What is osteosarcopenic adiposity/obesity and what is in its name?
Introduction
Osteosarcopenic adiposity/obesity (OSA/OSO) was officially identified in 2014, when a theoretical concept supported by scientific evidence and research findings, hypothesized its existence and identification as a new condition/syndrome . At that time, it was described as a syndrome in older Caucasian women and subsequently linked to their lower functionality . Since then, OSA has been recognized and studied throughout the world and identified in various populations, age groups, and individuals with different health conditions . The original description of the condition still holds; however, the name was revised from osteosarcopenic obesity (OSO) to osteosarcopenic adiposity (OSA) . The rationale for changing the name is discussed below, but in this paper, the terms (OSA/OSO) are used interchangeably, especially since most of the previous literature refers to the condition as OSO. However, the original working definition did not change: “It is a condition encompassing simultaneous deterioration of bone (osteopenia/osteoporosis) and muscle (sarcopenia/dynapenia), with the increased presence of body fat (adipose tissue), either as an overt overweight/obesity and/or as redistributed fat (particularly in visceral areas) and/or as infiltrated fat (ectopic) into bone and muscle” .
Fig. 1 depicts body composition impairments, where any of the conditions (osteopenic adiposity, osteopenic sarcopenia, sarcopenic adiposity) may result in different stages of body composition derangement, leading ultimately to the most detrimental impairment; the triad of OSA. Also, each of the conditions may arise where the fat infiltration is predominant or where the fat accumulation originated. For example, osteopenic adiposity would be the result of fat infiltrating in bone, due to a multitude of causes, including diet and lifestyle, aging, inflammation, or other comorbidities, leading to lower bone mass/quality and increased fracture risks. Similar effects may cause fat infiltration into muscle, lowering muscle mass/strength, resulting in sarcopenic adiposity and causing functional disabilities and frailty. In this context, OSA is considered the most advanced impairment in bone, muscle, and body fat . Actually, there is the possibility that osteoporosis, sarcopenia, osteopenic adiposity, and sarcopenic adiposity are the same disease at different stages of development into OSA. The important point is that the key consequences of OSA are functional disabilities, increased fracture risks, and increased systemic metabolic deregulation leading to various morbidities.
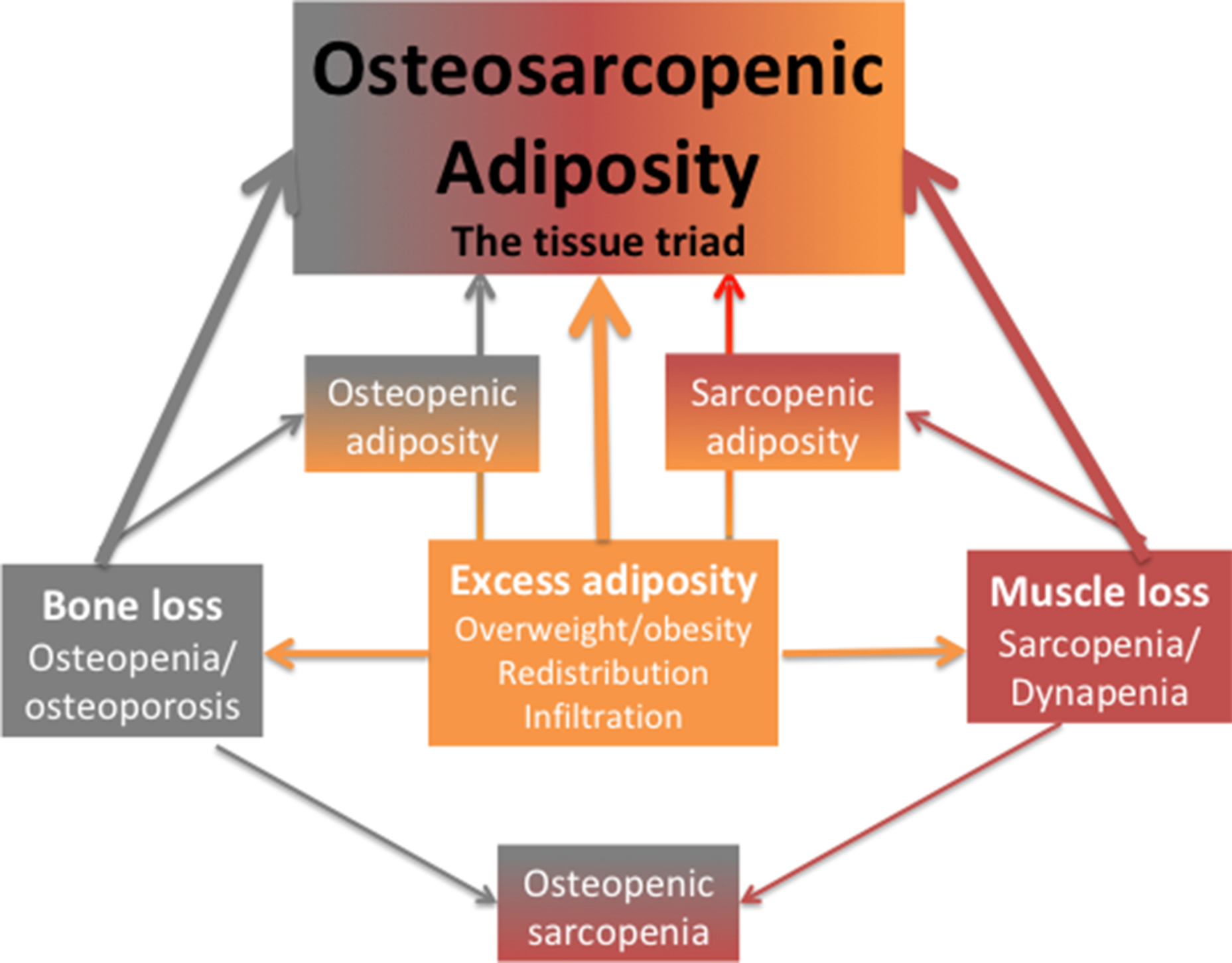
Why the name change?
