Opioid Pharmacotherapy
Judith A. Paice
Opioids and their derivatives have been used to relieve pain for centuries, and their contribution to relief of suffering is well established. Many of the earliest recorded reports of opioid use are attributed to the Egyptians and extend back many centuries. In the 1600s, Thomas Sydenham promoted the use of laudanum, a mixture of opium, saffron, cinnamon, and cloves in wine (1). Two centuries later, the pharmacist Wilhelm Sertürner extracted morphine from poppy juice, calling this substance morphium after Morpheus, the Greek god of sleep (1). Since that time, many new opioids have been developed, and information about the pharmacodynamics, pharmacokinetics, and pharmacogenomics of these compounds has increased greatly. This understanding has led to improvements in their clinical application, for relief not only of pain but also of dyspnea, cough, and intractable diarrhea. Yet, more than any other agent, opioids generate fear, misunderstanding, and controversy. To address these misconceptions and provide optimal relief, those caring for patients with pain and other symptoms associated with cancer or other life-threatening illnesses must have a strong knowledge base regarding the pharmacology and clinical application of opioids.
Pharmacology of Opioids
To fully appreciate the optimal clinical use of opioids, the clinician must understand the pharmacodynamics (the mechanism of opioid analgesia) and the pharmacokinetics (the process by which opioids are absorbed, distributed, metabolized, and excreted) of this class of drugs. The pharmacogenomics of opioids helps explain the variability in response seen in the clinical setting.
Pharmacodynamics
Opioids act through three major types of opioid receptors, including μ (MOR for μ opioid receptor), δ (DOR for δ opioid receptor), and κ (KOR for κ opioid receptor). These receptors are distributed widely throughout the nervous system, including the peripheral nerves, spinal cord, and brain (2). The highest density of opioid receptors include lamina I and II of the dorsal horn of the spinal cord (3). Opioid receptors are also found in the brainstem, including the periaqueductal gray, nucleus raphe magnus, and locus coeruleus, areas known to be involved in the mediation of opioid analgesia (4). More recently, opioid receptors have also been found on immune cells.
Opioid receptors are G protein–coupled, activating a complex cascade of events. These include increased conduction through potassium channels, which hyperpolarizes the sensory neuron. Opioid receptor binding results in diminished conduction through calcium channels, resulting in decreased release of neurotransmitters involved in nociception. Finally, opioid receptor binding leads to inhibition of adenylate cyclase. Together, these actions contribute to the analgesia resulting when an opioid agonist binds to the above receptors.
Pharmacokinetics
As with all other compounds, the absorption, distribution, metabolism, and elimination of an opioid influences the efficacy of the drug. Alterations are of particular concern when caring for patients with advanced malignancy or other life-threatening illness because any of these phases of the opioid may be altered by extensive disease.
Absorption
Absorption is influenced by the lipophilicity of an agent. Morphine and hydromorphone have a partition coefficient (octanol/water) of 1 compared with 115 for methadone and 820 for fentanyl (5). Therefore, fentanyl can cross biologic membranes more avidly when compared with morphine, making it the more appropriate agent for transdermal delivery. The lipophilicity affects the time to maximal serum concentration (Cmax). The Cmax for a hydrophilic drug, such as morphine, is approximately 60 minutes after oral administration, 30 minutes after subcutaneous (s.c.) delivery, and 6 minutes or more after intravenous (i.v.) delivery. The half-life of oral morphine is approximately 4 hours. Because steady state is reached in approximately four to five half-lives, it will be reached within approximately 16–20 hours of regular immediate-release oral morphine administration. Little is known about the alterations in absorption of opioids that occur when patients have extensive disease. For example, factors such as shortened transit time may delay the absorption of oral opioids, particularly long-acting or sustained-release compounds.
Distribution
Plasma proteins and lipid solubility of a particular opioid affect the distribution of the drug throughout the vasculature (5). Other mediating factors include body fat stores and total body water. All of these listed above can be significantly altered in
older adults or in persons with cachexia and dehydration, the common sequelae of advanced disease.
older adults or in persons with cachexia and dehydration, the common sequelae of advanced disease.
