HR pathway proficient tumors with CCNE1 amplification were common in primary resistant and refractory cases [7]. Inactivation of the p53 pathway and activation of the CCNE1 pathway also contribute to chromosomal instability [16]. Alterations in nucleotide excision repair (NER) and mismatch repair (MMR) have been reported in up to 8% and 3% of high-grade serous ovarian carcinomas, which tumors are sensitive to platinum and resistant to PARP inhibitor [17].
Mechanism of acquired resistance to chemotherapy included breakage of tumor suppressor genes, reversion mutation of BRCA1/2 mutated cases, and upregulation of BRCA1 gene expression by demethylation of the methylated BRCA1 promoter region in a primary tumor. Additionally, gene fusion of ABCB1 with SLC25A40 promoter caused upregulation of ABCB1 expression, which can cause increased excretion of chemotherapeutic agents [7] (see also Sect. 3.4.1.1 in Chap. 3).
7.2.3 Experiments to Identify Origin of High-Grade Serous Ovarian Carcinoma
Recently, fallopian tubal epithelial cell has been thought as the origin of high-grade serous ovarian carcinoma [1]. Using a genetically engineered mouse that expresses Cre recombinase from a Pax8 promoter, Brca, Tp53, and Pten genes were targeted in fallopian tubal secretory epithelial cells. This mouse model generated serous tubal intraepithelial carcinoma as the precursor lesion that gave rise to high-grade serous ovarian and peritoneal carcinomas [18]. In this model, tumor-bearing mice had higher serum CA125 levels than controls. Furthermore, the tumors had extensive copy number alterations similar to human high-grade serous ovarian carcinomas.
There is another idea regarding cell of origin of high-grade serous ovarian carcinoma. Cells of the hilum ovarian surface epithelium, the transitional area between the ovarian surface epithelium, mesothelium, and tubal epithelium, express stem cell markers and display stem cell properties. The hilum cells show increased transformation potential after inactivation of tumor suppressor genes Tp53 and Rb1. Therefore, stem cell niches in those areas are susceptible to malignant transformation and could be the origin of high-grade serous ovarian carcinoma [19].
7.3 Ovarian Clear Cell Carcinoma
7.3.1 Gene Expression of Ovarian Clear Cell Carcinoma
Ovarian clear cell carcinoma shows unique clinical features including an association with endometriosis and poor prognosis. A gene expression microarray analysis identified genes commonly expressed in both ovarian clear cell carcinoma cell lines and clinical samples, which comprise an ovarian clear cell carcinoma gene signature. The gene signature contains known markers of ovarian clear cell carcinoma, such as HNF1B, VCAN, IL6, and other genes that reflect oxidative stress. Expression of ovarian clear cell carcinoma signature genes was induced by treatment of immortalized ovarian surface epithelial cells with the contents of endometriotic cysts, indicating that the ovarian clear cell carcinoma signature is largely dependent on the tumor microenvironment [20].
7.3.2 DNA Methylation Analysis of Ovarian Clear Cell Carcinoma
Recently, genome-wide methylation and expression data were generated for 14 ovarian clear cell carcinoma, 32 non-ovarian clear cell carcinoma, and four normal cell lines. Consensus clustering showed that ovarian clear cell carcinoma is epigenetically distinct. Inverse relationships between expression and methylation in ovarian clear cell carcinoma were identified, suggesting functional regulation by methylation, and included 22 hypomethylated genes and 276 hypermethylated genes. The ovarian clear cell carcinoma-specific hypomethylated genes were involved in response to stress and many contain HNF1-binding sites, while the ovarian clear cell carcinoma-specific hypermethylated genes included members of the ERα network and genes involved in tumor development [21].
