Fig. 4.1
(40× and 200×) Specimens with predominantly tumor and minimal non tumor diluting cells. This is still not 100 % tumor as there are still non tumor nucleated cells including stromal cells, endothelial cells, and inflammatory cells
Mucin/mucus: Mucin or mucinous product can suppress PCR [1, 28]. Hence, it is important to minimize the quantity of mucin [29] present in the specimen/sample (Fig. 4.2) sent for molecular testing. Nonetheless, mucinous tumors/carcinomas can be and have been successfully evaluated by molecular PCR techniques [30–32]. Some sort of endogenous internal control might help determine the presence of inhibitor(s), because both the target signals and endogenous internal control signals would be suppressed [33, 34].
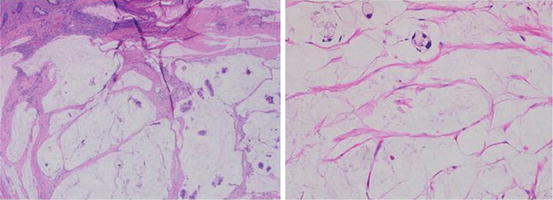
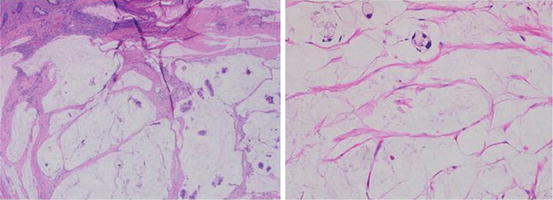
Fig. 4.2
Minimal tumor, mostly diluted by mucin. For cases like this laser microdissection may be appropriate as mucin may inhibit PCR
Blood/heme: PCR may also be inhibited by hemolysis or the presence of excess heme [1, 35, 36]. Heme can act as a competitive inhibitor of the target DNA and a non-competitive inhibitor with the nucleotides through direct action against the DNA polymerase [37, 38]. This may be more relevant to PCR performed on blood specimens, not solid tumors where the specimen has been processed in fixatives. Nonetheless, areas with extensive hemorrhage should probably be avoided or minimized [35], although this may not adversely affect testing on FFPE specimens, as nucleic acid extraction process using this type of specimen may eliminate this inhibitor [39].
Necrosis: It is important for pathologists to avoid or minimize areas of necrosis and superficial ulceration (if necrotic) in the specimen (Fig. 4.3) as extensive necrosis might complicate analysis [28, 29, 40]. Necrosis degrades DNA and RNA leading to reduction in target signal and might serve as some sort of inhibitor of PCR. In addition, cells surrounding the necrotic area may have unique alterations related to the exposure to such necrosis, as change in the transcriptome of these cells has been reported [41]. Comparison of target signal to internal amplification control (if endogenous DNA or RNA) would show suppression of both signals suggesting some sort of inhibitor.
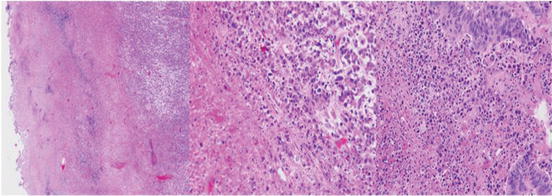
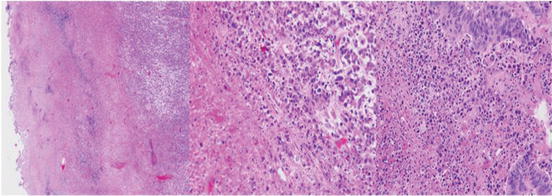
Fig. 4.3
(40× and 200×). Areas of abundant necrosis or surface ulceration should be excluded as necrosis may inhibit PCR. Manual macrodissection or laser microdissection may be necessary to minimize necrosis and enrich for tumor
Desmoplasia: It is also important to avoid areas with prominent desmoplastic reaction (Fig. 4.4) as the increased non-tumor nucleated cells may dilute the tumor, reduce cellularity, and complicate percent estimation of tumor cells [29]. This is also important in specimens procured after neo-adjuvant treatment. It important to remember that all nucleated cells should be included in the denominator.