Metabolism
Most opioids are metabolized through glucuronidation, dealkylation, or other processes, and are then excreted by the kidneys. Although some metabolites produce analgesia (e.g., morphine-3-glucuronide (M3G), they may also contribute to neurotoxicity (6). Myoclonus has been associated with both M3G and hydromorphone-3-glucuronide (H3G), metabolites that appear to pose a risk for accumulating in patients receiving high doses of opioid for extended periods or in those with renal disease (7). Metabolism is known to be affected by advanced age, liver disease, genetics, and other factors that are prominent in palliative care.
Elimination
Most opioids are excreted renally, with a small percentage eliminated fecally. Experience suggests that patients with renal failure or those receiving dialysis might benefit from the use of agents that are more readily dialyzable, such as fentanyl, as opposed to morphine or codeine (8). However, even in patients who have undergone a successful renal transplantation, the large variability in the kinetics of fentanyl after surgery supports the axiom that all opioid therapy must be individualized (9). Much more research is needed on the interaction between advanced disease and the pharmacokinetics of opioids.
Pharmacogenomics
The field of pharmacogenomics is rapidly growing and much evidence for the variability in response to opioids seen in the clinical setting is related to inborn properties caused by genetic variability (10). The MOR was cloned in 1993 and was called MOR-1. More recent work has identified splice variants of the MOR-1 receptor, with different localization of the splice variants within the nervous system (11). The efficacy of morphine varies among the variants, and this may explain, in part, the variability in response to opioids seen in the clinical setting, including efficacy and adverse effects. The translation of this information to the clinical experience includes the practice of opioid rotation when a particular opioid agonist is either ineffective or produces unmanageable adverse effects.
Another clear clinical example of the contribution of pharmacogenomics to the variability in analgesia to opioids is related to codeine. People who are poor metabolizers of the enzyme CYP2D6 derive little analgesic effect from codeine, a prodrug that must be metabolized to morphine to produce analgesia. Approximately 5–10% of whites, 1% of Asians, and 0–20% of African Americans are poor metabolizers (12). Conversely, a recent case report described overdose in a patient taking a small dose of codeine. Analysis revealed him to be an ultrarapid metabolizer of CYP2D6, which theoretically resulted in a rapid, extensive conversion of the drug to morphine (13).
A recent example of the role of pharmacogenomics in cancer pain control is related to the catechol-O-methyltransferase (COMT) gene, which inactivates dopamine, epinephrine, and norepinephrine in the nervous system. A recent study of 207 white patients with cancer found that polymorphism of the COMT gene contributes to variability in response to morphine in pain control (14). A common functional polymorphism (Val158Met) leads to a significant variation in the COMT enzyme activity, with the Met form displaying lower enzymatic activity. Patients with the Val/Val genotype needed more morphine, when compared with the Val/Met and the Met/Met genotype groups. The investigators could not explain these differences by other factors such as duration of opioid treatment, performance status, time since diagnosis, perceived pain intensity, adverse symptoms, or time until death. Much more research is needed to fully understand the pharmacogenetics of opioids and their implications for those with cancer or other life-threatening illnesses.
Clinical Application
Opioids are a critical component of the armamentarium used to control pain in palliative care. They are indicated in moderate to severe pain, as well as in the management of cough, dyspnea, and severe diarrhea. Because opioids alter pain signal transmission and perception throughout the nervous system, they have an analgesic effect despite the underlying pathophysiology of pain. In fact, despite earlier beliefs, opioids have been shown to be effective in providing relief of neuropathic pain.
Specific Opioids
Opioids are generally categorized as agonists, partial agonists, and mixed agonist–antagonists. Additionally, antagonists to opioids may be used to counteract adverse effects to opioids, although most must be used with significant caution in the palliative care setting.
Agonists
Numerous opioid agonists are available for clinical use. These agents can be subcategorized as alkaloids and synthetic opioids (Table 3.1). Attributes associated with the more commonly used opioids are described in the following text and in Table 3.2.