7.3.3 Genetic Analyses of Ovarian Clear Cell Carcinoma
ARID1A mutations were reported in 46–57% and PIK3CA mutations in 31–33% of ovarian clear cell carcinoma samples [22–24]. A whole-exome sequencing of 39 ovarian clear cell carcinoma samples identified recurrent somatic mutations in 426 genes [25]. In these 39 samples, ARID1A (62%) and PIK3CA (51%) were frequently mutated, and known key ovarian clear cell carcinoma-related genes such as KRAS (10%), PPP2R1A (10%), and PTEN (5%), as well as novel genes MLL3 (15%), ARID1B (10%), and PIK3R1 (8%) were also mutated. Gene interaction analysis and functional assessment revealed that mutated genes were clustered into groups pertaining to chromatin remodeling, cell proliferation, DNA repair and cell cycle checkpointing, and cytoskeletal organization.
A copy number variation analysis based on the above exome sequencing identified frequent amplification of MYC (chr8q, 64%), ZNF217 (chr20q, 54%), and ERBB2, STAT3, HNF1B, PPM1D (chr17q, 46%) loci as well as deletion in SMARCA4 (chr19p, 41%), RB1 (chr13q, 28%), NOTCH1 (chr9q, 21%), and SMAD4 (chr18q, 21%) loci. Other copy number alterations included amplification of IL6, IL6R, KRAS, PIK3CA, PIK3C2B, CDK2, CDK4, and CCNE1, as well as deletion of ARID1A, SMARCC1, SMARCA2, ARID1B, CDKN1A, CDKN2A, CDKN2B, and TP53. Integration of the analyses discovered that frequently mutated or amplified/deleted genes were involved in the KRAS/PI3K signaling (82%) and MYC/RB signaling (75%) pathways as well as the critical chromatin remodeling complex SWI/SNF (85%) [25] (see also Sect. 3.4.3 in Chap. 3).
7.3.4 Role of ARID1A, PIK3CA, and IL6 in the Carcinogenesis of Ovarian Clear Cell Carcinoma
Concurrent Arid1a inactivation and Pik3ca activation in mouse ovaries generated adenocarcinomas similar to human ovarian clear cell carcinomas. These tumors expressed Hnf1b, a marker of ovarian clear cell carcinoma. Furthermore, in this model, the tumor growth was promoted through sustained IL6 overproduction [26].
Ovarian clear cell carcinoma was generated in vitro by introducing ARID1A knockdown and mutant PIK3CA into a normal human ovarian epithelial cell line. Loss of ARID1A impairs the recruitment of the Sin3A-HDAC complex, while the PIK3CA mutation releases RelA from IκB, leading to NF-kB pathway activation resulting in IL6 overexpression [27].
Collectively, these findings indicate that ARID1A and PIK3CA mutations, frequently seen in ovarian clear cell carcinoma, are sufficient to generate ovarian clear cell carcinoma, associated with the specific gene expression including HNF1B and IL6 (see also Sect. 3.4.3 in Chap. 3).
7.4 Ovarian Endometrioid Carcinoma
7.4.1 Genetic Analysis of Ovarian Endometrioid Carcinoma
Gene mutations in ovarian endometrioid carcinoma samples with different grades (grade 1, n = 20; grade 2, n = 26; grade 3, n = 26) were analyzed, and mutations in CTNNB1 (13%, 5%, 0%), APC (5%, 0%, 0%), KRAS (10%, 12%, 0%), PTEN (20%, 8%, 0%), PIK3CA (20%, 8%, 0%), and TP53 (15%, 46%, 65%) were found [28]. Therefore, high-grade ovarian endometrioid carcinomas are likely to harbor TP53 mutations, while low-grade ovarian endometrioid carcinomas frequently harbor mutations of Wnt/β-catenin pathway and/or KRAS/PI3K pathway genes. In another study, ARID1A mutations were reported in 10 of 33 ovarian endometrioid carcinomas (30%) [23]. Another group reported mutations of CTNNB1 (53%), PIK3CA (40%), ARID1A (30%), PTEN (17%), KRAS (33%), PPP2R1A (17%), and TP53 (7%) in low-grade (grade 1 and 2) ovarian endometrioid carcinomas (n = 30) [29]. Activating mutations of the CTNNB1 gene is associated with squamous differentiation [30].