In the context of this text, and in general terms, body composition includes bone, muscle (or lean tissue as its proxy), and adipose tissues. The criteria to diagnose osteopenia/osteoporosis, based on the dual-energy X-ray absorptiometry (DXA), are well known and widely accepted. To a certain extent, that is also true for sarcopenia, although there are a few working definitions. The most widely accepted one is from the European Working Group on Sarcopenia in Older People . However, there is still no global consensus on cut-offs for obesity or the amount of adipose tissue present in the whole body or its different compartments, as recently addressed . Most of the reasons are due to the high level of heterogeneity of adipose tissue which encompasses visceral, subcutaneous, and ectopic (infiltrated in liver, heart, bone, muscle) fat, as well as white, brown, and beige fat cells, each with different metabolic pathways and molecules secreted. Therefore, the difficulties in identifying either osteopenic/osteoporotic or sarcopenic obesity/adiposity, in addition to reaching the consensus on the cut-off criteria for overall body adiposity become apparent.
Generally, it could be stated that obesity typically refers to overweight, as most often defined by body mass index (BMI) ≥ 30 kg/m 2 with all limitations attributed to BMI , while body adiposity includes all types of fat discussed above. With that regard, and aligned with our original OSA/OSO definition, some argue that there should be separate terms to include osteosarcopenic subcutaneous obesity and osteosarcopenic visceral obesity . While these terms might provide a clearer picture of the OSA/OSO phenotype, they still do not include ectopic fat infiltrated in bone and muscle, probably the most important aspects in OSA/OSO syndrome, due to their metabolic effects. In view of the heterogeneity of adipose tissue, we believe that the more appropriate term for osteosarcopenic obesity (OSO) should be osteosarcopenic adiposity (OSA), but again, in this text, the terms are used interchangeably.
The proof of concept
In our original concept paper , we outlined the cellular, endocrine, and whole-body connections among bone, muscle, and body adiposity. Subsequently, as research in OSA evolved, the possibilities of how each tissue (or in combination with others) may contribute to its development are briefly summarized below .
Cellular connections and interactions among three tissues
There is an increasing understanding and appreciation for cellular connections and interactions among bone, muscle, and adipose tissues and the recognition that they should be studied together. With that regard, we summarized some possible pathways for a better understanding of their interactions and connections. For example, as our stem cells age or are exposed to other stressors caused by unfavorable micro or macro environments, they may take different lineage commitments, ultimately producing more adipocytes in bone, muscle, as well as in fat tissue itself . In this context, it is particularly important to focus on mesenchymal stem cells (MSC) as precursors for osteoblasts and adipocytes and on myogenic lineage from mesoderm as precursors for myocytes. In short, the stem cells’ normal regulatory commitments when affected by adverse metabolic processes in the body, will be disrupted and along the way, both bone and muscle cells may be replaced by fat cells.
As already established, the major osteoblastogenesis and osteoblast maturation and differentiation promoter is runt-related transcription factor 2 (RUNX2) along with the musculoaponeurotic fibrosarcoma oncogene homolog (Maf) . In addition, RUNX2 is also essential in shifting the MSC differentiation from adipogenic (slightly favored even under normal conditions) to osteoblastogenic lineage. The key signaling molecules regulating MSC toward myogenesis and promoters of MSC/satellite cells differentiation into myocytes are myogenin and myoD . The nuclear peroxisome proliferator-activated receptor gamma (PPAR-gamma) is an ultimate promoter of adipogenesis, adipocyte maturation, and metabolism and it hinders both osteoblastogenesis and myogenesis .
Regarding the master cell regulators, transforming growth factor-beta (TGF-beta) is the one that maintains the MSC population and promotes both osteoblastogenesis and myogenesis, but inhibits adipogenesis . Insulin growth factor-1 (IGF-1) exerts similar regulations . On the contrary, tumor necrosis factor-alpha (TNF-alpha), a major proinflammatory cytokine produced by visceral adipocytes, promotes adipogenesis and reduces osteoblastogenesis and myogenesis .
Endocrine connections and interactions
Each of the three tissues—bone, muscle, and fat, exerts a specific regulatory action on another one with possible systemic effects. Regarding the crosstalk between bone and muscle, the mechanical loading of muscle exerted on the bone (muscle as a puller, bone as a lever) has been investigated the most and previously believed to be the only connection between two organs. Recently, more insight is gained on bone and particularly on muscle acting as endocrine organs. For example, osteocalcin, a protein secreted by osteoblasts and best known as a bone formation marker, but also coined as the first osteokine , is anabolic for muscle and has a role in energy metabolism by regulating insulin sensitivity and glucose utilization, as well as adiponectin secretion by adipocytes . A marker of bone resorption, sclerostin, also causes muscle breakdown, while other molecules, like receptor activator of nuclear factor kappa-B ligand (RANKL) released during bone resorption, has negative effects on both bone and muscle . Other bone cells, including osteocytes and their secretory molecules, have been implicated in muscle and other organ regulations, as recently reviewed .
Muscle-secreting myokines may influence bone, as well as other organs. For example, myostatin is a major bone and muscle catabolic agent, along with the skeletal muscle-specific troponins (sTnT), the latter including a family of key regulatory contractility proteins which are released only during muscle damage . Interleukin 6 (IL-6) is well known for its strong proinflammatory effects in the systemic circulation, however, when released from the working muscle it exerts antiinflammatory properties and improves glucose utilization and satellite cell proliferation, but also decreases the low-grade chronic inflammation and indirectly bone loss . Other myokines released from the working muscle, interleukins IL-7, and IL-8 mediate fatty acid beta-oxidation, while IL-15, improves glucose utilization, indicating the common link between muscle and fat tissues . Additionally, the irisin-hormonal-system provides probably the best example of muscle-fat axis interaction. Irisin, secreted from the exercising muscle, causes the browning of the white fat cells converting them into the beige fat cells , the latter characterized by increased mitochondrial numbers and the expression of uncoupling protein-1, exerting higher thermogenesis. Although irisin effects and pathways are still under investigation, they may explain the higher energy expenditure occurring with exercise, which could not have been expounded by energy expenditure from exercise only .