Table 3.1 Opioids By Classification | |||||||||
---|---|---|---|---|---|---|---|---|---|
|
Table 3.2 Properties of Commonly Used Opioids | ||||||||||||||||||||||||||||||||||||||||||||||||||||||||||||||||||||||||||||
---|---|---|---|---|---|---|---|---|---|---|---|---|---|---|---|---|---|---|---|---|---|---|---|---|---|---|---|---|---|---|---|---|---|---|---|---|---|---|---|---|---|---|---|---|---|---|---|---|---|---|---|---|---|---|---|---|---|---|---|---|---|---|---|---|---|---|---|---|---|---|---|---|---|---|---|---|
|
Codeine
Codeine is a relatively weak opioid that is more frequently administered in combination with acetaminophen. Codeine is metabolized by glucuronidation primarily to codeine-6-glucuronide, and to a much lesser degree to norcodeine, morphine, M3G, morphine-6-glucuronide, and normorphine (15). As described earlier in the section Pharmacogenomics, codeine is converted to morphine by CYP2D6. The polymorphism seen in this enzyme between various ethnic groups, and between individuals, leads to a significant percentage obtaining reduced analgesia.
Fentanyl
Fentanyl is a highly lipid-soluble opioid (partition coefficient 820) that has been administered parenterally, spinally, transdermally (including an iontophoretically administered patient-controlled device), transmucosally, intranasally, and by a nebulizer for the management of dyspnea (16, 17). Dosing units are usually in micrograms because of the potency of this opioid. Questions arise about the efficacy of fentanyl and related compounds, alfentanil, remifentanil, and sufentanil, in the face of extremes in body weight. A recent study of i.v. fentanyl for acute postoperative pain in lean and obese patients found no relationship between plasma levels required for analgesia and total body weight. Therefore, using i.v. dosing on the basis of weight (or milligram per kilogram dosing) in patients with cachexia could lead to underdosing, whereas the same practice in the obese patient could lead to overdose (18). Similar studies are needed to determine the effect of weight on the plasma levels of fentanyl after transdermal delivery, particularly in the often cachectic palliative care patient.
Hydrocodone
Hydrocodone is more potent than codeine and is found only in combination products, primarily acetaminophen, but also ibuprofen. Liquid cough formulations of hydrocodone also contain homatropine. These additives limit the use of hydrocodone in palliative care when higher doses of opioid are required. Hydrocodone is metabolized through demethylation to hydromorphone. Laboratory evidence suggests that CYP2D6 polymorphism may alter the analgesic response to hydrocodone (19).
Hydromorphone
Hydromorphone is a derivative of morphine, with similar properties, and is available as oral tablets, liquids, suppositories, and parenteral formulations (20). Because it is highly soluble and approximately five to ten times more potent than morphine, hydromorphone is used frequently in palliative care when small volumes are needed for s.c. infusions. A long-acting formulation is available outside the United States. Hydromorphone undergoes glucuronidation and the primary metabolite is H3G (15). Recent experience suggests that this metabolite may lead to opioid neurotoxicity, similar to those seen with morphine metabolites, including myoclonus, hyperalgesia, and seizures (7, 21, 22). This appears to be of particular risk with high doses, prolonged use, or in persons with renal dysfunction (23).
Methadone
Methadone has been gaining renewed popularity in the management of severe, persistent pain. Methadone is a μ and Δ agonist and is an antagonist to the N-methyl-D-aspartate (NMDA) receptor, with affinity similar to that of ketamine (24). This antagonism is believed to be of particular benefit in neuropathic pain. A double-blind study in palliative care patients revealed its efficacy in the management of neuropathic pain, although a Cochrane review of existing studies found no obvious clinical effects (25, 26). Methadone also blocks the reuptake of serotonin and norepinephrine, another potentially favorable attribute to its use in treating neuropathic pain. The prolonged plasma half-life of methadone (ranging from 15 to 60+ hours) allows for a relatively convenient dosing schedule of every 8 hours. Furthermore, methadone is much less expensive than comparable doses of commercially available continuous-release formulations.
Another advantage of the use of methadone in palliative care is the variety of available routes that can be used, including oral, rectal, s.c., i.v., and epidural (27). Nasal and sublingual administration has been reported to be effective, but preparations are not currently available commercially. The ratio of oral to parenteral methadone is 2:1 and of oral to rectal is 1:1. Subcutaneous methadone infusions may produce local irritation, although using a more dilute solution or changing the needle more frequently can mediate this.