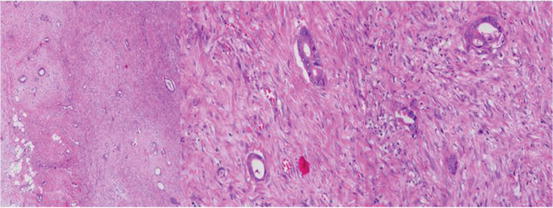
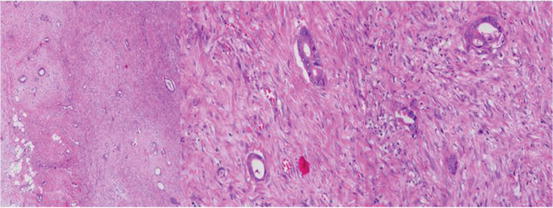
Fig. 4.4
(40× and 200×): Image of tumor showing extensive dilution by desmoplasia. Careful review shows only scant tumor cells in a background of abundant desmoplastic stroma cells and endothelium of blood vessels. All the nucleated cells have to be factored into the denominator for calculation of percentage of tumor. Tumor enrichment like laser microdissection may be useful in such cases
Melanin: Areas with abundant melanin should also be avoided as melanin has been reported to be a potent inhibitor of PCR [29, 37, 42–44] and may interact with the thermostable DNA polymerase [42, 43]. The effects of melanin on PCR can be mitigated by methodology that removes the melanin from the specimen [43, 45]. It is probably prudent to avoid areas with heavy melanin pigments.
Skeletal muscle: Muscle tissue contains PCR inhibitors, and myogloblin, a component of skeletal muscle, acts as a potent Taq polymerase inhibitor; however the effect can be minimized or eliminated by the use of Thermus thermophilus (Tth) polymerase, especially in small samples [46]. This is generally not a big issue in the molecular testing of solid tumors.
Inflammatory cells and stromal cells: Minimizing the amount of inflammatory cells (neutrophilic or lymphoplasmacytic) and stromal cells [28, 29] is important as these dilute the tumor (Fig. 4.5). The inflammatory cells can be intratumoral (or intraepithelial) or in the stroma. The inflammatory cells in most cases are smaller than the tumor cells and may be easily overlooked with their proportion underrepresented in the denominator for percentage tumor estimation.
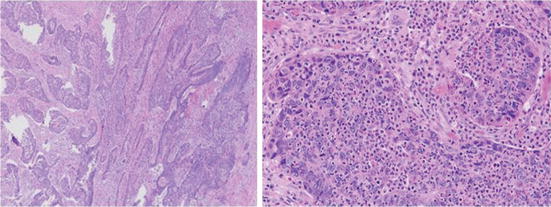
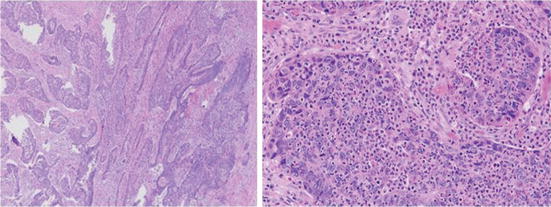
Fig. 4.5
(40× and 200×): Image of tumor showing extensive dilution by inflammatory cells. Careful review shows the inflammatory cells are in the lamina propria, intraluminal, and intraepithelial. In addition there are stromal cells and endothelium of blood vessels. All the nucleated cells have to be factored into the denominator for calculation of percentage of tumor
From the foregoing the importance of paying careful attention to the determination of specimen adequacy for molecular testing should critical. In addition, standardization of the pre-analytic processes is equally important as these variables may enhance the yield and quality of nucleic acid used for molecular testing [11, 14, 16, 24, 35, 39, 47–49].
Decalcification and Molecular Testing
Bone is often the site of metastasis of a wide range of solid tumors. Bony spicules are rich in calcium which may inhibit nucleic acid assays. Furthermore, bone specimens (biopsy or resection), often requires decalcification for routine histologic evaluation. Most of the decalcification agents using strong inorganic acid (like hydrochloric or nitric acid) allow for rapid decalcification, but often cause significant nucleic acid degradation [50–52] often precluding further molecular testing. It has been suggested that decalcification agents containing 14 % EDTA or formic acid either as sole agents or in combination are preferred for molecular testing [50]. While EDTA containing decalcification agent is preferred it is slow acting and decalcification may take a considerable longer time [50, 52]. Some have advocated using ultrasonic decalcification, though it is not widely adopted [51].