Regarding the endocrine role of adipose tissue, probably the most notable is its endogenous estrogens formation via extra glandular aromatization of androgens. These estrogens are the only ones circulating in postmenopausal women, thus providing beneficial signals to both bone and muscle, and inhibiting adipose tissue accumulation . However, the complex actions of adipose-derived adipokines, namely leptin and adiponectin, are still not quite elucidated. Briefly, leptin and adiponectin have an opposing relationship in circulation, have receptors on both osteoblasts and myocytes, and exert dual effects on bone and muscle, mostly depending on whether their influence is on local or systemic levels . Due to these complexities, it is hard to deduce some general statement about the actions of these two adipokines on bone and muscle, especially in view of their possible involvement in other organ systems and interactions with multiple other molecules.
The adverse influence of the visceral fat and its secretory molecules are widely known and confirmed, not just in bone and muscle deterioration, but also in multiple other organ systems. This is especially important to address in the COVID-19 era (as discussed below). Visceral fat releases multiples of inflammatory cytokines, including tumor necrosis factor (TNF)-alpha, Il-1 and Il-6, C-reactive protein, fibrinogens, and lipoprotein-associated phospholipase, all producing an “inflammatory shift,” causing not just the damage to bone and muscle, but to the whole body organ systems . These are some of the same inflammatory cytokines released in a “cytokine storm” that viruses such as COVID-19 trigger, as discussed in the further text.
Whole-body levels
The inevitable changes in body composition occurring with aging, but possibly accelerated by other chronic conditions (e.g., low-grade chronic inflammation, chronic stress, diabetes, some cancers) cause the decrease in bone mass leading to osteopenia/osteoporosis and/or decrease of muscle mass and strength leading to sarcopenia. At the same time, there is an increase in body adiposity, redistribution of fat mass, and its infiltration into bone and muscle. These changes may lead to any of the body composition disturbances and ultimately to osteosarcopenic adiposity (see Fig. 1 ) with all its detrimental consequences .
Fig. 2 depicts the progression of OSA from cellular/endocrine/whole body changes to clinical manifestations.
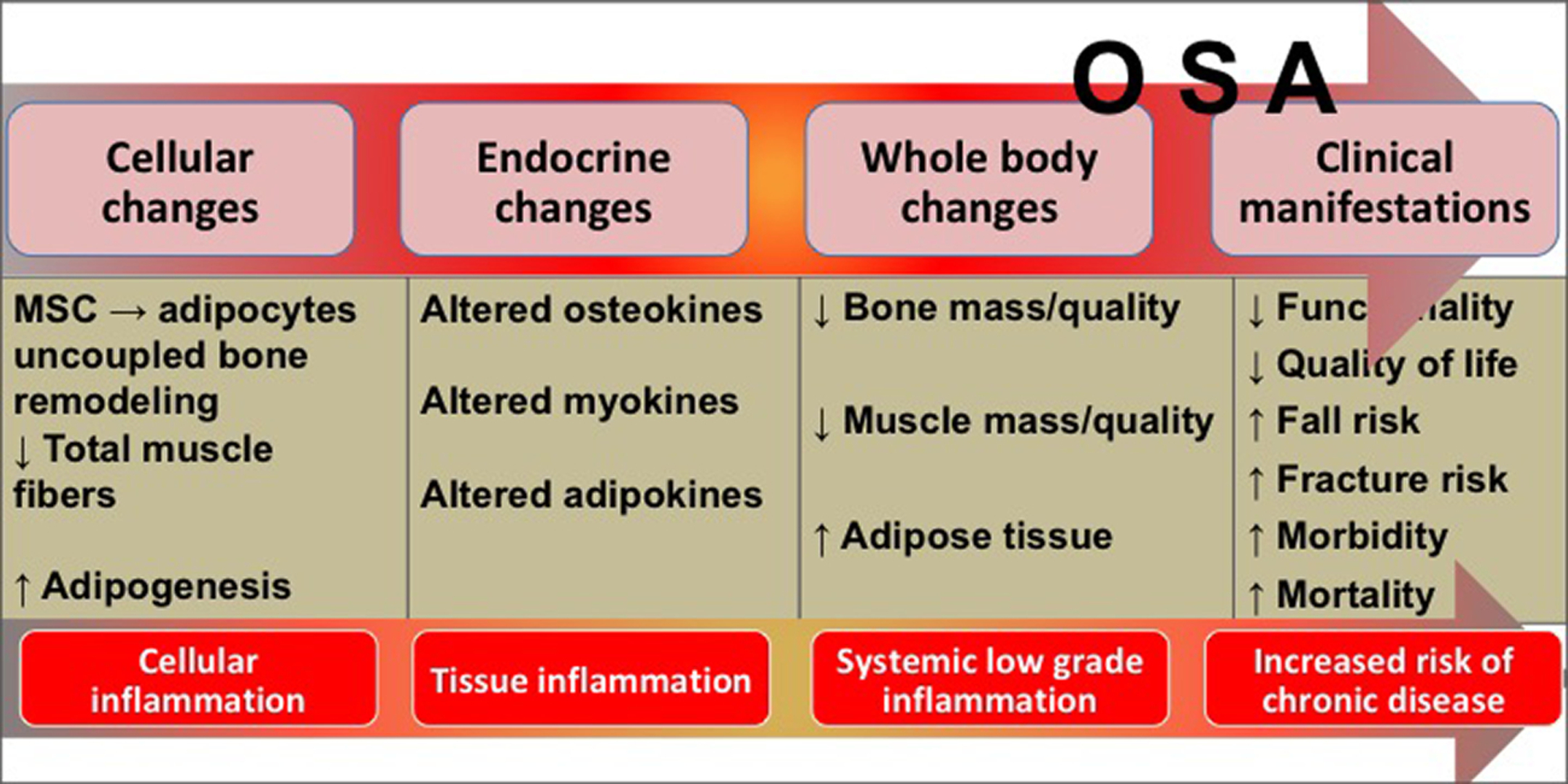
Identification of OSA and persisting difficulties
The updated diagnostic criteria for both men and women have been recently published . Since OSA is a complex condition involving multiple organs and tissues (each also limited to its own individual diagnosis), it is reasonable to expect that its identification has been difficult and still poses problems. The diagnosis based on deteriorations of body tissue could be performed when clinically relevant stages of bone loss, muscle loss, and fat gain are detected and assessed with some of the available technologies, as discussed below. The tests on functional performance could even precede any of the screening for body composition impairments, as outlined previously . When both of these measurements (bone/body composition and functional performance) are available, they can provide a more comprehensive way to grade the extent of the physical and functional deterioration in diagnosing OSA syndrome.