Despite the many advantages, much is unknown about the efficacy of methadone when compared with other agents, the appropriate dosing ratio between methadone and morphine, or the safest and most effective time course for conversion from another opioid to methadone. Despite much anecdotal support for the use of methadone, Bruera et al. conducted a randomized controlled trial in patients with cancer and found no significant difference when compared with morphine (28). Early reports suggested the analgesic ratio of morphine to methadone might be 1:1, yet this appears to be true only for individuals without recent prior exposure to opioids (29). For individuals currently taking another opioid, the dose ratio increases as the dose of oral opioid equivalents increases (30, 31). The oral morphine to oral methadone ratio may be 2:1 for patients on <30 mg of oral morphine equivalent daily doses (MEDD) but may be 10:1 or 20:1 for patients on >300 mg oral MEDD (Tables 3.3 and 3.4). An additional complicating factor in the use of methadone is the limited experience in reverse rotation from methadone to another opioid (29, 30).
The kinetics of methadone vary greatly between individuals, and causes for the variability include protein binding, CYP3A4 activity, urinary pH, and other factors. Methadone binds avidly to α1 glycoprotein (AAG), the level of which is increased in advanced cancer, leading to decreasing amounts of unbound methadone and initially delaying the onset of effect. As a result the interindividual variability of the pharmacokinetics of methadone is more pronounced in patients with cancer (24).
Methadone is metabolized primarily by CYP3A4, but also by CYP2D6 and CYP1A2 (24, 32, 33). Drugs that induce CYP3A4 enzymes accelerate the metabolism of methadone, resulting in reduced serum levels of the drug. Patients report shortened analgesic periods or reduced overall pain relief (34). Drugs that inhibit CYP3A4 enzymes slow methadone metabolism, potentially leading to sedation and respiratory depression. Table 3.5 lists many agents commonly used in palliative care that are CYP3A4 inducers and inhibitors.
Table 3.3 Methadone Dose Ratios | ||||||||||||||||
---|---|---|---|---|---|---|---|---|---|---|---|---|---|---|---|---|
|
Table 3.4 Protocols for the Conversion from Other Opioids to Methadone | |||||||||
---|---|---|---|---|---|---|---|---|---|
|
Urinary pH can account for a significant amount of the variability seen in methadone plasma levels. Clearance of methadone is greater when the pH is more acidic (<6). As a result, urinary alkalizers, such as sodium bicarbonate, will decrease methadone excretion. Other factors that alter methadone kinetics include drug interactions. In addition to the CYP3A4 interactions listed previously, others have been described. For example, the proton pump inhibitor omeprazole increases gastric pH, increasing the rate of absorption.
Although the extended half-life of methadone allows longer dosing intervals, it also increases the potential of drug accumulation, leading to delayed sedation and respiratory depression. This may occur 2–5 days after initiating the drug or increasing the dose. Another consequence of prolonged or high-dose opioid administration, myoclonus, has been reported with methadone use (35). Finally, controversy exists about the role of methadone in QT-wave interval prolongation (Torsade de pointes). Some question whether this is due to preservatives in the parenteral formulation, although the syndrome has been reported with oral administration of methadone (36). A more recent study of 100 patients taking methadone found that one third had prolonged QT-wave intervals on electrocardiogram, occurring more frequently in men, yet there did not appear to be a risk of serious prolongation (37, 38).
