Fig. 1.1
Relationship between gene, DNA, RNA, and protein (the central dogma of molecular biology) with omics technologies
Genomics
Genomics may be described as the comprehensive analysis of genes and their DNA structure and function or as the scientific discipline looking for information about the entire genome. Before the existence of omics technologies, DNA was sequenced gene by gene, but improving informatics technology and integrating them to the biology have allowed the sequencing of the entire genomes of many types of organisms and the classification and storage of DNA sequences in genomic databases. Variations of the genes or DNA sequences can be created through gene amplification, gene deletion, or gene rearrangement [8].
Copy number aberrations (CNA) or copy number variations (CNV) are the names that encompass the alterations of the organization and amount of DNA within a cell. Genetic alterations are generated by deletions, duplications, inversions, or translocations of chromosomes; moreover these modifications are capable of being inherited. CNA or CNV can disturb the normal transcriptional activity of a cell, increasing or decreasing it, changing the normal expression of genes [9].
Gene amplification is one of the mechanisms in which a gene can be overexpressed and cause the activation of oncogenes; therefore it is related to disease progression and poor prognosis. It is created by an increase in the copy number of a loci or restricted area of a chromosome arm. Amplification occurs recurrently on some chromosomal locations, indicating the common activation of some oncogenes during tumor development [10]. The HER2 is the most studied breast cancer oncogene; it is located on chromosome 17q21.1 and encodes a transmembrane protein that is similar to the epidermal growth factor the HER1. A normal cell presents two copies of the HER2 gene and about 50,000 copies of the protein, whereas by gene amplification in a breast cancer cell, there can be more than one copy of the HER2 gene and more than one million copies of the protein [11].
High-throughput technologies are needed to discover, monitor, and quantify these DNA modifications. The most widely used technique involves the microarrays that simultaneously monitor the expression levels of thousands of genes inside different samples. This technique has advanced rapidly in recent years. It involves a surface fixed with either a tissue or a panel of different DNA or RNA molecules, proteins, or antibodies that have the possibility of linking up with a corresponding DNA/RNA/protein/antibody from a sample. Genomic microarrays, also called array comparative genomic hybridization (array-CGH), are used to study and quantify chromosomal abnormalities, microdeletions and microduplications, and copy number aberrations (CAN), at a wide level in an entire genome. Array-CGH has a refined process and resolution, which enables evaluation of the genome of any cell, while chromosomal-CGH allows the identification of gene regions where there is DNA gain or DNA loss [1, 12].
Chromosomal-CGH studies have identified that the loss of 16q is one of the most consistent DNA aberrations found in infiltrating lobular carcinoma (ILC). Etzell et al. studied genomic alterations in lobular carcinoma in situ (LCIS). Using comparative genomic hybridization, they found loss of chromosome 16q was in 88 % of cases [13]. Mastracci et al. studied the genetic profile of atypical lobular hyperplasia (ALH) and lobular carcinoma in situ (LCIS), using microarray comparative genomic hybridization (CGH). They found a common alteration in both ALH and LCIS—the loss of 16q21-q23.1 [14]. This common genetic alteration highlights the possibility of a relationship between ILC and LCIS as they may be different forms of the same disease, and it seems that lobular carcinoma in situ (LCIS) is a precursor to infiltrating lobular carcinoma and therefore a marker of risk for breast cancer. This serves as an example of the application of genomic studies in order to discover breast cancer initiation, progression, and metastasis [13, 14].
Breast cancer gene expression profiling permits the collection of all the information about a whole set of genes in a tumor cell, including variations, gene expression, and the way those genes interact with each other and with the environment. The associations of specific genes with a common characteristic of expression or phenotypes are called gene profiles or gene signatures. Gene expression profiling has been used to create a complete new classification of breast carcinomas. The different kinds of clinical breast cancer have been correlated with diversity in gene expression profiles, which usually are studied using DNA microarray techniques [1]. Perou et al. using DNA microarrays in samples of breast cancer and normal breast tissue studied the presence of sets of genes that they called intrinsic genes, because these genes were found repeatedly inside the same patient biopsies but were not found repeatedly in all samples from other patients. A new group of genes associated with different breast cancer types were discovered, showing four distinct molecular subgroups: ER+/luminal-like, basal-like, HER2 enriched, and normal breast-like [15]. This new classification is being studied and recognized as distinct diseases with different treatment options [16, 17]. Table 1.1 summarizes the main findings of several studies that confirm the existence of the intrinsic subtypes, the technology used, and the novel finding that each study provided to this new breast cancer classification.
