its appropriate orientation. For example, 12 o’clock—blue; 3 o’clock—red; 6 o’clock—green; 9 o’clock—yellow, or anterior—red, superior—green, inferior—blue, posterior—yellow, and deep—black. The use of multiple colored dissection inks help to maintain the margin orientation until tissue processing to be present on microscopic examination. The inks are designed specifically to withstand tissue processing and be visible on the glass slide.
Adequate fixation is essential for optimal morphology. In addition, fixation may also impact ancillary testing. This is best illustrated in the processing of breast lesions. In recent years the importance of cold ischemic time (the time interval when the tissue is removed from the patient to the time that the tissue is placed in formalin) has been determined to be a factor in immunohistochemical variability and ancillary testing. Using breast tissue specimens as an example, cold ischemic times of more than 1 hour and formalin fixation times of less than 6 hours or more than 72 hours all adversely influence the reliability of biomarker results for hormone receptor and Her-2 neu status. Khoury and colleagues reported that cold ischemic times greater than 1 hour affected the detectability of hormone markers. Her-2 neu gene signal detection by fluorescent in situ hybridization was particularly vulnerable.7
One of the important factors of adequate fixation is the size of the specimen and the appropriate amount of formalin it is placed in. Formalin penetrates tissue at approximately 4 mm per hour. A general guideline is 10 volumes of formalin for every 1 volume of the specimen. Invariably, specimens should be inserted in 10% buffered formalin and not formaldehyde. Ten percent buffered formalin is a 1:10 dilution of formaldehyde, which has a phosphate buffer to control the pH of the tissue sample. Without the buffer, artifacts are introduced which hinder the morphologic interpretation of the specimen. It is also prudent to note that formalin is toxic and should not come into contact with the skin or eyes. If it does, the area that comes into contact must be rinsed for 15 to 20 minutes with copious amounts of water. Formalin is considered a carcinogen and should be handled in a well-ventilated area.
Special techniques can assist with fixing large tissues: the hollow specimens can be opened and pinned to a dissection board to ensure adequate fixation of the inner and outer surfaces (e.g., bladder, colon); solid organs and masses can be sectioned prior to fixation and paper towels placed between the cuts to wick formalin deeper into the tissue. This increases the fixation surface area. Critical sections can be taken and fixed by themselves in smaller containers of fixative. This last technique has the added benefit of preserving the spatial relationship of a lesion and its surrounding landmarks. Depending on the tissue, formalin fixation can reduce the tissue by 20% to 25% of the original length. This contraction can twist and distort the tissue making dissection difficult. Pre-fixing sections on a pin board may better preserve these relationships so that more representative sections can be sampled for microscopic examination. All of these special techniques should only be performed by the pathologist, as margins will need to be inked prior to cutting the specimen, and certain relationships will be lost after opening the specimen. Specimens should never be cut open by the surgeon without the presence of the pathologist that will handle the case.
Tissue sections are taken. These representative sections often include the lesion, its relationship to the surrounding margins, and sampling of adjacent normal structures. The sections are placed into plastic cassettes with appropriate patient identifiers. Barcoding can be useful in this situation to ensure adequate tracking of the specimen throughout tissue processing. Over the day, the cassettes are placed into metal racks submersed in 10% buffered formalin, awaiting tissue processing.
The processing of tissue to microscopic slides is a fundamental activity in any successful surgical pathology laboratory. The quality of a laboratory can be defined by this one activity. Microscopic tissue sections are usually cut at 4 µm. Cutting extremely thin sections of unfixed biopsy and resection material will destroy the tissue and render the histomorphology uninterpretable. In order to achieve the appropriate thickness for light microscopy, a solid support structure must be infused in the tissue to prevent this loss of diagnostic material.