DXA technology has been a gold standard for osteopenia/osteoporosis diagnosis, as well as for the assessment of lean and fat tissue, for many years . However, the limitations of DXA for bone assessment are well recognized; it yields only areal bone mineral density (BMD), does not distinguish between trabecular and cortical bone (except the rough assumptions based on which parts of the skeleton are analyzed), and provides no information about bone structure/quality, thus limiting predictability for fracture risks. Additionally, utilizing DXA to assess lean (often used as a proxy for muscle) and fat tissue, each has its own limitations when considering mass screening and remains dependent on its availability, costs, and radiation exposure. Therefore, using some alternative technologies to measure body composition have been explored, including the bioelectrical impedance analysis (BIA). Most of the BIA devices assess total lean mass (muscle, organs, and body water) as a proxy for muscle. This limitation is offset in some devices by measuring only the appendicular tissue, mostly comprising muscle . However, the presence of ectopic fat complicates this approach. Additionally, most of these devices do not detect bone, thus cannot be utilized for OSA identification.
New technologies
Bone and muscle
Recently, a new device, BIA-ACC (BioTekna, Marcon-Venice, Italy), based on multi-frequency bioelectrical impedance and able to assess quantitative and qualitative body composition parameters, has been introduced. It was validated against DXA and was used to detect OSO in young men and women . This instrument detects total bone mass in the body (kg), along with minerals, calcium, magnesium, and phosphorus, but cannot distinguish among different skeletal sites or types of bone. The bone status is determined based on a normal reference population (which may be instrument-specific); therefore, a T-score of ≤−1 SD would indicate osteopenia/osteoporosis. This technology does not assess the ectopic fat in bone or changes in bone marrow fat; in other words, it does not address the quality of bone. However, BIA-ACC provides the information on skeletal muscle mass (kg and % fat-free mass) and S-score for muscle (analog to T-scores for bone), as well as intramuscular adipose tissue (IMAT). It also yields the amount of fat mass (kg and % body weight), total body water, and extracellular and intracellular water. The BIA-ACC may be complemented by another device, the PPG-StressFlow (BioTekna, Marcon-Venice, Italy), which assesses the biofeedback of the autonomic nervous system including heart rate, and provides information on chronic stress, systemic inflammation, oxidative stress, and insulin resistance . Therefore, in addition to their portability and ease of use, the synergy of BIA-ACC and PPG-StressFlow provides body composition assessment enabling the diagnosis of OSA, and at the same time shows underlying metabolic derangements such as low-grade chronic inflammation and stress, offering a more comprehensive assessment about overall health.
The unique material properties of bone regarding its strength and quality could not be measured in vivo (other than with some invasive technologies, like computerized tomography or expensive MRI) until recently. However, the introduction of impact micro-indentation (IMI) technology lent the evaluation of in vivo tissue-level properties of bone in humans. The metaanalysis evaluating studies using this technology, focusing on the OsteoProbe (ActiveLife Scientific Inc., Santa Barbara, California, United States), has been recently published . Briefly, the IMI measures the reference point micro-indentation and bone’s ability to resist the separation of mineralized collagen fibrils after the probe insertion. The final output is a calculated bone material strength index (BMSi), a ratio of the indentation distance in bone versus a reference material; the compromised bone strength allows the deeper probe indentation resulting in the lower BMSi. However, the OsteoProbe technology is invasive as it requires the application of a local anesthetic at a mid-shaft tibia where the probe is inserted through the skin. Nevertheless, this is a promising technology for the in vivo bone material properties and bone quality and fragility evaluation, especially when complemented with other technologies (e.g., DXA) .
Regarding the muscle quality assessment, the easiest way would be to calculate the phase angle from the BIA data by finding the arc-tangent of the reactance/resistance ratio . Although this does not directly measure the infiltrated fat in muscle, it could serve as a proxy for muscle tissue quality. Similarly, some researchers are using the knee extension measurements to assess muscle quality, as well as the lower body strength, where one repetition maximum in kg from the knee extension is divided by the leg lean mass in kg (most measured by DXA or BIA) . The BIA-ACC conveniently provides the analysis of phase angle, in addition to other muscle and body composition properties mentioned above (e.g., IMAT). Recent attention has been given to the advanced assessment of muscle tissue via ultrasound technology, particularly regarding its ability to distinguish different properties of the muscle. For example, the high-frequency ultrasound can evaluate the quality of skeletal muscle (e.g., quadriceps) by finding the echo intensity and using grayscale analysis with software such as Adobe Photoshop that can identify myosteatosis (infiltrated fat), otherwise negatively correlated with muscle strength and thickness, as well as age.
Adipose tissue
In order to offset the lack of consensus for overall obesity cut-offs, as well as a technological limitation for detection of ectopic/infiltrated fat, we recently proposed some alternatives for body fat assessment to help in OSA diagnosis . For example, instead of using the total percent of body fat, fat mass index (FMI) can be used, with the proposed cut-offs for men and women of ≥ 9 and ≥ 13 kg/m 2 , respectively . Regarding visceral fat, several criteria could be used including the ratio of visceral to subcutaneous fat or ratio of android to gynoid fat (when DXA measurements are available). In other words, several options are available, however, there is a need to establish the scientific group on osteosarcopenic adiposity to guide researchers in its diagnosis and identification, as is currently established for sarcopenia .
