Biomarker
Description
Method
Reference
CA 15-3
Tumor antigen
Serum ELISA
Woolas et al. [41]
CA 19-9
Tumor antigen
Serum ELISA
Woolas et al. [41]
TAG-72
Tumor antigen
Serum ELISA
Woolas et al. [41].
CA 54/61
Tumor antigen
IHC
Suzuki et al. [42].
OVX1
Tumor antigen
Serum RIA
Woolas et al. [17]
M-CSF
Growth factor
Serum RIA
Woolas et al. [17].
CEA
Tumor antigen
Luminex® (serum)
Yurkovetsky et al. [43].
CASA
Tumor antigen
Serum ELISA
Sehouli et al. [44].
LASA
Tumor antigen
Serum ELISA
Crump et al. [45]
UGF
Tumor antigen
Serum ELISA
Crump et al. [45]
HER2/neu
Growth factor receptor
Serum ELISA
Crump et al. [45]
EGFR
Growth factor receptor
MS/serum ELISA
Baron et al. [46].
sICAM1
Adhesion molecule
Serum ELISA
Callet et al. [47].
VEGF
Angiogenesis factor
Serum ELISA
Oehler et al. [48].
Lysophosphatidic acid
Mitogenic factor
Plasma ELISA
Xu et al. [49].
Kallikreins 4–8,11,15
Proteases
Various methods
HE4
Tumor antigen
Microarray
Schummer et al. [57].
Prostasin
Protease
Microarray
Mok et al. [58].
Osteopontin
Bone factor
Microarray
Kim et al. [59].
Phases of Cancer Biomarker Development
Biomarker development efforts to date clearly indicate that no individual biomarker, including CA-125, can provide sufficient SN at high SP for the early detection of ovarian cancer. Therefore, the bulk of research efforts are now focused on the development of robust multimarker algorithms incorporating additional informative biomarkers which complement CA-125. According to the requirements of the Early Detection Research Network/NIH, biomarker development should include five phases: preclinical exploratory Phase 1, clinical development and validation in Phase 2, validation in a retrospective longitudinal study in Phase 3, prospective screening in Phase 4, and randomized clinical trials in Phase 5 [61].
Methodological Approaches to Protein Biomarkers Development
Noninvasive biomarker testing is essential for practical general population monitoring. Therefore, biomarkers that can be detected in bodily fluids such as blood, urine, saliva, or cervical mucus could be considered. The candidate marker must be both stable in these body fluids and present in quantities that can quantitatively differentiate individuals with ovarian cancer from individuals without disease. In the arena of Phase 1 protein biomarker development, a number of conceptual and methodological approaches have been utilized for biomarker discovery in bodily fluids. Early efforts, including those responsible for the identification of CA-125, described the analysis of murine antibodies capable of reacting with tumor cells from ovarian cancer patients [62–64]. Later, biomarker discovery was achieved primarily by means of high-throughput screening methodologies, such as genomic, transcriptional, and proteomic profiling [2, 65–75]. Proteomic profiling provides direct information about proteins differentially expressed in cancer patients and control individuals. Such profiling has been performed by mass spectrometry (reviewed in [66]) and, lately, through the use of antibody arrays, such as multiplex bead-based arrays or reverse antigen arrays [43, 76]. Both approaches have limitations. To date, mass spectrometry (MS)-based methods have demonstrated limited analytical sensitivity, and consequently most of the biomarkers discovered by proteomics represent high-abundance, predominantly acute-phase proteins [4]. Recently developed methods aimed at the depletion of abundant serum proteins may overcome this limitation and lead to the enhanced discovery of lower-abundance biomarkers [77, 78]. Immunoassays permit the analysis of proteins present in bodily fluids at much lower concentrations, as low as fg/mL, but this approach is limited by the availability of suitable immunoassays. However, the menu of candidate biomarker immunoassays continues to expand as the popularity of multiplex bead-based platforms grows. For instance, three large screening exercises aimed at the identification of ovarian cancer biomarkers using multiplex bead-based immunoassays evaluated over 300 candidate biomarkers in serum obtained from large groups of ovarian cancer patients and controls [43, 76, 79].
A comprehensive review of the relevant literature identified over 160 proteins reported to be differentially expressed in early ovarian cancer in comparison to healthy controls (Table 3.2). The ongoing analysis of these proteins and the biological and pathological pathways they regulate could provide powerful tools for determining the mechanisms of ovarian cancer initiation and progression that could eventually lead to individualized molecular medicine. However, as mentioned above, further validation steps are necessary to determine the diagnostic value of these proteins, and relatively few have been evaluated in studies which meet the criteria for Phase 2 and/or 3 biomarker development [43, 76, 125–129].