The solution for this barrier is paraffin wax . Paraffin continues to be the most popular infiltration and embedding medium. It provides an optimal support matrix to prevent tissue distortion during microtomy. Human tissue is approximately 50% to 65% water. In order to permeate this organic substance into a tissue that is primarily water, the aqueous elements of that tissue must be extracted with an organic solvent, like xylene. This is a fundamental principle of modern tissue processors. Through gradient alcohols (two baths of 95% ethanol, two baths of 100% ethanol, followed by two baths of clearing agent such as xylene), pressure, heat, and fluid circulation, tissue processing has become more efficient. In the past, motors, gears, and buckets basically dipped the tissue in gradient solutions using older Technicon, Inc. tissue processing units. The processing time was over 16 hour time period. In modern tissue processors this exchange can be achieved within an hour for biopsy-sized tissues.
On emerging from the processors, the tissue goes through a process that truly embodies the art of histotechnology—embedding. Tissues are taken out of the cassettes and cast into paraffin molds. The importance of embedding is far-reaching. The placement of that tissue determines the ease with which the tissue is cut during microtomy, the appearance of the tissue on the glass slide, and the efficiency in scanning of the slide during a pathologist’s review of the case (Fig. 6-1A).
FIGURE 6-1
Slide processing. A. Tissue is embedded in metal paraffin molds that form the paraffin block. B. The paraffin block is then cut on a microtome forming a paraffin ribbon. This paraffin ribbon is floated on a heated water bath that smoothes out wrinkles and folds. C. When the ribbon is flat, sections are picked up on a glass slide for staining.
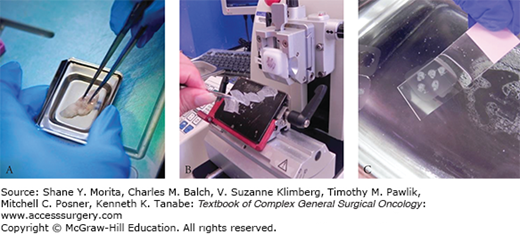
Tubal structures should always be embedded on cross-section. Epithelial surfaces should be oriented to pass the microtome blade edge last, thus minimizing compression and distortion during microtomy. Multiple segments of tissue if submitted together should be oriented side by side with the epithelial surface oriented in the same direction. These are some examples of good tissue embedding practices.
After the paraffin blocks have been cast, the tissue goes on to slide processing. Histotechnology is an essential aspect. Sllides are cut at 4 to 5 µm. The surface of the paraffin block is initially trimmed to ensure a flat surface prior to sectioning for the glass slide (Fig. 6-1B). This is called “facing the block.” This trimming is important for smaller lesions. Facing the block could cut through a small lesion abutting the block surface, or can cut through a small sample such as a cell block for a cytology specimen. A timely note to the histotechnologists cutting the tissue to limit the trim could prevent technical loss of the lesion. The paraffin ribbon is submerged onto a heated water bath that spreads the section out. This prevents wrinkles and folds and ensures a flat, even two-dimensional section. Ribbons are applied onto the glass slides for staining. Most slides are untreated glass. Other slides are coated with an adhesive material or positively charged to help with tissue adherence during the prolonged incubation times of immunohistochemistry or fluorescent in situ hybridization (Fig. 6-1C). Slides are dried and then placed in an oven at 60° to further promote adhesion to the glass slides and to melt the paraffin wax. The slides are then immersed in a xylene bath to wash out any remaining paraffin. The slides proceed through gradient alcohol solutions and finally equilibrate in an aqueous medium. In this state the slides can be stained with aqueous stains such as hematoxylin and eosin. Water is again extracted from the tissue by gradient alcohols and any excess water is cleared by xylene. The slide can now be coverslipped and preserved in an organic/hydrophobic mounting media. Slides are required to be held in the pathology lab for 10 years (42 CFR 493.1105). If the slides are not coverslipped, the tissue will fade with time and the tissue will eventually decompose. To prevent this, slides are coverslipped in an organic mounting media, which seals the slides to air and water.
The mounting media is allowed to briefly dry after coverslipping. The slides are paired with their patient requisition using appropriate patient identifiers. In some labs, barcodes are used to track this process. Once the slides and requisition are together, a scan of the barcode will indicate that the case is complete and ready for distribution to the appropriate pathologist.