Serum biomarkers for bone, muscle, fat, inflammation, and stress—Developing the omics for OSA
Research within OSA identification is needed in the development of serum biomarkers, to eventually develop the omics for body composition and complement them with physical and functional measurements. The markers of bone turnover are well developed and have been used extensively in clinical and research settings with various successes for their predictability of bone status or fractures. The biomarkers for muscle breakdown/turnover are being investigated with heightened interest. We recently reported that sclerostin and skeletal muscle-specific troponin (sTnT), the markers of bone and muscle breakdown, respectively, were more sensitive in identifying OSA in overweight/obese older women than some other serum indicators . Serum sTnT has been previously associated with the onset of sarcopenia in older adults, and it decreased during their strength training, particularly in those who showed improvement in handgrip strength . The search for biomarkers may be accelerated by using and developing both female and male animal models, as it has been introduced for the former .
The circulating levels of inflammatory cytokines such as TNF-α, IL-1, and IL-6 and C-reactive protein increase with increased adiposity, particularly visceral , and cause low-grade chronic inflammation perpetuating obesity and damaging both muscle and bone . Additionally, it is of crucial importance to account for chronic stress that could affect a multitude of organs, including body composition via immune system modulation, all contributing to MSC lineage shift toward adipogenesis. In this context, the Western-type proinflammatory diet, altered lifestyle with reduced physical activity, and misaligned circadian rhythm, all create a vicious cycle contributing to compromised body composition and health .
The bottom line
While all mentioned instruments and technologies could be used in research and special circumstances, the mainstay technologies (although not available for a mass use or routine measurements) include MRI or EchoMRI and computed tomography (CT) that give the most comprehensive picture about any of the tissues discussed. The development of new technologies and easier use of the existing ones will enable a more universal identification of OSA and any of its components. Overall, while further studies are needed to determine the exact cut-offs and validation for various instruments, it is important to keep assessing body composition components with whatever tools are available and contribute them to the OSA diagnostic criteria. With such an approach, it will be possible to eventually assess all other ethnic and age groups of the population, as well as the individuals of various body composition categories and health conditions, and develop diagnostic cut-offs for each, and ultimately, gain better insights into the OSA syndrome.
What has the research on OSA/OSO showed so far?
Since its identification in 2014, the published research on OSA/OSO has grown rapidly, either based on retrospective and epidemiological studies, prospective analyses, or clinical interventions in different age and ethnic groups and populations across the world. Even more important is the fact that numerous published studies have accumulated data from which OSA/OSO could be retrospectively identified and related to various conditions, although at the time the condition was not reported as such. The problems for determining the overall prevalence of OSA/OSO in men and women still exist, due to the different ethnic groups and populations in which it has been studied and to the lack of uniformly accepted diagnostic criteria (as discussed above). In addition to the recently published review , the newest brief appraisal is captured in the following few paragraphs.
Several published studies originated from the Korean National Health and Nutrition Examination Surveys (mostly data from 2008 to 2009 and/or 2010 to 2011) and showed that individuals with OSO (≥ 50 years) had lower serum vitamin D levels , poorer diet quality , higher dietary inflammatory index and consumed a diet lower in fruit compared to their counterparts without OSO, but with some other of its components. Similarly, another cross-sectional study in individuals with OSO, showed that they had a less favorable body composition, fat distribution, and vitamin D levels, compared with only obese individuals in both sexes . Based on fracture site, OSO prevalence was more common in men with vertebral, hip, and ankle fracture while among women, only those with ankle fracture had higher OSO prevalence . However, another recent study showed no stronger correlation with insulin resistance in individuals with OSO compared to those with any other co-existing components or obesity alone , indicating greater fat mass is the strongest contributor to insulin resistance.
Researchers from other Asian countries reported various findings as well. For example, Chinese older women with OSO had significantly poorer blood lipid profiles compared to their counterparts without OSO . Another Chinese study conducted in four minority populations reported that those with OSO had a higher prevalence of hypertension . A Japanese study investigating the body composition impairments in patients with cardiovascular disease (CVD) and comparing them with community-dwelling controls, showed that the prevalence of sarcopenia, osteosarcopenia, and osteosarcopenic obesity was higher in the CVD patients than in the controls (16.9% vs 4.4%, P < .001; 8.8% vs 2.6%, P = .009; and 4.4% vs 0.9%, P = .036, respectively) indicating that CVD may aggravate body composition, or vice versa, creating a vicious cycle. Another Japanese study (only the abstract is in English) reported that the prevalence of sarcopenic obesity was 16% among older Japanese women (mean age 72.5 years), however, over half of them also had osteopenia, identifying them as actually having OSO .
Our previous study in Caucasian, overweight/obese postmenopausal women showed that those with OSO had lower handgrip strength, poorer balance, and inferior walking abilities compared to women with obesity-only . This lower functionality may emphasize even more the intrinsic complex nature of the OSO syndrome and the increased risk for falls. For example, in the study with 543 Mexican middle-aged and older males and females, OSO (16.6%of study population) was independently associated with frailty (as measured by the Frailty Phenotype and FRAIL scale) and a lower Short Physical Performance battery score .
There were also a few interventional studies with resistance training examining its effect on various OSO markers. A Brazilian study in older women with OSO showed that a 12-week resistance training improved some of their functionalities, even in a dose-response, where three sets induced more benefits than one set . Another study in older Brazilian women who were followed for 24 months with regard to their functionality and habitual physical activity, showed that regardless of whether they had OSO or not, the physical activity was inversely related to their functionality. However, those who had OSO were less active and presented with higher functional disabilities . The intervention with elastic band resistance training for 12 weeks (three times/week) was conducted as a randomized controlled trial in Iranian older women with OSO. The results showed that women who received training improved in some of the functionality measures (e.g., handgrip strength, 30-s chair test), but not in others (e.g., gait speed) or any of the body composition parameters (BMI, BMD, or body fat). Likewise, there was no improvement in the serum sclerostin and skeletal troponin , the markers shown previously as sensitive indicators for bone and muscle loss, respectively .