Table 3.2
Ovarian cancer-associated proteins
Biological function | Biomarkers | Role in cancer |
---|---|---|
Cytokines/chemokines | IL-1,IL- 6, IL-7, IL-8, IL-10, IL-11, IL-12, IL-16, IL-18, IL-21, IL-23, IL-28A, IL-33, LIF, TNFR1-2, HVEM (TNFRSF14), IL-1Rα,b, IL-2R, M-CSF, MIP-1α, TNF-α, CD40, RANTES, CD40L, MIF, IFN-β, MCP-4 (CCL13), MIG (CXCL9), MIP-1delta (CCL15), MIP-3α (CCL20), MIP-4 (CCL18), MPIF-1, SDF-1a+b (CXCL12), CD137/4-1BB, lymphotactin (XCL1), eotaxin-1 (CCL11), eotaxin-2 (CCL24), 6Ckine (CCL21), BLC (CXCL13), CTACK (CCL27), BCA-1 (CXCL13), HCC4 (CCL16), CTAP-3 (CXCL7) | Tumor growth, angiogenesis, immune functions, inflammation, invasion |
Growth/angiogenic factors | IGF-I, VEGF, VEGFR3, EGFR, ErbB2, CTGF, PDGF AA, BB, PDGFRb, bFGF, TGFbRIII, betacellulin, IGFBP-1–4, 6, BDNF, PEDF, angiopoietin-2, renin, lysophosphatidic acid (LPA), b2-microglobulin, sialyl TN, ACE | Tumor growth, angiogenesis |
Adhesion molecules/proteases/related molecules | CA-125, CA 19-9, CEA, CA 15-3, CA-50, CA 72-4, OVX1, mesothelin, sialyl TN [80], MMP-2,3,7,9, VAP-1, TIMP1-2, tenascin C, VCAM-1, osteopontin, KIM-1, NCAM, tetranectin, nidogen-2, cathepsin L, prostasin, matriptase, kallikreins 2,6,10, cystatin C, claudin, spondin-2, SLPI | Invasion/metastases |
Hormones/related molecules | bHCG, urinary gonadotropin peptide, inhibin, leptin, adiponectin, GH, TSH, ACTH, PRL, FSH, LH, cortisol, TTR, osteocalcin, insulin, ghrelin, GIP, GLP-1, amylin, glucagon, peptide YY (PYY), follistatin, hepcidin | Tumor growth, transformation |
Acute phase reactants/coagulation factors | CRP, ApoA1, CIII, H, transthyretin (TTR), SAA, SAP, complement C3,4, complement factor H, albumin, ceruloplasmin, haptoglobin, beta-hemoglobin, transferrin, ferritin, fibrinogen, thrombin, von Willebrand factor, myoglobin, immunosuppressive acidic protein, lipid-associated sialic acid (LASA), S100A12 (EN-RAGE), fetuin A, clusterin, alpha1-antitrypsin, a2-macroglobulin, serpin1 (human plasminogen activator inhibitor-1, tPAI-1), Cox-1, Hsp27, Hsp60, Hsp80, Hsp90, lectin-type oxidized LDL receptor 1 (LOX-1), CD14, lipocalin 2, ITIH4 | Inflammation, tumor growth, angiogenesis |
Apoptosis factors | sFasL, Cyfra 21-1, TPA, perforin, DcR3 | Tumor growth, immune functions |
Energy homeostasis | AGRP, creatine kinase-MB | Tumor growth |
Proteins with undefined or other functions | HE4, human milk fat globule (HMFG 1–2), NT-Pro-BNP, neuron-specific enolase (NSE), CASA, NB/70 K, AFP, afamin (AFM), collagen, prohibitin, keratin-6, PARC, B7-H4, YK-L40 |
The complex and multifaceted process of ovarian epithelial tumorigenesis necessitates the development of an equally complex tumor microenvironment. Within this microenvironment, tumor cells, stromal fibroblasts, and infiltrating leukocytes produce a multitude of autocrine and paracrine factors, including but not limited to cytokines, chemokines, growth factors, hormones, inflammatory mediators, and acute-phase reaction molecules. It is this complex signaling network which mediates tumor/stroma interactions and facilitates tumor proliferation, invasion, neoangiogenesis, and metastasis. As illustrated in Table 3.2, concentrations of numerous proteins involved in the above listed tumorigenic biological functions are altered in the circulation of ovarian cancer patients [130]. Mediators of inflammation, including cytokines and acute-phase reactants, were the most numerous among the various biological groups followed by adhesion molecules/proteases, growth/angiogenic factors, and hormones. Contrary to the commonly held notion that useful biomarkers represent proteins which are significantly elevated in the circulation of cancer patients, many of the identified proteins were observed to be significantly decreased in the ovarian cancer group in a number of studies (Table 3.3). These findings represent the complexity of signaling mechanisms at work in ovarian cancer development and a potential paradigm shift in biomarker research.