In the early days of American surgical pathology fixation was a problem. Early coagulant fixatives such as chromic acid took approximately 6 weeks for the tissue to be suitable for cutting. In 1893 a German physician discovered the value of formaldehyde as an effective tissue fixative. Fixation enhanced histologic morphology by supporting the tissue during microtomy. Formaldehyde chemically interacts with the tissue and makes proteins easier to stain. Fixation preserves macromolecules for ancillary studies such as fluorescent in situ hybridization, DNA sequencing, expression analysis, and possibly proteomic studies.1 In the expanding molecular era of surgical pathology, it is of some debate whether formalin remains a useful tool in the pathology laboratory or has become a hindrance to its future growth.
Despite this, the most common fixation used in the surgical pathology laboratory remains formalin. Formalin is formaldehyde diluted 1:10 with neutral buffered water. Formalin is a noncoagulant cross-linking fixative that achieves preservation by the formation of methylene groups on several different side chains of amino acids. The side chains most reactive with these methylene groups are lysine, cysteine, histidine, arginine, tyrosine, and serine and threonine. Formalin fixation over longer periods of time also cross-links lysine and amide groups along the protein backbone. Cross-linking of adenine and thymidine nucleotides is another result of longer fixation times and can have deleterious implications in further DNA sequencing–based assays.8
There are some important key points to formalin fixation. The fixative must penetrate and infuse the submitted specimen. The general guideline is 10 volumes of fixative per 1 volume of tissue. This is important for larger specimens that require placement in larger containers for appropriate fixation. Poor fixation and histology are often the fate of large sarcomas that find themselves in a container that is too small and with too little fixative. Formalin penetrates at a rate of 4 mm of tissue per hour. A 1-cm sphere of tissue would take approximately 25 hours for adequate fixation.8 The gross exam can have a substantial impact on the fixation of large specimens. In the initial dissection, larger specimens can be sectioned into smaller segments that increase the surface area and enhance overall fixative penetration. Also, a tissue that is mostly fat will not be well-fixed due to the hydrophobic nature of fat. Breast specimens have an abundant amount of fat and are susceptible to this fixation problem.
Hollow or large tubal specimens are also suceptible to poor fixation techniques. These cavitary specimens are best sectioned and pinned open, exposing the epithelial surface for adequate fixation. It’s unlikely in its intact structure to allow a fixative to penetrate the outer layers of soft tissue and into the lumen of the specimen to preserve the epithelial surface. This will ultimately affect the histomorphology of an epithelial tumor located on the inner lining and its associated ancillary molecular diagnostics.
Extraneous proteins covering the specimen can also affect fixation. Fixed hemoglobin forms a black pigment that retards fixation. This is often the case for hemorrhagic specimens coated in blood. Layers of adherent blood prevent the penetration of fixative to the underlying specimen.8 In these situations, the hemorrhagic coating needs to be rinsed off with water before sending it to the container for fixation. Furthermore, specimens with a lot of blood will also diminish the buffering capacity of the fixative and introduce formalin-heme pigment that obscures histomorphology.
Fixation without a doubt preserves tissue for exquisite morphologic assessment. However, as we progress, precision medicine demands more from the paraffin block than just morphology. It requires the preservation of macromolecules to ensure accurate ancillary testing. Test manufacturers have achieved much in working with this inescapable fixative. RNA microarray manufacturers have reported near 90% of mRNA expression content compared with fresh frozen tissue.9 Still with the mounting importance of ancillary testing in the pathologic process, the question remains “is there a substitute that can achieve all of these requirements and still provide formalin-type tissue morphology?”