High-grade endometrioid carcinoma tumors with TP53 mutations have expression profiles similar to those of high-grade serous carcinoma [31]. However, these tumors may have been misclassified, as suggested by more recent studies reporting a subset of high-grade serous carcinomas that display a pseudoendometrioid pattern [32] (see also Sect. 3.4.2 in Chap. 3).
7.4.2 Mouse Models of Ovarian Endometrioid Carcinoma
Like ovarian clear cell carcinomas, ovarian endometrioid carcinomas are frequently associated with endometriosis. Peritoneal endometriosis occurs in mice by the activation of an oncogenic K-ras. Additionally, expression of oncogenic K-ras and Pten deletion within the ovarian surface epithelium leads to the induction of adenocarcinomas similar to human ovarian endometrioid carcinomas [33]. In another study, inactivation of the Pten and Apc in murine ovaries resulted in the formation of endometrioid adenocarcinomas [28]. More recently, codeletion of Arid1a and Pten resulted in ovarian endometrioid carcinoma [34].
7.4.3 Microsatellite Instability (MSI) in Ovarian Endometrioid Carcinoma
Ovarian cancer, particularly endometrioid adenocarcinoma, is associated with Lynch syndrome, although the risk is much smaller than for uterine cancer. Among 71 cases with ovarian endometrioid adenocarcinoma, 7 (10%) tumors had abnormal mismatch repair (MMR) protein status, defined as complete loss of expression of MLH1, MSH2, MSH6, and/or PMS2. Each of these tumors with abnormal MMR status demonstrated MSI. Importantly, concurrent uterine tumor was present in 5/7 patients whose ovarian tumor had abnormal MMR/MSI [35].
7.4.4 Genetic Analysis of Synchronous Endometrial and Ovarian Carcinoma
Five to ten percent of women with ovarian endometrioid carcinomas present with concurrent endometrial carcinoma. Based on both targeted and exome sequencing of 18 synchronous endometrial and ovarian tumors, most (17/18) cases showed evidence of clonality. Importantly, 10 of 11 cases that fulfilled clinicopathological criteria that would lead to classification as independent endometrial and ovarian primary carcinomas showed evidence of clonality [36]. Therefore, the genome-wide analysis demonstrated that most synchronous endometrial and ovarian carcinoma tumors develop from a clonal origin.
7.5 Mucinous Ovarian Tumors
7.5.1 Origin of Mucinous Ovarian Tumors
Mucinous ovarian carcinomas typically display heterogeneity, with lesion of mucinous cystadenoma admixed with borderline tumor and carcinoma. The identical KRAS mutation in these components provides strong evidence that mucinous cystadenomas are the precursor lesions of mucinous carcinoma [37, 38].
In terms of the origin of mucinous cystadenoma, a subset develops from mucinous epithelium in mature teratomas. A microsatellite genotyping analysis of mucinous tumors associated with a teratoma revealed five of six pairs of tumors with teratoma showed a high or complete degree of allelotype matching, which differed from the somatic allelotypes of the normal control tissue [39].
It has been proposed that many of nongerm cell mucinous tumors are derived from Brenner tumors. In a study of 40 mucinous cystadenomas, 67 Brenner tumors, and 13 combined tumors, a total of 25% of tumors with a mucinous component contained a Brenner component, and 16% of tumors with a Brenner component contained a mucinous component. Mucinous tumors are typically large, whereas Brenner tumors tend to be smaller. Accordingly, the Brenner tumor is compressed by the large mucinous cystadenoma and may be overlooked [40]. This hypothesis was supported by a recent study showing that, in combined Brenner and mucinous tumors, the Brenner and mucinous components are clonally related [41] (see also Sect. 3.4.4 in Chap. 3).