Table 3.3
Trends in serum biomarker alteration in three large studies
Biological function | Trend in OC | Biomarkers |
---|---|---|
Cytokines/chemokines | Elevated | IL-10a, IL-6a, TNF-αa, TNFR2a, 6Ckine, BCA-1, BLC/CXCL, CCL20/MIP-3α, CD40, CXCL9/MIG, IL-1Rα, IL-11, IL-16, IL-1β, IL-21, IL-28A, IL-2R, IL-33, IL-7, IL-8, LIF, MIF, MIP-1δ, RANTES, sCD137/4, TNFR1 |
Decreased | Eotaxin-1, eotaxin-2, IL-18, macrophage-derived chemokine, XCL1/lymphotactin | |
Growth/angiogenic factors | Elevated | IGFBP-1a, angiopoietin-2, β2-microglobulin, betacellulin, CTGF, bFGF, heparin-binding EGF-like growth factor, IGFBP-2, IGFBP-4, PDGF, VEGF |
Decreased | EGFRb, BDNFa, ErbB2a, IGFBP-3, IGFBP-6, IGF-I, PEDF, stem cell factor | |
Adhesion molecules/proteases | Elevated | CA-125a, CA 19-9a, OPNa, TIMP-1a, CA 15-3, CA 72-4, cathepsin D, MMP-9, tenascin C |
Decreased | VCAM-1a, MMP-2, MMP-3, NCAM | |
Hormones | Elevated | ACTH, GH, GLP-1, prolactin, TSH |
Decreased | Adiponectin, estrogen receptor α, FSH, ghrelin, GIP, insulin, leptin, LH, osteocalcin, thrombopoietin | |
Acute phase reactants/coagulation factors | Elevated | α1-Antitrypsina, CRPa, EN-RAGE, ferritin, fibrinogen, haptoglobin, HSA, PAI-1, protein S100-A12 (EN-RAGE), SAP, von Willebrand factor |
Decreased | a2-Macroglobulina, ApoA1a, apolipoprotein CIII, apolipoprotein H, clusterin, complement C3, complement C4, Factor VII, fetuin A, prealbumina | |
Apoptosis factors | Elevated | Cyfra 21-1 |
Decreased | Fas, perforin | |
Energy homeostasis | Elevated | AGRP |
Decreased | Creatine kinase-MB | |
Undefined or other functions | Elevated | Anti-TFF-3, FABP, HE4, NSE, NT-Pro-BNP |
Decreased | none |
Advances in Population-Based Screening for Ovarian Cancer
The development of multimarker panels for the diagnosis of ovarian cancer is currently advancing on two fronts. The first of these fronts includes investigators seeking improved tools for use in screening strategies for ovarian cancer in the general population. Given the limited performance of currently used imaging techniques and CA-125 testing as well as the overall rarity of ovarian cancer, routine population-based screening is not recommended by any of the major relevant professional societies [24]. It also remains unlikely that any stand-alone biomarker-based screening test will be capable of overcoming the 10 % PPV level required for implementation. However, work has persisted based on the notion that biomarker testing may prove effective in sufficiently defined high-risk groups or as part of a multimodal screening strategy involving TVU or an equivalent imaging method as a second-line test. The second front in the clinical use of ovarian cancer biomarkers pertains to the use of multimarker panels in the triage of women presenting with a pelvic mass. This aspect of early detection is discussed in depth in the next section.
Current State of Ovarian Cancer Screening: Clinical Trials
In addition to the PLCO and UKCTOCS screening trials, a number of additional clinical trials have examined the efficacy of imaging and CA-125. Imaging of the ovary has been proposed as a strategy to detect changes in size and architecture that might precede the development of symptoms and detection by pelvic examination. Studies involving healthy women have established the upper limit of ovarian size as greater than 5 cm and a set of morphologic characteristics of ovarian masses in order to identify malignant neoplasms [131, 132]. Therefore, considering the above model, these trials could be expected to preferentially diagnose cancer at invasive late stages, thus limiting their efficacy as screening tools [133]. In fact, several prospective studies of transvaginal ultrasonography that involved more than 136,000 women had mixed results, with some but not others showing a nonsignificant trend toward the diagnosis of ovarian cancer at an early stage [12, 32, 134–139]. The PPV of ultrasonography in these studies ranged from 1.0 to 27 % [137]. The interpretation of these results is complicated by differences with respect to inclusion criteria, with some studies enrolling only women at high risk (on the basis of a family history) [12, 135], some enrolling only women at average risk [32, 134, 138, 139], and others enrolling both women at high risk and those at average risk [136, 137].