The holy grail of formalin substitutes and alternative tissue fixatives are well placed. Ideally, it would be a substance that would preserve morphology yet protect macromolecules for efficient extraction and ancillary testing. Specifically, it would maintain the integrity of DNA sequence by preventing nucleic acid cross-linking, preserve the expression patterns of the mRNA milieu, and protect the breadth of proteomic content. As a result, a number of formalin substitutes have found their way into the marketplace that report morphologic preservation and protection of macromolecules: Z7 (Montreal, Quebec, Canada), RCL2 (ElanCourtandPlainsir, France), PAX gene™ (Quigen-PreAnalytic, Venlow Netherlands), Allprotect® (Quiagen, Venlo, Netherlands), and the HOPE fixative (Polyscience, Inc., Warrington, PA). All candidates show varying strengths and weaknesses based on nucleic acid extraction, DNA and RNA quality, in situ hybridization, and immunohistochemistry compared to neutral buffered formalin.10
Formalin provides an economical solution for preservation of morphology based on generations of pathologist’s expectations of tissue morphology. It sterilizes tissue of viruses and bacteria, although it has no effect on neutralizing prions. It also preserves carbohydrate antigens. As the focus of fixation looks beyond morphology to ancillary genomic testing, formalin cross-links proteins in situ and protects them for further proteomic analysis. This loss of protein content can be seen when compared to non-cross-linking fixatives such as alcohol- or methanol-based solutions.
There is a high burden of proof placed on alternative fixatives. Virtually all of the evidence-based literature on outcomes of immunohistochemical and molecular studies have been performed on formalin-fixed paraffin embedded tissues. To change to a different, nonstandard fixative would require extensive validation studies to ensure reliability of results in immunohistochemistry and molecular techniques. In addition, formalin’s use in the surgical pathology workflow is so institutionalized that unless the benefits of an alternative fixative are decisively clear, switching may be too disruptive to feasibly engender adoption by most laboratories.