7.5.2 Genetic Features of Mucinous Ovarian Tumors
KRAS-activating mutation is the most common single molecular genetic alteration in mucinous carcinomas, occurring in 65% of cases [42]. Another study identified mutations in a novel gene, RNF43, a zinc finger-dependent E3 ubiquitin protein ligase. RNF43 mutations were observed with a frequency of 2/22 (9%) in mucinous ovarian borderline tumors and 6/29 (21%) in mucinous ovarian carcinomas [43]. In contrast to other type I ovarian carcinomas, TP53 mutation is frequent in mucinous carcinomas, being present in approximately one-half of cases [42, 43]. In a genetic analysis of a total of 82 mucinous ovarian tumors, which included exome sequencing of 24 tumors and a validation cohort of benign 58 tumors for specific gene regions, benign, borderline, and carcinoma samples harbored mutations in BRAF (0%, 10%, 23%), TP53 (9%, 14%, 52%), and RNF43 (0%, 7%, 20%), respectively, which mutations were associated with progression of the disease. Other recurrent, but not associated with progression, mutations were found in KRAS (54%), CDKN2A (16%), ARID1A (8%), ELF3 (6%), GNAS (6%), ERBB3 (5%), and KLF5 (5%) [44].
Overexpression and amplification of ERRB2 was observed in 11/176 (6%) mucinous borderline tumors and 29/154 (19%) mucinous cancers. KRAS mutations and ERRB2 amplification are near mutually exclusive (#41#). Thus, mutations in KRAS, BRAF, and/or ERRB2 amplification are present in the majority of mucinous neoplasms, indicating RAS/RAF pathway activation is frequent in this tumor. (See also Sect. 3.4.4 in Chap. 3).
7.6 Serous Borderline Tumor and Low-Grade Serous Ovarian Carcinoma
It has been well established that low-grade serous ovarian carcinomas can develop from serous borderline tumor. Deletions of ch1p36 and ch9p21 are much more common in low-grade serous ovarian carcinomas than in serous borderline tumors [45]. The ch1p36 region contains several candidate tumor suppressor genes including miR-34a. Then, the ch9p21 region including the CDKN2A/B locus encodes three tumor suppressor proteins, p14 (Arf), p16, and p15. Thus, deletions of ch1p36 or ch9p21 may cause progression of some serous borderline tumors to low-grade serous carcinomas.
KRAS mutations occur in one-third of serous borderline tumors and log-grade serous ovarian carcinomas, and BRAF mutations occur in another one-third of serous borderline tumors but less commonly in low-grade serous ovarian carcinomas [46, 47]. BRAF-mutated advanced-stage low-grade serous ovarian carcinomas are much less common than are BRAF-mutated advanced-stage serous borderline tumors [48–50]. ERBB2 and NRAS mutations are also detected in a small percentage of low-grade serous ovarian carcinomas [47, 51]. These mutations result in activation of the MAP kinase signal transduction pathway. Exome sequencing analyses also identified BRAF and KRAS as the most frequently mutated genes (#43#, #44#).