An alternative strategy is to evaluate serum tumor markers for the early detection of ovarian cancer. This strategy is potentially attractive in that measurement of markers is broadly available, can be repeated at appropriate intervals, and is minimally invasive. In addition, biomarker testing does not depend on operator interpretation and is less costly than ultrasonography. As of yet, the most robust serum biomarker for detection of ovarian cancer is CA-125. In clinical trials, CA-125 as a single screening biomarker demonstrated limited utility when examined in a retrospective analysis of serum samples from 5,550 women enrolled in a population-based registry in Sweden [140]. Later it was suggested that measurement of CA-125 values in an individual patient over time could improve the estimation of a patient’s risk of ovarian cancer [141]. To evaluate CA-125 dynamics, the ROCA was developed based on the slope of serial CA-125 measurements drawn at regular intervals [34]. This algorithm was based on the observation that irrespective of the initial level, CA-125 measurements are stable in non-cases for periods of more than 5 years, indicating that each woman has her own baseline level of CA-125 [34]. In contrast, exponentially increasing serial values readily identify cases. When the ROCA score exceeds a 1 % risk of having ovarian cancer, patients undergo TVU to determine whether additional intervention is warranted. In a retrospective examination of 33,621 serum samples from 9,233 women, ROCA provided an SN of 86 % at a fixed SP of 98 % for the preclinical detection of ovarian cancer, as compared with an SN of 62 % for a single CA-125 value [34]. This algorithm was confirmed to have a reasonably high PPV (19 %) in a subsequent prospective pilot study involving more than 13,000 postmenopausal women [142]. This strategy is currently being prospectively studied among more than 2,600 high-risk women by the Gynecologic Oncology Group (protocol #199) as well as in a parallel trial being conducted by the National Cancer Institute-Cancer Genetics Network [143]. Another algorithm used in screening of high-risk women is the Parametric Empirical Bayes (PEB) algorithm [45, 144]. In the PEB model, each woman’s complete screening history, not only her most recent index value, acts as her own control, and the slightest deviation from that person-specific norm is used to detect elevated values at a fixed SP. The PEB model will assign the great majority of subjects lower thresholds, allowing their cancers to be detected earlier. Additionally, PEB allows for assessment of multiple markers.
Selection of Multimarker Panels Based on Postdiagnostic Samples
A number of studies have utilized different criteria for biomarker prioritization and selection (Table 3.4). In the study by Mor et al., 169 proteins were analyzed in serum samples obtained from ovarian cancer patients by means of RCA immunoassay microarray, and 35 of these proteins were found to be differentially expressed between cases and controls [79]. Biomarkers were further prioritized for independent ELISA testing if (1) similar levels of expression were observed in newly diagnosed patients and patients with recurrent disease; (2) ELISA tests were available commercially for these analytes; and (3) they had compelling biological reasons for their evaluation. These analytes were EGF, MIF-1, TNF-α, leptin, prolactin, IL-17, OPN, and IGF-II. Leptin, prolactin, OPN, and IGF-II showed perfect correlation between the RCA microarray immunoassays and the ELISA assays and were able, when used together, to discriminate the control and cancer training group samples with 95 % SN at 95 % SP. Adding CA-125 and MIF to this panel provided a classification power of 92 % SN at 99 % SP. This panel was equally sensitive to different histological subtypes of primary epithelial ovarian cancers: papillary serous, clear cell, endometrioid, and mixed [128]. Unfortunately, following a high level of initial enthusiasm and the subsequent marketing of this panel under the trade name OvaSure, deficiencies in study design have been identified which illustrate the challenges facing biomarker development efforts in general. The most prominent among these deficiencies was the inaccurate calculation of PPV based on improper estimates of ovarian cancer prevalence [143, 149]. This observation coupled with the lack of evaluation in a large prospective study led to performance revisions and the withdrawal of the commercial kit.