The core activity of the surgical pathology laboratory is the macroscopic inspection and microscopic examination of tissue. Over the years, additional ancillary techniques and assays have been developed to support and optimize this primary function. The following sections highlight key methodologies utilized in the modern-day surgical pathology report.
Some of the founding advances of surgical pathology were histochemical stains. These are differential stains that accentuate and characterize unique features of the histomorphologic landscape. Currently more complex analyses are performed on tissue sections; however, special stains continue to be useful adjuncts in the histologic diagnosis (Table 6-1).11 For example, acid-fast stains provide a rapid evaluation of Mycobacterium sp.
Selected Histochemical Stains Used in Surgical Pathology
Material | Stain | Result | Comment |
---|---|---|---|
Amyloid | Congo Red | Amyloid: Red Amyloid: Green birefringence (polarization microscopy) | Provides additional evidence of a plasmacytic neoplasm |
Bacteria | Brown-Brenn | Gram-positive: Blue Gram-positive: Red | Organism identification |
Fungi | Periodic acid-Schiff (PAS) GMS | Cell wall: Red Cell wall: Black | Organism identification |
Fat | Oil Red O (Frozen section only) | Fat: Red | Assists in establishing tumor differentiation for sebaceous neoplasms |
Glycogen | Periodic acid-Schiff (PAS) with diastase digestion | Mucin: Red; absent staining after digestion | Used to show fatty changes of the liver |
Cytoplasmic microvesicles | Hale’s colloidal iron | Microvesicles: Blue | Renal oncocytoma |
Hemosiderin | Prussian blue Perl’s potassium ferrocyanide | Iron: Blue Iron: Blue | Provides additional evidence of liver cirrhosis |
Mucin | Mucicarmine Alcian blue Periodic acid-Schiff (PAS) with diastase digestion | Mucin: Pink to red Mucin: Blue Mucin: Red; no change after digestion | Used to confirm adenocarcinoma versus squamous cell carcinoma |
Melanin | Fontana-Masson | Melanin: Black | Provides additional evidence of melanocytic origin in a necrotic tumor |
Reticulin | Wilder or Gridley reticulin stain | Reticulin: Black | Provides additional evidence of expanded liver plates in hepatocellular carcinoma |
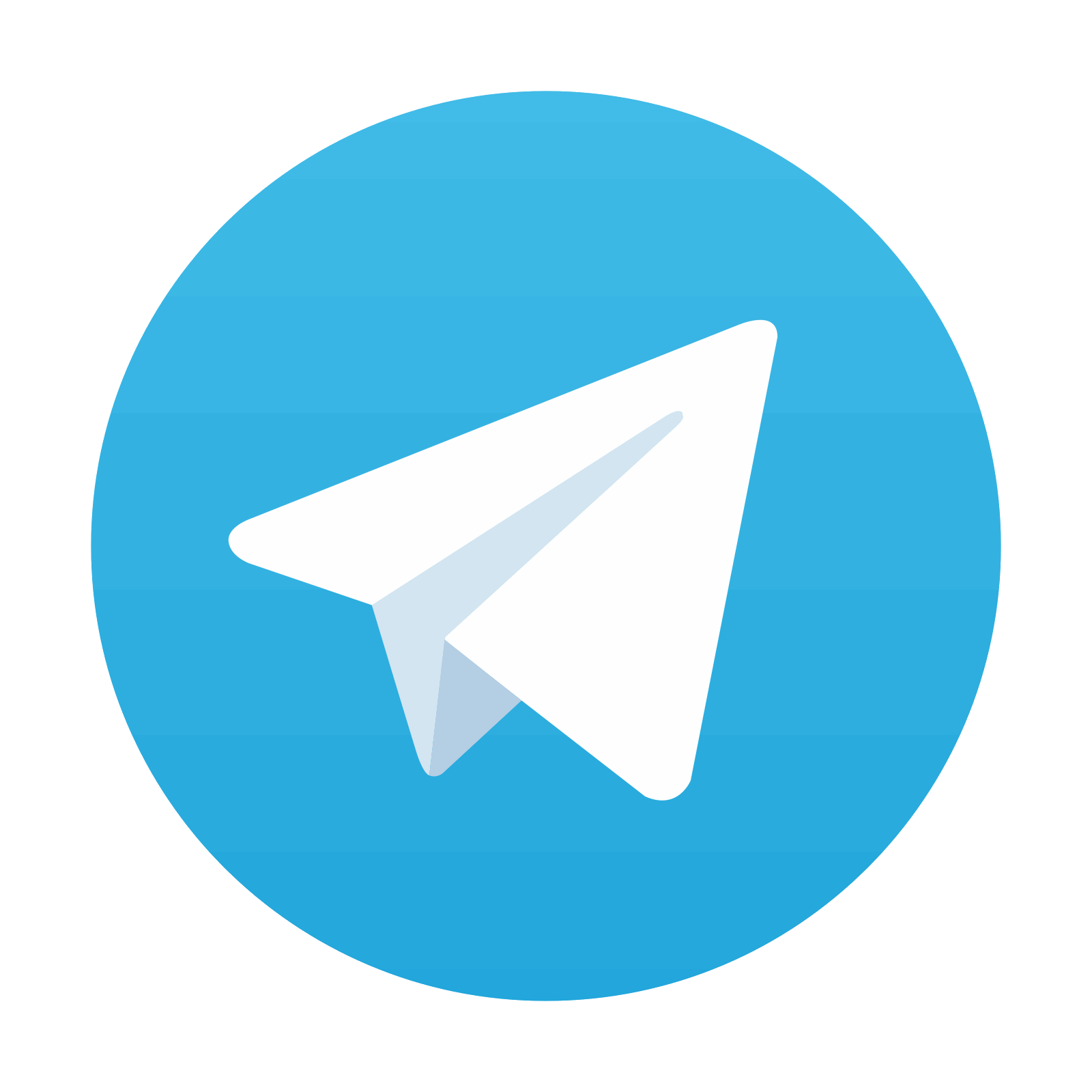
Stay updated, free articles. Join our Telegram channel

Full access? Get Clinical Tree
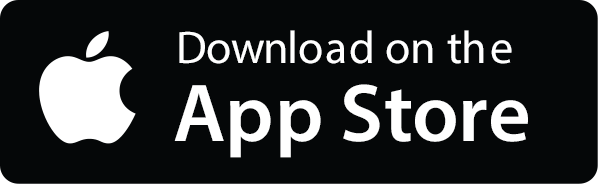
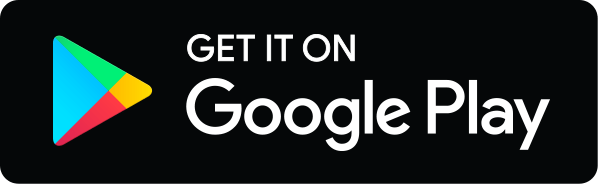