Nucleic Acid Extraction
The FFPE specimens (e.g., the paraffin block or unstained FFPE slides) sent for molecular testing are deparaffinized and treated/digested with proteinase K. Pre-digestion heat treatment may be used as validated in the laboratory [53–56]. Digestion with proteinase K or similar agents is important because it removes or digests unwanted protein bound to DNA allowing nucleic acid extraction and subsequent easier amplification [24, 47]. Since it has been shown that DNA cross-linking caused by formalin is heat reversible [57, 58], increased exposure to high temperatures (90–98 °C) steps have been added to the extraction protocols from FFPE specimens to counteract such cross-linking. In addition, it is worth noting that DNA extracted from FFPE specimens following such protocols is usually of lower molecular weight (i.e., fragmented) than DNA isolated from fresh or frozen samples. The degree of fragmentation is often related to the type and age of the sample as well as the fixation conditions. After digestion, well developed and documented methods of extracting nucleic acid from FFPE are then employed to obtain DNA [or RNA as needed] [8, 11, 24, 39, 47, 53–56, 59, 60]. Following nucleic acid extraction, a validated methodology is then used to analyze the specimen. Some of these methodologies are discussed in Chap. 5 of this text.
Reporting
Immunohistochemistry
It is important to point out that since proteins are translated from mRNAs which are in turn transcribed from DNA, abnormalities detected at the protein level by immunohistochemistry may be a reflection of abnormalities at the mRNA and DNA [61]. This becomes relevant when immunohistochemistry is used as a screening test for germline mutation as in the cases of mismatch repair for colorectal cancer. Immunohistochemistry detects abnormalities at the protein level, specifically the epitope at which the immunohistochemistry antibody is directed. However, in some cases immunohistochemistry may be used to screen for abnormalities at the genetic level. For example, immunohistochemistry is generally used for breast carcinoma ERBB2 (aka HER2) predictive markers. Immunohistochemistry is also used for as a screening test for mismatch repair (MMR) abnormalities in colorectal carcinoma (MLH1, MSH2, MSH6, and PMS2). It should be remembered, however, that post translational modifications, alteration of the tertiary and quaternary structure (e.g., by fixation) may modify the antigen conformation and mask epitope(s) such that it is not recognized by the IHC antibody, even with antigen retrieval leading to a negative result, when in fact the mRNA is normal. The converse also applies, with mutations of the gene but normal protein expression on immunohistochemistry [62–65]. The proteins from abnormal mRNA may have lost their functions but retain their antigenicity. While awareness of these possible limitations of immunohistochemistry is important for pathologists, immunohistochemistry remains an important standard practice in pathology.
Summary
It is essential for pathologists to understand and embrace the critical role they play in molecular oncology testing or risk abdicating this role to others. Effectiveness in carrying out this role requires basic knowledge of how normal pathologic processes might affect nucleic acid testing or extraction. This chapter has highlighted some factors that may affect nucleic acid extraction and testing. Careful attention to the adequacy of the specimens sent for molecular testing is required for optimal testing and reliable results.
References
1.
CLSI. Establishing molecular testing in clinical laboratory environments: approved guideline. CLSI document MM19-A. Wayne, PA: Clinical and Laboratory Standards Institute; 2011.
2.
3.
4.
5.
Kashofer K, Viertler C, Pichler M, Zatloukal K. Quality control of RNA preservation and extraction from paraffin-embedded tissue: implications for RT-PCR and microarray analysis. PLoS One. 2013;8(7):e70714.PubMedCentralCrossRefPubMed
6.
7.