Table 3.4
Multimarker panels which discriminate ovarian cancer cases from healthy controls
Panel | Cases/controls | SN/SP | Analytical platform | Bioinformatics method | Biomarker prioritization | Year/reference |
---|---|---|---|---|---|---|
CA-125, ApoA1, TTR | 200/142b | 74/97 | MS | SVM | No | 2004 [129] |
CA-125, CA 72-4, M-CSF | 123/224b | 70/98 | ELISA | CT, MDA, LR | Yes | 2004 [127] |
CA-125, IL-6, IL-8, VEGF, EGF | 44/45 | 85/95 | BBIA | CT | No | 2005 [145] |
CA-125, TTR, ApoA1 | 20/82 | 89/92 | MS | LR | No | 2007 [146] |
CA-125, leptin, PRL, OPN, IGF-II, MIF | 156/362b | 95.3/99.4 | BBIA | LR | No | 2008 [128] |
CA-125, HE4, glycodelin, Plau-R, MUC1, PAI1 | 200/396 | 80–89/87 | BBIA | LR | Yes | 2008 [147] |
CA-125, HE4, CEA, VCAM-1 | 456/2,000b | 86–93/98 | BBIA | MMC | No | 2010 [43] |
CA-125, CA 19-9, EGFR, CRP, myoglobin, ApoA1, CIII, MIP-1α, IL-6, IL-18, tenascin C | 176/187b | 91.3/88.5 | BBIA | Knowledge Discovery Engine-VS | No | |
CA-125, CRP, SAA, IL-6, IL-8 | 150/212b | 94.1/91.3 | BBIA | LR | Yes | 2010 [126] |
CA-125, HE4, SIa | 74/137 | 84/98.5 | ELISA | N/A | Yes | 2010 [148] |
In the recent study by Havrilevsky et al. [147], a literature review was conducted for investigations describing transcriptional profiling of epithelial ovarian cancer. Candidate biomarkers meeting the following inclusion criteria were selected: (1) overexpression of candidate gene in epithelial ovarian cancer relative to normal ovarian epithelium; (2) overexpression of encoded protein in ovarian tissue; (3) localization of encoded proteins to extracellular compartment as membrane-bound or secreted protein; and (4) discrimination of ovarian cancer from normal sera utilizing prototype immunological assays. Correlation with CA-125 was not evaluated. Based on these criteria, eight genes, HE4, glycodelin (PAEP), MMP-7, MUC-1, PAI-1, and SLPI, Plau-R, and inhibin A, were selected as candidate markers for inclusion. Several combinations of these proteins were evaluated, and a combination of CA-125, HE4, glycodelin, Plau-R, and MMP7 was selected that offered 79 % SN at 93 % SP for early-stage ovarian cancer [147].
Yurkovetsky et al. utilized a subject cohort which included more than 2,000 healthy women in an unbiased analysis of serum biomarker candidates [43]. Feature selection was performed based on both bioinformatics and the following biological considerations: high accuracy in early-stage disease, equal classification of all ovarian cancer histologies, and selectivity for ovarian cancer versus benign disease and other epithelial cancers [43]. The identified panel of CA-125, HE4, CEA, and vascular cell adhesion molecule-1 (VCAM-1) was found to discriminate early-stage ovarian cancer from the control group with 86 % SN at 98 % SP.
In a multicenter case-control study, Zhang et al. utilized surface-enhanced laser desorption/ionization (SELDI) to analyze sera obtained from 195 patients with invasive epithelial or other types of ovarian cancer along with patients diagnosed with benign pelvic masses and healthy control women [129]. The investigators employed subsets of five separate centers for sequential components of their analysis. Two centers were designated for biomarker discovery, and candidate peaks from the proteomic analysis were identified by cross-validation. These candidate biomarker peaks were then validated in sera collected at two additional sites. Validated biomarker peaks were then identified and the corresponding proteins purified. Candidate biomarkers for which suitable immunoassays were available were then chosen for validation in sera collected at the fifth site. Analysis of immunoassay results led to the identification of a four-biomarker panel consisting of CA-125, ApoA1, transthyretin (TTR), and H418. In the validation set, this panel provided an SN of 74 % at an SP of 97 % for the discrimination of early-stage ovarian cancer from healthy women.
In a separate study, Skates et al. evaluated serum levels of four biomarkers by immunoassay in patients enrolled at 3 independent collection centers [127]. Sera collected at the first center constituted the training set, and biomarker data was analyzed using three statistical models: logistic regression, classification tree, and mixture discriminant analysis (MDA). The MDA model is unique in that it emulates the heterogeneity of ovarian cancer with respect to histological subtype and utilizes the distribution of biomarker levels within each subtype to estimate the histological type of each sample analyzed. The authors conclude that logistic regression and MDA modeling perform similarly and both outperform classification tree in the discrimination of ovarian cancer cases from controls. The combination of CA-125, CA 15-3, CA 72-4, and M-CSF, identified using the MDA model, performed best in the independent validation set, providing an SN of 70 % at 98 % SP for early-stage ovarian cancer.