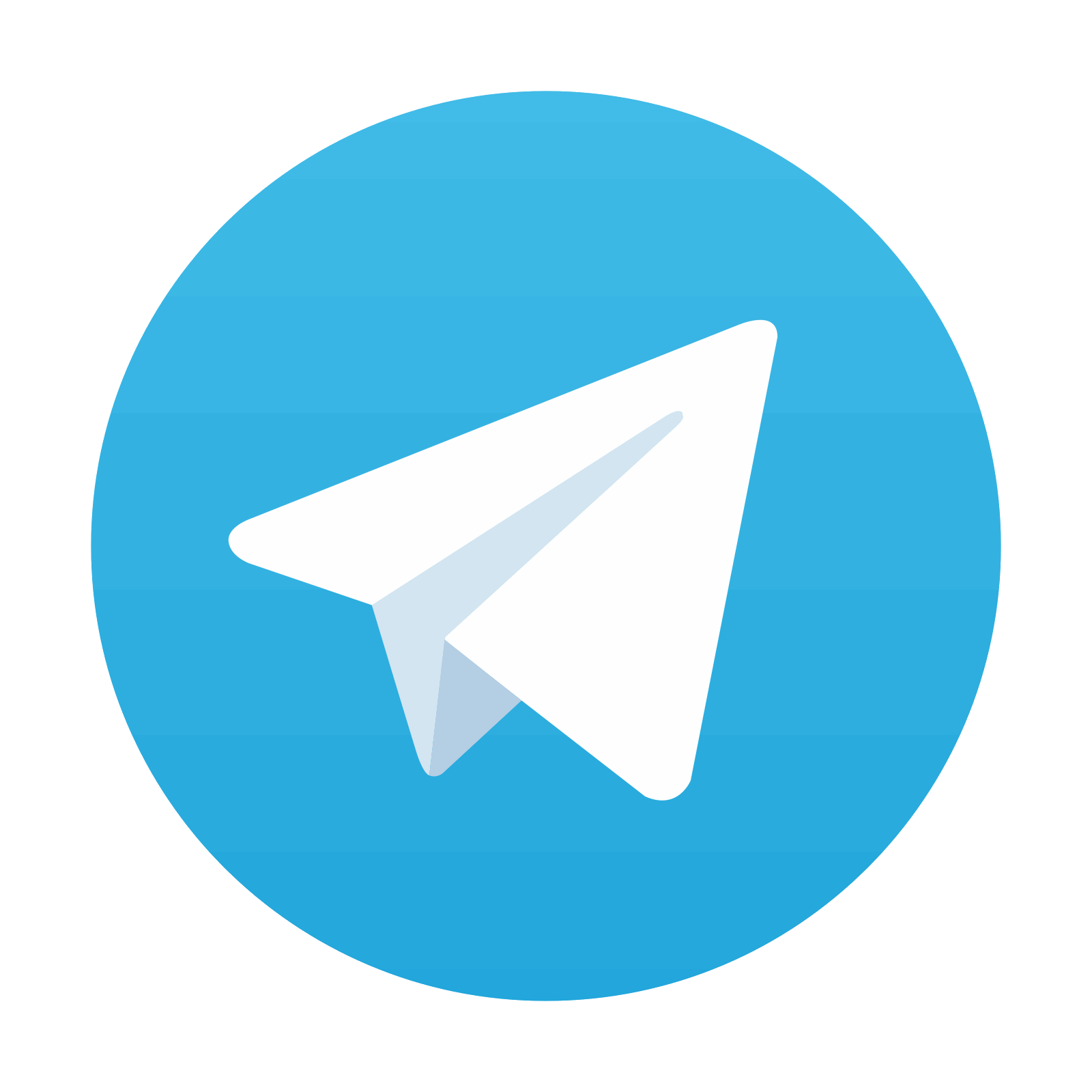
Stay updated, free articles. Join our Telegram channel

Full access? Get Clinical Tree
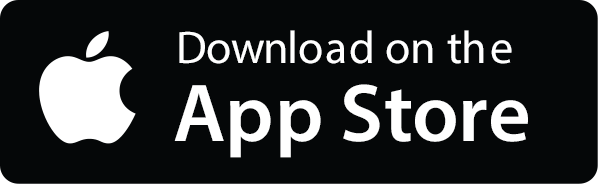
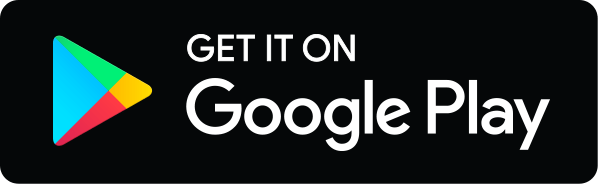