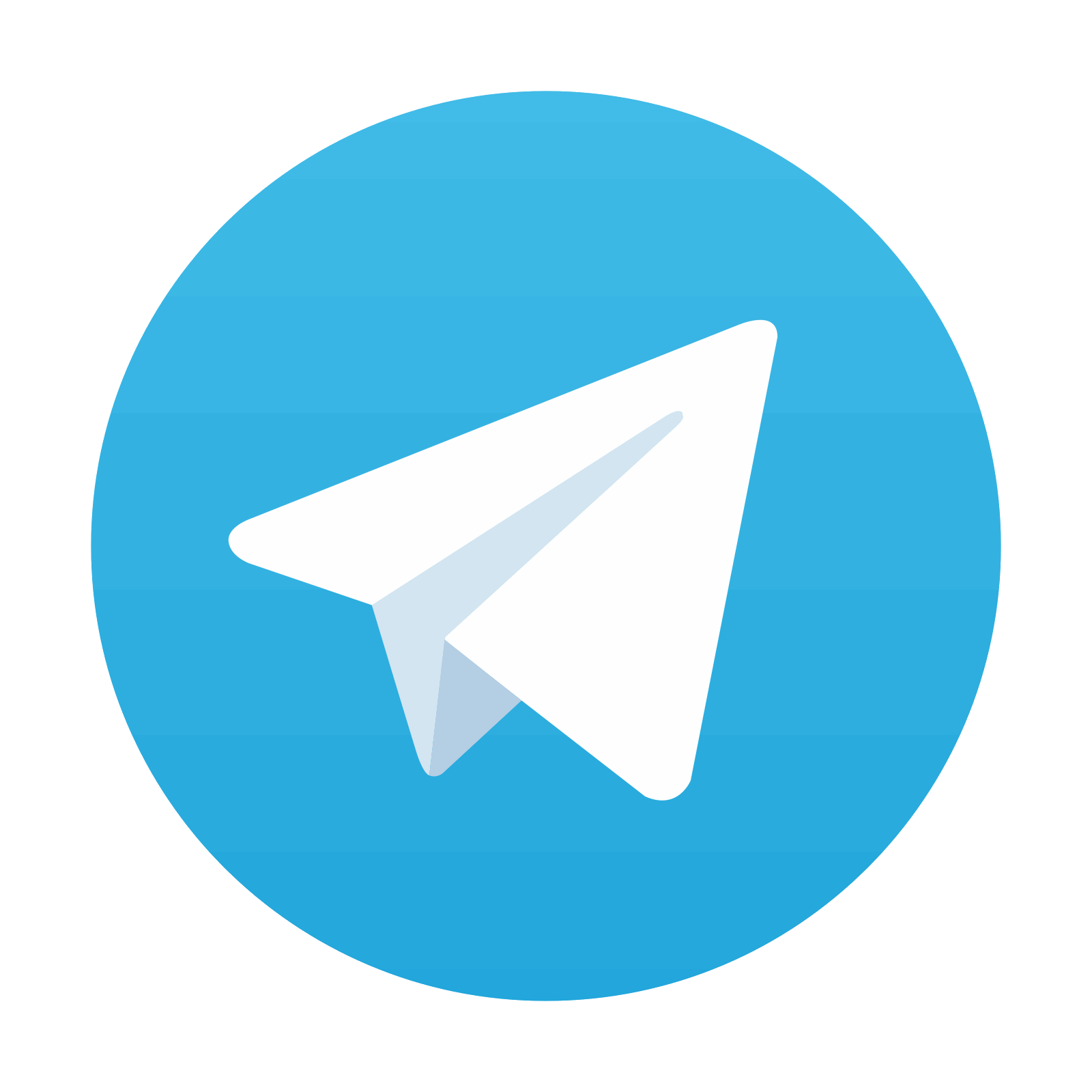
Stay updated, free articles. Join our Telegram channel

Full access? Get Clinical Tree
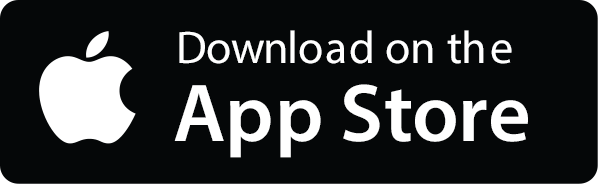
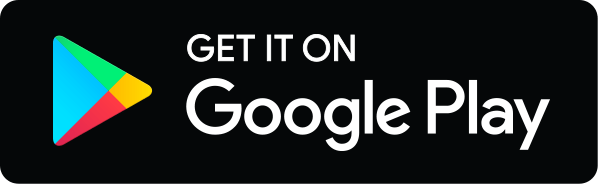