Recently, Edgell et al. reported on a retrospective case-control study (Phase 2 biomarker study) in which the authors utilized a multiplexed bead-based immunoassay platform to evaluate five ovarian cancer biomarkers (CA-125, CRP, SAA, IL-6, IL-8) in 362 plasma samples obtained from ovarian cancer patients and healthy controls split into independent training and validation sets [126]. Through multivariate modeling the authors demonstrated SN/SP of 94.1/91.3 for the five-biomarker panel in the validation set and an SN/SP of 92.3/91.3 for all early-stage ovarian cancers.
In another recent study, Amonkar et al. demonstrated the value of multiplexed analysis in their evaluation of 104 candidate biomarkers in a cohort of over 350 ovarian cancer patients and controls [125]. The cohort represented various stages of disease and each of the most common histological subtypes of epithelial ovarian cancer and most prevalent benign ovarian conditions. Their training analysis led to the identification of an 11-analyte profile consisting of CA-125, CA 19-9, EGFR, CRP, myoglobin, ApoA1, ApoCIII, MIP-1α, IL-6, IL-18, and tenascin C. In an independent validation set, this panel provided an SN of 91.3 % at an SP of 88.5 %.
Multimarker Panels in Prediagnostic Samples
While many of the reports represented in Table 3.4 are initially encouraging, several hurdles remain before any of the identified models can be implemented clinically on a widespread basis. Foremost is the need to evaluate the most promising panels in prospective randomized clinical trials. Additional preclinical validation will be required to fully characterize the efficacy of selected panels before this significant next step is warranted. A key component of this validation process is the evaluation of panels in samples obtained from prediagnostic ovarian cancer patients. Progress toward this type of validation is greatly hindered by the rarity of this sample type; however, several significant findings have been reported by a group under the direction of Nicole Urban. In a pair of reports, this group first describes elevated levels of CA-125, HE4, and mesothelin in the sera of symptomatic ovarian cancer patients and then in the sera of patients 0–3 years prior to diagnosis, noting an optimal lead time of 1 year [150, 151]. In a separate study, the investigators utilize a combinatorial approach including CA-125 and HE4 measurements in addition to the Symptom Index (SI) to prospectively classify benign from malignant pelvic masses with an SN of 84 % at an SP of 98.5 % (Table 3.4) [148].
Recently, a collaborative study was performed to assess the prediagnostic performance of candidate biomarkers in the PLCO trial [152, 153]. Forty-one biomarkers selected by five leading ovarian cancer biomarker laboratories from Harvard University, Fred Hutchinson Medical Center, MD Anderson Cancer Center, University of Pittsburgh, and Yale University were utilized in the analysis of 118 cases and 473 matched healthy controls [153, 154]. The selection of these markers proceeded from a Phase 2 validation study using a common reference set of 160 ovarian cancer cases (approximately 50 % stage I/II) and 480 general population controls based solely on individual performance (SN at 95 % SP, AUC). The study demonstrated that none of these biomarkers or multimarker panels added any appreciable improvement in SN over that of CA-125 alone. In addition, levels of CA-125 began to increase, on average, less than 1 year prior to diagnosis with ovarian cancer (Fig. 3.1). Subsequent analyses revealed that 10 of the selected biomarkers, HE4, mesothelin, CA 15-3, CA 72-4, KLK6, spondin-2, TTR, Cyfra 21-1, IGFBP-2, and YKL-40, were strongly (r > 0.35) correlated with CA-125. This lack of feature independence could be credited as a limiting factor in the ability of these additional biomarkers to complement the diagnostic performance of CA-125. Similarly, Anderson et al. [151] measured several biomarkers over time in prediagnostic samples obtained from ovarian cancer patients and matched controls and observed that CA-125 (along with HE4 and mesothelin, but not of B7-H4, DcR3, and spondin-2) demonstrated appreciable increases in patients approximately 3 years before diagnosis. However, this CA-125 velocity reached detectable elevations only within the final year before diagnosis implying that a more sensitive assay could possibly detect early individual changes.