In the Australian Busselton Healthy Aging Study, higher body fat was associated with a lower bone mineral density in females but not in males; however, the highest body fat groups also had the lowest lean mass index (kg/m 2 ) in both males and females , indicating the presence of OSO. The Louisiana Osteoporosis Study, a large cross-sectional study of ≥ 18 years individuals, showed the prevalence of sarcopenic obesity of 7% in males and 2.5% in females, with the older age groups contributing the most . This is another important study as it also collected bone density measurements, making it an ideal database to estimate the prevalence of OSO in that wide age-range population. However, the most striking report was the presence of OSO in apparently healthy young overweight/obese Greek males and females compared to their normal-weight counterparts as the OSO phenotype was not anticipated in young, healthy individuals. Another striking result is a recent finding of high prevalence of OSA in Croatian nursing home residents (Keser et al., abstract in press). In that study, n = 84 nursing home residents (82.1% females, 81.2 ± 6.8 years old with BMI of 29.2 ± 5.0 kg/m 2 ), were analyzed by bioimpedance devices, BIA-ACC and PPG-Stress Flow (BioTekna, Marcon-Venice, Italy). The prevalence of OSA was 66.7% in women and 40.0% in men, with a very low phase angle and a high percentage of extracellular water indicating poor health. These findings indicate the urgency of evaluating body composition and OSA in that population (Keser et al., abstract in press), particularly during COVID-19, as addressed below.
To our knowledge, there were no explicit longitudinal or other studies investigating various diseased states and how they might influence OSA/OSO or vice versa. However, there are some correlational studies, worth mentioning. For example, Carsote and colleagues reported that malignancy might lead to OSO and that malignancy of all causes in the presence of OSO would result in a worse prognosis . In another study investigating males with or without HIV, all three components of OSO, osteoporosis, sarcopenia, and obesity (visceral adipose tissue area), were associated with frailty, although BMI or subcutaneous fat were not associated with similar relationships . The authors emphasized that interventions for all three conditions (OSO) would help to treat and reduce the risk for frailty in this population. In a sample of 40 women (mean age of 40.7 years) with rheumatoid arthritis, 42.5% were diagnosed with OSO , suggesting rheumatoid arthritis may promote its development or vice versa. A case study in male identical twins 76 years of age suggested that the twin undergoing androgen deprivation therapy for prostate cancer was at higher risk for OSO and frailty due to the overt worsening in body composition, namely increase in visceral adiposity and muscle weakening . It is known that both estrogen and androgen deprivation therapies in breast and prostate cancers respectively, result in hypogonadism and altered bone and body composition. Therefore, it was recently suggested that when evaluating fracture risk in those patients, it might not be enough to just evaluate BMD and Fracture Risk Assessment Tool (FRAX) scores, but also overall body composition (often underestimated in cancer patients), indicating the need for comprehensive analysis for the presence of OSA syndrome . A recent study in a group of patients with Cushing’s disease identified significantly lower irisin levels compared to controls, and irisin’s independent relationship with osteosarcopenic obesity, suggesting irisin could serve as a possible marker for OSO in Cushing’s Disease .
While low-grade chronic inflammation has been linked earlier to the development of osteoporosis, obesity, and subsequently to OSA , recent reviews show the association of chronic stress, including dehydration, with OSA , as well as the immune system modulating stress and body composition . This insight gives rise to yet another component; chronic stress affects body composition via immune system and opens up the less explored area where nutritional and lifestyle measures could be implemented for the improvement of body composition, inflammation, and stress.
Overall, there is a growing body of evidence supporting the existence of OSA/OSO as a separate entity in populations of different ethnicities and backgrounds, not limited just to aging or to women (as initially identified) and indicating that its consequences are larger than the sum of each condition alone, as discussed below.
OSA health consequences
It is reasonable to hypothesize that due to fat infiltration, inflammation and metabolic and functional dysfunction in three tissues, the health consequences of OSA with comorbidities are greater than the sum of its individual components, despite some doubts . For example, when examining each of the individual conditions, osteopenia/osteoporosis with its diminished BMD leads to increased risk of fractures, which will be aggravated by impaired mobility and increased falls secondary to sarcopenia. The weakened muscle might weaken the bone even more, or vice versa. The distorted and redistributed adipose tissue with its infiltration in bone and muscle will weaken each, as well as cause low-grade chronic inflammation resulting in multiple ill consequences to all other organs and tissues, including susceptibility to and poor outcomes in COVID-19 infection, as discussed below. In other words, although each individual condition has its associated comorbidities, health outcomes are compounded when all three are present (osteopenia/osteoporosis + sarcopenia + adiposity) and their outcomes are worse than just a sum of three. Crosstalk occurs between all three tissues, therefore in the OSA syndrome, each tissue may worsen another, multiplying the overall ill effects and resulting in heightened vulnerability to chronic poor health, risk of falls and bone fractures, disability, and frailty. Such conditions lead to low quality of life, nursing home placement, and morbidity, as well as premature death—definitely more than just the sum of each of OSA components alone.
Since a progressive loss of bone and muscle and increase in adipose tissue is inevitable with normal aging (and even more so in some chronic conditions), identification of the epidemiological overlap of osteoporosis, sarcopenia, and increased adiposity is crucial, especially given the common risk factors and cellular origins. Evidence for the pathophysiological overlap of these conditions is expanding, particularly with more understanding about the interplay of inflammatory and hormonal mechanisms. However, a causal relationship among osteosarcopenic adiposity components is not clearly identified, although some origins were pointed out in the text above. Obviously, more comprehensive research to understand the underlying biological phenomena linking the three conditions are needed. In other words, the OSA model is ideal for studying, monitoring, and possibly managing and treating changes after any internal or external insults (including age) affect body composition, especially considering that those tissues originate from the same stem cell lineage.
OSA in the time of COVID-19
The COVID-19 pandemic has brought much-deserved attention to the importance of the immune system and the fact that underlying conditions worsen the outcomes from COVID-19, especially in obesity . The OSA syndrome emphasizes the interconnected nature of the human body and the postulate that having one condition may result in the development of others with the resulting ill condition being greater than the sum of its parts. The evidence for crosstalk between bone, muscle, and adipose tissues and the connection between the immune system and inflammation , the common traits in OSA, are well known. The cytokine storm described in COVID-19 may be rooted in the low-grade chronic inflammation present before contracting COVID-19.