Table 1.1
Confirmation of the intrinsic subtypes with several studies
Study | Main technique | Other technique | Novel finding |
---|---|---|---|
Sørlie et al. 2001 [18] | cDNA microarrays | Hierarchical clustering | Division of luminal A and B subtypes |
Sørlie et al. 2003 [19] | cDNA microarrays | Hierarchical clustering | Association of BRACA1 with basal subtype |
Sørlie et al. 2003 [19] | cDNA microarrays | Hierarchical clustering | Correlation of ER status with intrinsic subtypes |
Abd El-Rehim et al. 2004 [21] | Tissue microarray | Immunohistochemistry | Correlation with cytokines expression |
Carey et al. 2006 [22] | Microarrays | Immunohistochemistry | Population-based distribution of subtypes |
Hu et al. 2006 [65] | Microarrays | Hierarchical clustering | Prediction of survival |
Novel Classification of Breast Cancer Based on Genome Profile: The Intrinsic Subtypes
Luminal Type
This type responds to endocrine therapy and has the best prognosis of all subtypes although it shows only limited chemosensitivity. Sorlie et al. studied a subdivision of luminal-like carcinoma Type A and Type B with characteristic molecular profiles and different prognoses (better for the A type than the B) [18]. In luminal B type, the HER2 expression is greater and responds only slightly better to chemotherapy than luminal A, which seems to have lower risk for relapse [18, 19].
Basal-Like
HER2 Enriched
This type is characterized by the overexpression of the HER2 gene, and it has no expression of genes characteristic of the luminal subtypes. Not all breast cancers with HER2 positive by immunohistochemistry are classified as HER2-enriched type by molecular profiling. The basal-like and the Her2 enriched seem the ones with worst prognoses, the luminal A the best, and the luminal B in the intermediate [18, 19].
Breast cancer tumors had been separated by expression of hormone receptor status defining biologically distinct phenotypes that are used on clinical basis for prognosis and treatment. Genomics links phenotypes to genotypes of breast cancer by means of the study of the intrinsic subtypes and their clinical characteristics. The relationship between breast cancer molecular subtypes and prognosis has been studied extensively by gene expression profiling in order to find the differences that are used in clinical basis using hormone receptor status, HER2 status, and other classical characteristics. Among the intrinsic subtypes, luminal A and B have been identified as the ones with high expression of hormone receptor, while the HER2-enriched and basal-like subtypes have low expression of hormone receptors. In addition, low claudin, interferon rich, androgen receptor, and normal-like are the other subtypes studied in new trials [19]. There are many studies that find a relationship between traditional prognostic factors with gene expression signatures [21–23].
Genomic Testing
The molecular subtypes in breast cancer have shown different tumor characteristics, tumor aggressiveness, and different response to certain types of chemotherapy. They all have the ability to progress to metastases, but with differences regarding their leaning toward relapse into different organs. Smid et al. used microarrays and analyzed them with statistical significance analysis of microarrays (SAM). Studying the preference for organ-specific metastases of each of the intrinsic subtypes, they found that the rate and location of relapses are correlated to different molecular subtypes and metastases conserve genetic similarities with their primaries [24].
Following the intrinsic gene research of today, a new classification of breast cancer exists using omics technologies. New trials show the possibility of more subtypes, but this pathway is barely started, and currently it is not possible to exactly compare the new subtypes with the classical ones, and it is necessary for more studies to set up many new targeted therapies for these new molecular subtypes [11, 15].
Applying this new genetic information on the cluster of genes related to breast cancer, and using the new subtypes or intrinsic types of breast cancer, there is now a connection between the lab and the patient with the creation of several molecular assay tests that use genomics to calculate risk assessment and prognosis, creating a personalized diagnosis, prognosis, and therapy. This genomic test calculates certain clinical outcomes, such as patient risk of relapse if avoiding chemotherapy or if treated only with hormonal therapy and patients having more global risk of relapse. Although these tests searched different genes, when they are applied within the same patient data, they show similar results on prognosis of breast cancer. The MammaPrint and Oncotype DX are the tests most used and studied [1, 3].