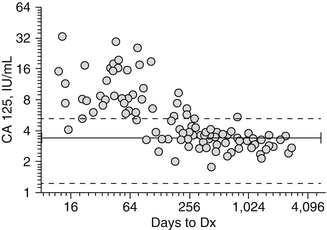
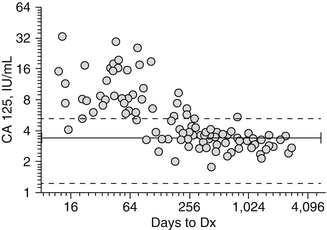
Fig. 3.1
Distribution of CA-125 concentrations in case samples from PLCO trial in relation to time of blood draw to diagnosis. Solid line mean for healthy controls, hatched line SD (minus outliers)
In nearly all reports, efforts aimed at novel biomarker development have relied upon the use of biological fluid samples collected at the time of first diagnosis. It would appear from the disappointing results of the PLCO study and others that this approach is not optimized for the development of prediagnostic tests offering a potential window of early successful intervention. Ongoing efforts of this type are unlikely to be productive, and the incorporation of new strategies focused on the characterization and detection of preclinical disease will be necessary for true advancement. Recent work suggests that a precursor lesion for serous carcinomas, termed serous tubal intraepithelial carcinoma (STIC), does indeed exist [155]. STICs originate from the fimbriated end of the fallopian tube, indicating a tubal, rather than ovarian epithelial, origin for serous carcinomas. The identification of the potential STIC precursor of serous carcinomas represents an important finding that may lead to advances in detecting these tumors at an earlier stage. Perhaps, analysis of blood samples from patients who had undergone prophylactic oophorectomy where preneoplastic lesions were discovered could prove useful although substantial effort would be needed to collect a statistically meaningful set of such samples. In fact, a recent publication based on mathematical modeling of ovarian cancer development using pathological findings from prophylactic bilateral salpingo-oophorectomies (PBSOs) suggested that ovarian cancers spend more than 4.3 years as in situ or stage I–II disease and that serous ovarian cancers have already progressed to a late stage by approximately 1 year prior to their discovery [133]. Therefore, if, as suggested above, changes in CA-125 levels are detectable within 3–12 months before clinical diagnosis, then current strategies are doomed to identify lower-volume high-grade disease, at best. Indeed, the authors conclude that in order to achieve 50 % SN in detecting tumors before they advance to stage III, an annual screen would need to detect tumors of less than 1.3 cm in diameter, whereas 80 % SN would require detecting tumors of less than 0.4 cm in diameter. No imaging modality could presently detect such small lesions, and therefore improved biomarker testing including the use of additional biomarkers and assays capable of detecting CA-125 with greater SN represents the most promising tools for early detection.
Advances in the Diagnosis and Triage of Malignant Pelvic Masses
The use of biomarker panels for the differential diagnosis of pelvic masses represents a clinical setting within the management of ovarian cancer where significant improvements could be achieved in the near future. According to current estimates, 1.4 % of women born today, or 1 in 72, will be diagnosed with ovarian cancer at some point in their lifetime. This year in the USA, there will be over 21,000 new cases of ovarian cancer along with nearly 14,000 deaths [156]. These cases arise from a much larger group of women presenting with pelvic masses. The overall prevalence of pelvic abnormalities is estimated at 7 %, and it is expected that 5–10 % of American women will receive prophylactic surgery for suspected ovarian cancer at some point in their lives [157]. Effective preoperative risk assessment of the pelvic mass is currently an area of great unmet need. At present, a patient’s age and menopausal status are the most important factors to consider upon the identification of an pelvic abnormality as the associated risk of malignancy increases from 13 % in premenopausal women to 45 % in postmenopausal women [158].
Although the vast majority of women diagnosed with ovarian carcinoma will initially present with a pelvic mass, only a small proportion of all masses detected will prove to be malignant. The expeditious triage of these patients often represents the most important component of their clinical management. The burden of early identification of potential ovarian cancer falls predominantly upon the obstetrician/gynecologist whose training in the management of cancer patients is usually limited. While these practitioners can effectively manage the high percentage of patients diagnosed with functional cysts and benign neoplasms through observation and surgery, respectively, the clinical outcome for a patient presenting with a malignant mass can be drastically worsened if she is not immediately referred to a gynecological oncologist [159–161]. A series of diverse studies have demonstrated a decrease in the relative risk of reoperation and increases in disease-free interval and overall survival for women operated on by gynecological oncologists compared with gynecologists and general surgeons [162–164]. The overall prognosis for patients diagnosed with ovarian cancer has improved significantly over the past 30 years, demonstrated by an increase in 5-year survival from 20 to 50 % [165, 166]. This improvement can be largely attributed to the development and application of cytoreductive surgical techniques and the optimization of chemotherapeutic regimens. Despite these findings and advancements, referral rates remain disappointingly low for patients diagnosed with a pelvic mass [167]. Improvements upon current screening methodologies and the emergence of new techniques should aid gynecologists and general practitioners in making appropriate referral decisions leading to improvements in the overall clinical management of ovarian cancer.