All tissues may have resident immune cells and the immune system involvement in bone resorption/formation is known as osteoimmunology . Skeletal muscle is no different; for example, there is a distinct type of regulatory T-cells present in the muscle of mice . Some of the myokines released from working muscles may be part of the crosstalk with the immune system and the absence of these (e.g., in sedentary muscle), may result in some immune dysfunction in muscle tissue . Muscle goes through a regulated cycle of events when undergoing repair; injury—inflammation—repair initiated—resolved, and a disruption of this can result in fat infiltration . Other acute or chronic muscle diseases as well as aging may disrupt the immune-cell-muscle balance . Compared to the role of the immune system in bone and muscle metabolism, the immune system’s role in excess adiposity is better known. Low-grade inflammation due to the increasing number and size of adipocytes is at the heart of the systemic metabolic disorders associated with obesity . In fact, it is possible that crosstalk between the immune system and adipose tissue regulates adipose tissue homeostasis .
For OSA specifically, no direct data on its relationship with COVID-19 are available (both conditions are new and the latter one has taken us by storm). That obesity increases the risk for contracting COVID-19 and worsen the outcomes has been shown recently . Regarding bone health and COVID-19, a small study ( n = 10) from China showed that patients with fractures and limited mobility had a higher mortality rate compared to those without fractures . Data from the New York City hospital showed an increased need for oxygen in patients with hip fracture after surgery, but those with asymptomatic or mild COVID-19 infection had only a 10% mortality rate (compared to more than 40% in other countries) . Regarding sarcopenia and COVID-19, no data are available to distinguish the outcomes for those with or without sarcopenia. However, COVID-19 probably results in muscle loss in patients who are symptomatic, even just considering the reduced food intake and lack of activity, with even more detrimental muscle loss in those who are severely affected or who drastically change their lifestyle during social isolation (sedentary, poor diet, depression, etc.) .
Our recommendations within the auspices of personalized nutrition/medicine
We have already published comprehensive nutritional and physical activity recommendations for OSA and we recently provided a preliminary algorithm for its treatment and management . Briefly, after a global medical, nutritional, and functional assessment has been performed, the strategies to maintain or treat OSA would depend on each individual’s status and may be considered within the auspices of personalized nutrition/medicine. Additionally, the steps to reduce low-grade chronic inflammation, chronic stress, and the importance of maintaining a healthy microbiome with nutrition, exercise, or even pharmacological agents, should be considered . No less important is the robust circadian rhythm for the proper functioning and synchronization of all body processes. Unfortunately, modern humans’ lifestyle does not always translate to the optimal chrono-biology leading to increased risks for metabolic disturbances and diseases of modern time, including altered body composition .
Below are general suggestions that could be individually adapted and fine-tuned for each patient. The target for energy intake would depend on the need for weight loss or maintenance, but in general should be 25–30 kcal/kg/day, with about 1–1.2 g/kg/day of good quality protein (possibly ~ 25% of overall energy intake). The remaining energy intake should be balanced between carbohydrate and fat, to include: ~ 40% from nonprocessed carbohydrates with very limited simple sugars and ~ 35% from fat with < 10% saturated fatty acids and no or very limited trans fatty acids. A focus should be to increase omega-3 polyunsaturated fatty acids, including more than 1 g/day (combination of eicosapentaenoic acid [EPA], docosahexaenoic acid [DHA], and the essential, alpha-linolenic acid). Fiber in the amount of 25–30 g/day is recommended, but it is usually harder to achieve. Some pre or pro-biotic foods or supplements may be recommended instead.
The micronutrients should include balanced minerals and vitamins intake, mostly at or above the Dietary Reference Intakes (DRI). That includes calcium, magnesium, and potassium (with about 1200 mg/day, 320 mg/day, and 4500 mg/day, respectively), but also adequate intake of zinc, selenium, manganese, and copper (deficiencies implicated in depression). All of them also improve the antioxidative capacity of mitochondria in repairing DNA damage and reducing inflammation and stress. On the other hand, among other minerals, sodium and phosphorus (except in severely sick patients) should be reduced and kept in check, along with too much iron .
Among vitamins, vitamin D has been studied most extensively and as of now, the intake of about 800 IU/day is recommended to keep the serum levels ≥ 20 ng/mL in older or otherwise compromised individuals. Besides its undisputed involvement in bone metabolism/calcium absorption, it also has a role in preventing falls and increasing muscle performance and its deficiency is associated with sarcopenia. Other antioxidant vitamins, like vitamin A, C, E, and carotenoids, along with vitamins from the B group are all involved in multiple metabolic processes regulating body composition, inflammation, and related chronic stress. In this respect, B 1 (thiamine), B 3 (niacin), and B 6 (pyridoxine) have been implicated in impaired neurotransmitter synthesis, while B 9 (folic acid) and B 12 (cyanocobalamin), both involved in the recycling of homocysteine (toxic to mitochondria and cardiovascular system) into methionine, have been implicated not just in somebody composition regulation but also in stress-related mitochondrial damage and impaired neurotransmitter signaling .
Regarding the exercise program, we also suggest that activities be developed on an individual basis and centered on preference and abilities. While following the official exercise regimens (e.g., for weight loss, muscle strength or balance improvement, or overall well-being) could serve as some general guideline, it depends on each individual’s condition, abilities, and state of the mind. Therefore, in the personalized-physical activity approach, the exercise or the amount and kind of physical activity should be addressed individually and recommended depending on the overall assessment. However, based on the evidence we have so far on OSA, we do recommend a comprehensive exercise program, depending on needs, but mostly on preferences, as the latter ensures adherence and regularity. The program could include all or some of the following varieties and options: aerobic, resistance, endurance, weight-bearing, strength, flexibility, and balance in the duration and frequency found most achievable by each individual. The official recommendations are 60 min of some exercise 5 days/week. However, performing each kind or amount according to the abilities and preferences makes each one easier to achieve and eventually leads to the overall goals; benefits to weight, body fat, bone, and muscle, improvements in balance, flexibility, cognition, and decrease in proinflammatory cytokines. Additionally, exercises like yoga, pilates, and tai chi have shown similar benefits but perhaps to a milder degree, especially considering body composition outcomes . However, more studies in these exercise forms will provide evidence regarding their benefits for the prevention and treatment of OSA.