The MammaPrint analyzes 70 genes and shows which patients have a low-risk molecular profile and therefore can avoid chemotherapy. It also identifies those women with good clinical factors but who carry poor prognosis genes [1, 3, 4]. Van de Vijver used inkjet-synthesized oligonucleotide microarrays to study the 70-gene expression profile that is associated with prognosis in patients with breast cancer and validate the classification system to predict the likelihood of distant metastases within 5 years [25].
Oncotype DX uses the intrinsic subtype model with 21 genes and is used in estrogen and progesterone receptor-positive and lymph node-negative breast cancer, to identify patients who can avoid adjuvant chemotherapy [1, 3]. Paik et al., using reverse transcription polymerase chain reaction (RT-PCR), studied 21 selected genes and found the correlation with the likelihood of distant recurrence in patients with node-negative disease and treated with tamoxifen, validating then a recurrence score [26, 27].
Although these tests are beginning to be used in daily medical practice, there is still no consensus about their reliability or guidelines for use, because there is little information about the impact that these tests may have on clinical outcomes in the long term. Where there seems to be agreement is that the information provided by these tests must be taken into account as additional information to that obtained by conventional methods [16, 17].
Regarding the possibility of properly selecting the patient who should use chemotherapy and the one who should avoid it, as personalized medicine should be able to do, it seems necessary to relate a set of genes and its regulation systems with a clinical outcome [4, 11]. In order to accomplish more scientific evidence, prospective clinical trials—MINDACT for MammaPrint and TAILORx for Oncotype Dx— are trying to improve their clinical application [28–30].
One of the main drawbacks of applying past genomics research on a daily clinical basis is the lack of validation because these trials were based on small samples. That is why the latest research is focused on using more biopsy samples and more genetic analysis than the first trials, creating a genomics landscape of breast cancer [6]. Using larger samples in order to integrate breast cancer genome and transcriptome, and search for molecular drivers and gene expression, seems to be more reliable than obtaining information through the analysis of smaller samples [6, 31]. The creation of biobanks allows the storage of multiple samples of different types of cancer, from different patients, and treatments using different techniques of conservation. The existence of a useful biobank offers multiple and extensive databases using many categories, such as family samples, pre- and posttreatment samples, and population characteristics, to classify and identify the samples. To improve the biobank utilization, ethical issues must be addressed regarding the use of the samples for different studies [32].
One example of storage of new public oncogenome data is the Cancer Genome Atlas (TCGA), which is “a comprehensive and coordinated effort to accelerate the understanding of the molecular basis of cancer through the application of genome analysis technologies, including large-scale genome sequencing.” This atlas is created with the efforts of the National Cancer Institute (NCI) and the National Human Genome Research Institute (NHGRI) that are applying omics technologies to profile DNA, RNA, protein, and others that can be related to this disease. This data is being stored within several samples of cancer, creating a new list of genes, mutations, and molecules.
Now there are tons of genetic data related to breast cancer, but there is lack in the final connection with the actual patient [6, 11]. Curtis et al. used almost 2,000 samples of breast cancer to analyze their genome and transcriptome within copy number variants, single nucleotide polymorphism, and acquired somatic copy number aberrations, and they found new subgroups to create a novel molecular stratification of the breast cancer population [33]. The Cancer Genome Atlas network 2012 used the analysis of large amounts of data through the TGGA platform studying the DNA copy number arrays, DNA methylation, exome sequencing, messenger RNA arrays, microRNA sequencing, and reverse-phase protein arrays from breast cancer, showing the existence of four main breast cancer classes but associating them with several gene mutations and subgroups of proteins expression. They also found many molecular commonalities between basal-like breast tumors and high-grade serous ovarian tumors [34].
Epigenomics
Epigenetic modifications are the alterations found in DNA not affecting its primary structure, such as DNA methylation, histone modifications, and chromatin or nucleosomal remodeling; these alterations are heritable and reversible. Therefore epigenomics is the study of the complete set of those epigenetic modifications [7, 35].
DNA methylation, histone modifications, and chromatin or nucleosomal remodeling mutually interact with each other to regulate gene expression. The modifications obtained through them sometimes end up with aberrant transcription disrupting the normal regulation of a cell. The epigenetics processes modulation of chromatin structure to form a loosely packed DNA that is transcriptionally active, called euchromatin, or a tightly packed DNA that is transcriptionally inactive, the heterochromatin. Therefore, these processes can either turn on or turn off the gene expression.