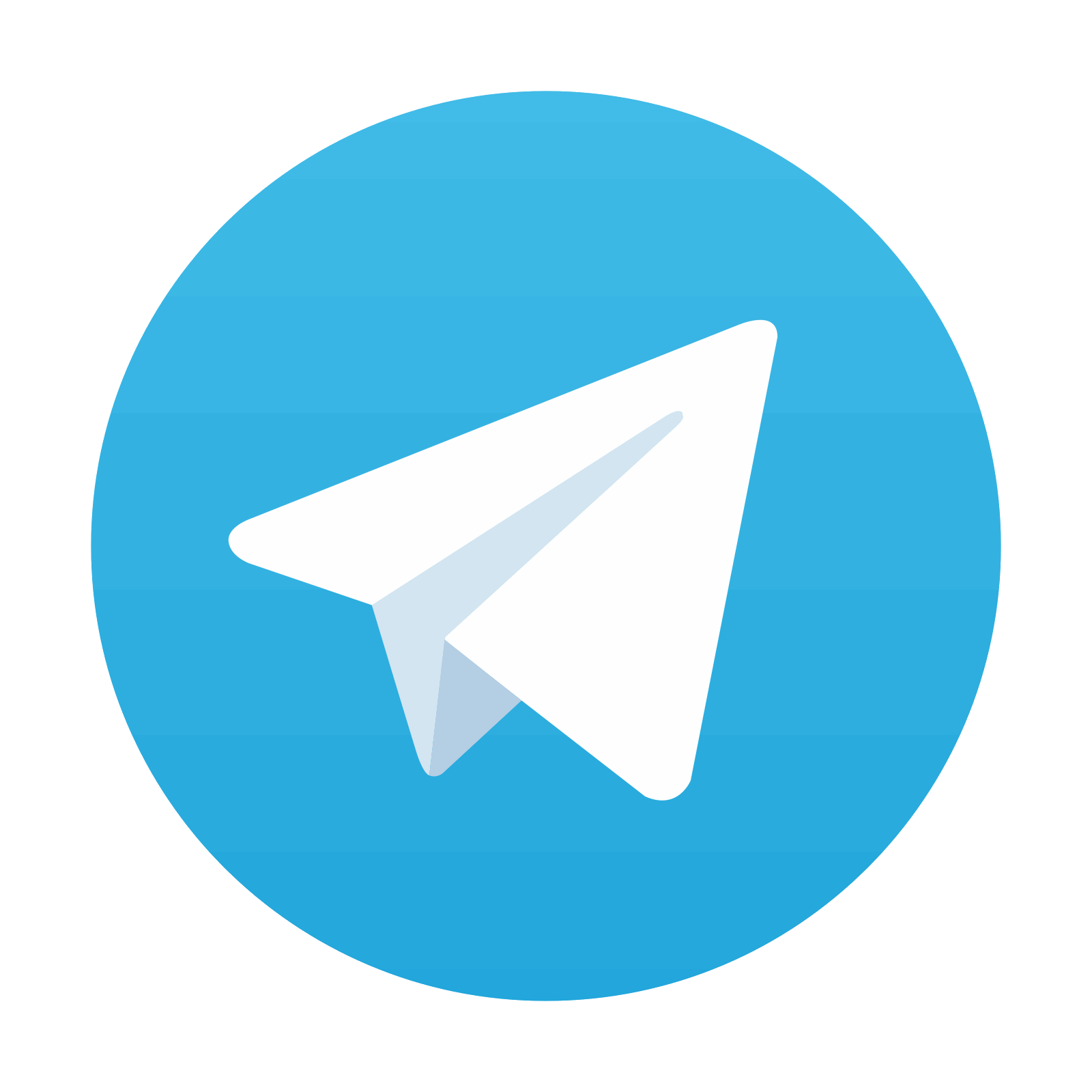
Stay updated, free articles. Join our Telegram channel

Full access? Get Clinical Tree
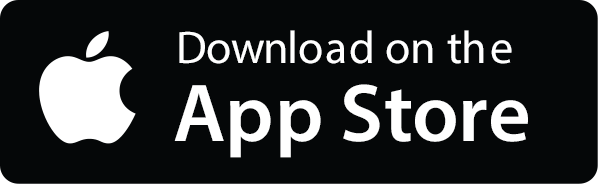
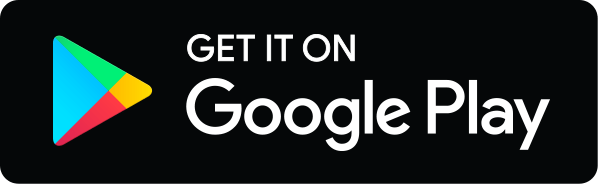