Evers DL, Fowler CB, Cunningham BR, Mason JT, O’Leary TJ. The effect of formaldehyde fixation on RNA: optimization of formaldehyde adduct removal. J Mol Diagn. 2011;13(3):282–8.PubMedCentralCrossRefPubMed
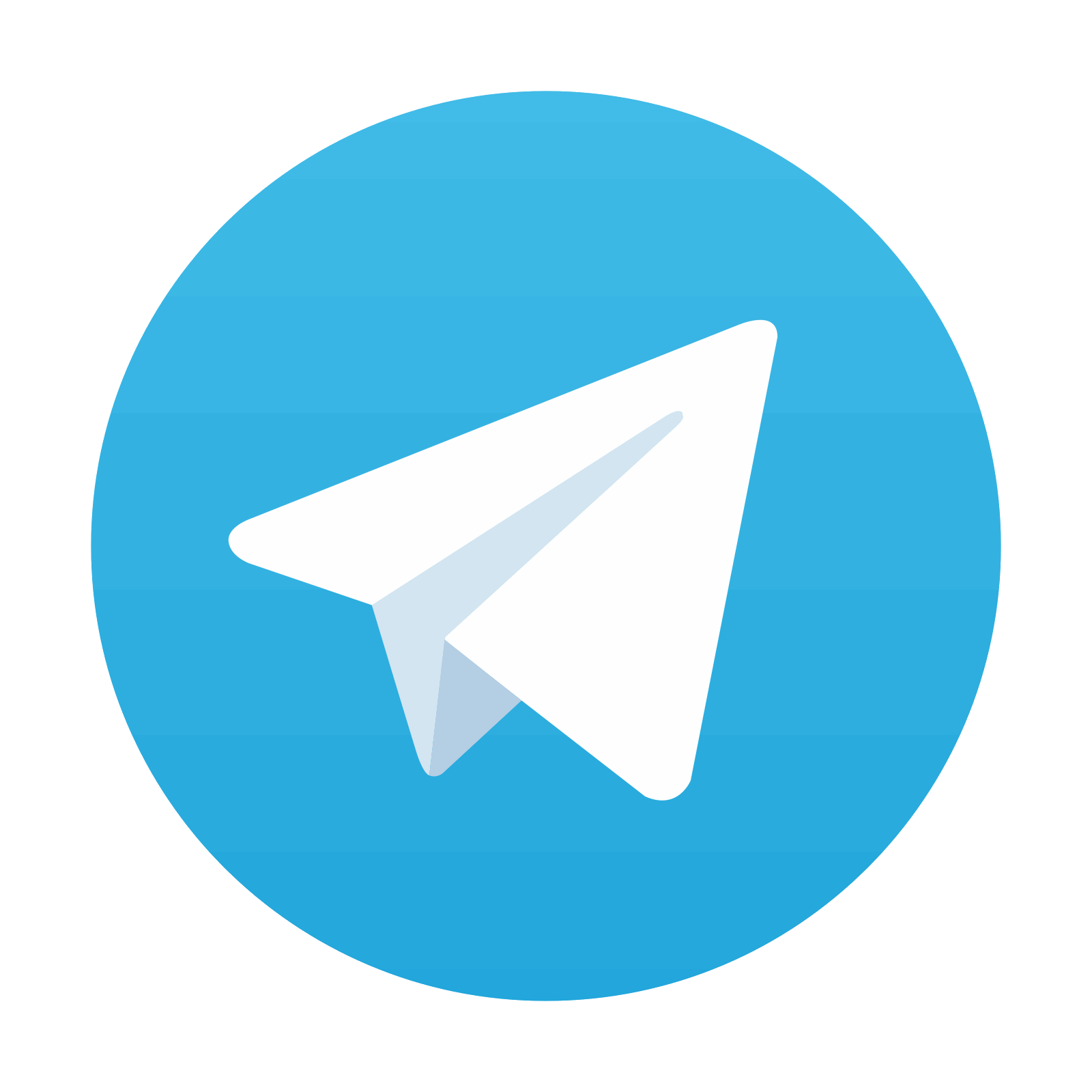
Stay updated, free articles. Join our Telegram channel

Full access? Get Clinical Tree
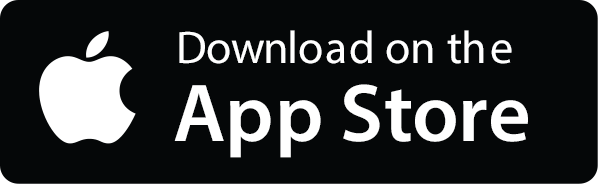
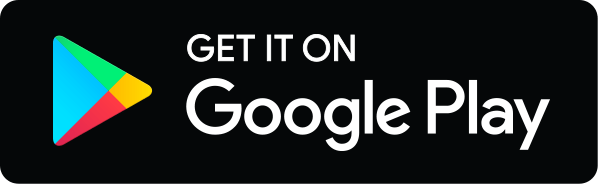