Conclusions
The evidence presented highlights the interconnectedness between bone, muscle, and adipose tissues and the central role of the immune system in their homeostasis. OSA is a multifactorial syndrome with its adverse health consequences larger than the sum of its parts (osteoporosis, sarcopenia, and obesity) alone. Crosstalk occurs between all three tissues, therefore within the OSA syndrome, each tissue may worsen another, multiplying the overall ill effects and resulting in heightened vulnerability to chronic poor health, risk of falls and bone fractures, disability and frailty, leading to low quality of life, nursing home placement, and morbidity, as well as premature death. All these effects are heightened and magnified in the COVID-19 pandemic. Optimizing the nutritional and lifestyle habits may contribute to better bone, muscle, and adipose tissues health as well as prevent/treat underlying conditions, including inflammation, and at the same time increase resilience.
In view that a progressive loss of bone and muscle and increase in adipose tissue is inevitable with aging and even more so in the presence of other comorbidities, identification of the epidemiological overlap of osteoporosis, sarcopenia, and adiposity is crucial. However, a causal relationship among osteosarcopenic adiposity components is not clearly identified although some common risk factors and cellular origins are outlined in the text. More comprehensive research to understand the underlying biological phenomena linking the three conditions and the inescapable impact of the immune system is needed.
References
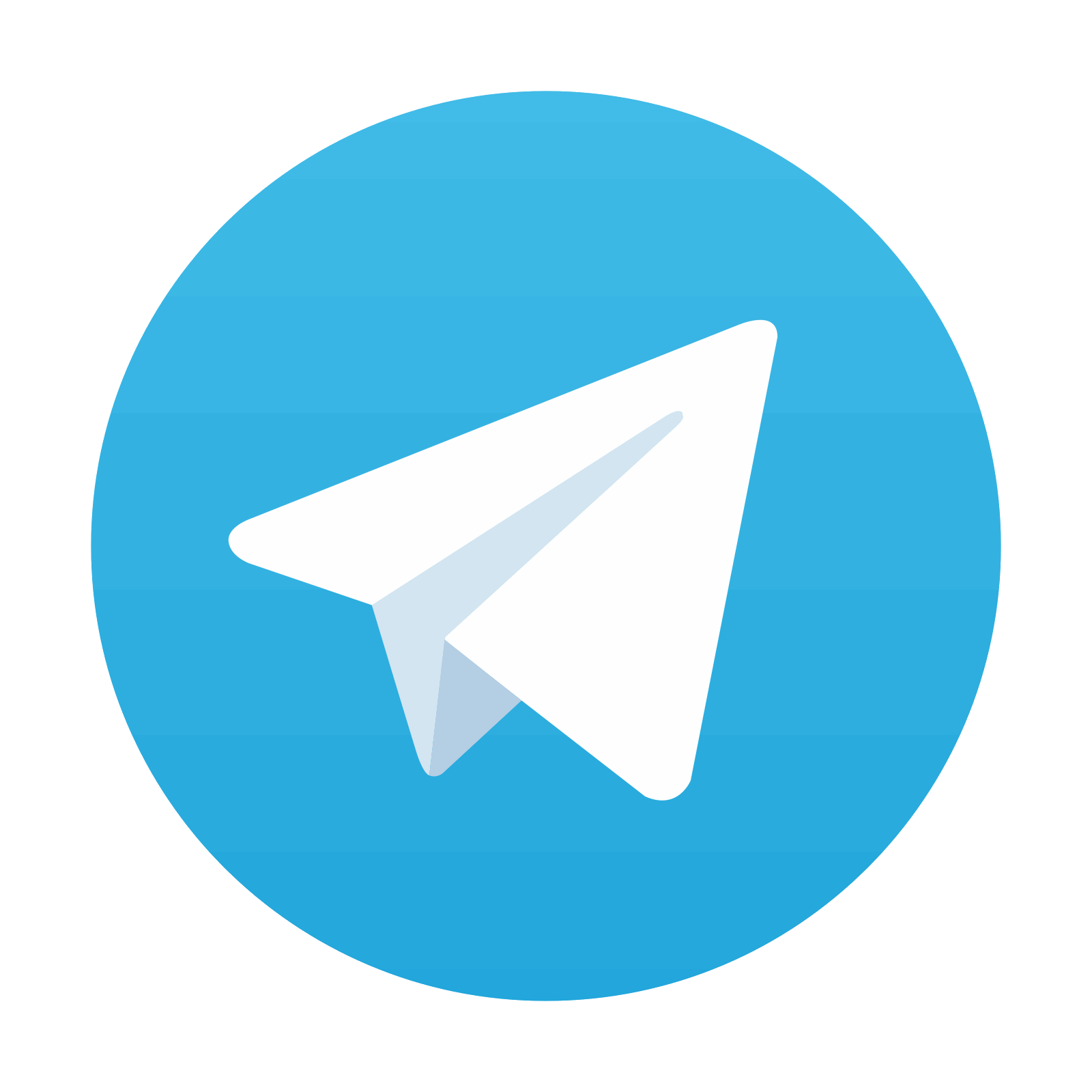
Stay updated, free articles. Join our Telegram channel

Full access? Get Clinical Tree
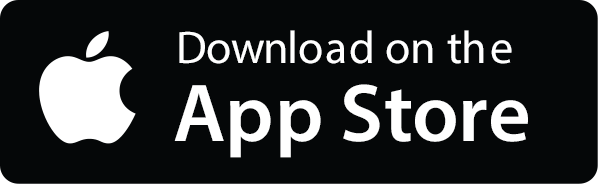
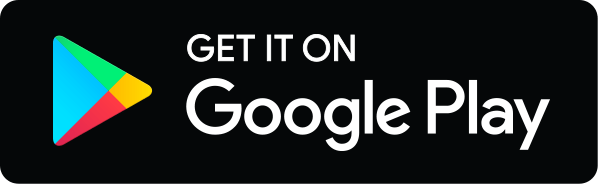