Cancer seems to be driven by these epigenetic alterations, which cause aberrant cell regulation that result in a change in expression patterns of genes implicated in cellular proliferation, survival, and differentiation. Therefore, in breast cancer cells, DNA methylation, histone modifications, and chromatin or nucleosomal remodeling can lead to initiation, promotion, and maintenance of carcinogenesis and treatment failure. Understanding epigenetic alterations that initiate and maintain gene silencing and oncogene activation in cancer cells is necessary in order to find clinical applications of epigenomics [36–38].
DNA Methylation
DNA methylation is the addition of a methyl group into a region of DNA with participation of the DNA methyltransferase enzymes. They create genomic instability and rearrangement, activation of oncogenes, and inactivation of tumor suppressor genes, resulting in a cancerous cell [36]. Inside a cell there may be a loss of DNA methylation (hypomethylation) or there can exist an excess of DNA methylation (hypermethylation). Currently genes that are aberrantly methylated in breast cancer can be identified; with DNA methylation mapping technologies, it would be possible to identify DNA methylation patterns within a tumor subgroup that are correlated to breast cancer subtypes, clinical stages, and prognosis.
Methods for DNA methylation analysis can be divided into global methylation analysis and gene-specific methylation analysis. The global methylation analysis measures the overall level of methyl cytosines. The gene-specific methylation analysis uses enzymes to digest DNA to process it and quantify and identify methylated genes. One of the processes used is the polymerase chain reaction (PCR), a main technique for omics that amplifies a DNA sequence using an enzymatic reaction to obtain thousands to millions of copies of it. Methylation-specific PCR (MSP) is able to identify methylated sequences or hypermethylated zones inside of the genome [39, 40].
Dejeux et al. studied DNA methylation of certain genes from samples of patients with locally advanced breast cancer before the neoadjuvant treatment with doxorubicin. To quantify the modification, they used DNA methylation analysis by pyrosequencing. They found aberrant methylation in 9 of 14 genes studied, and three of the variations found are possible biomarkers for prognosis for patients with this disease and the prediction of response of this neoadjuvant drug [41].
Hsu et al. studied the methylation of BRCA1 promoter by methylation-specific PCR. They found a significant correlation within triple-negative cancer and with poor overall survival and disease-free survival in patients with breast cancer with methylated BRCA1 promoter [42].
Histones
Histones are protein components of chromatin that package and order the DNA into structural units called nucleosomes. The nucleosome is the fundamental unit of chromatin and it is composed of a structure of four histones around which the DNA is wrapped. Histone modifications and chromatin or nucleosomal remodeling are mediated by various enzymes, and it seems that a deregulation of normal DNA arrangement patterns turns on modifications that generate a new histone code, resulting in chromatin regions for transcription activation or repression, leading to changed gene expression as tumor suppressor genes and oncogenes [37].
The study of histone modifications is more difficult than finding DNA methylation and needs high-throughput techniques such as proteomic techniques that are described in more detail in the section “Proteomics.” One such technique is stable isotope labeling with amino acids in cell culture (SILAC), based on mass spectrometry [43]. Cuomo et al. used SILAC technology to quantify and study histone modification changes in breast cancer cells and compared them to normal breast tissue histone modifications. They found significant changes for histone modifications in cancer cells that could represent biomarkers or what they called “breast cancer-specific epigenetic signature” [39].
Immunohistochemistry (IHC)
Another combined omics technique, IHC uses the linkage between antigen and antibody to localize specific antigens in microarray tissues by labeling antibodies with fluorescent material [44]. Elsheikh et al. used this technology with breast cancer samples in order to detect specific histone marks. They found significance between low and high levels of individual histone marks with a range of clinical and pathologic variables of the disease; also they could relate histone modifications with tumor type beside a relationship among histone modifications with biological markers and phenotypic groups of breast cancer such as estrogen receptor, progesterone receptor, expression of luminal cytokeratins, and others [45].
Transcriptomics
Transcription is the process by which the DNA is copied into RNA. The RNA can be translated into peptides or proteins and used as a guide for the synthesis of proteins by ribosome. Also RNA can work as gene regulation and enzymatic activity [46]. Transcriptome is the collection of all cellular RNA, and transcriptomics is the study of the whole transcriptome, measuring and classifying all the RNA within a cell. Transcriptome includes different kinds of transcript, such as a messenger RNA (mRNA), noncoding RNA, and small RNA; the structure of genes where the transcriptions are located; and the expression of each transcript under different conditions [47]. Transcriptomic techniques profile all types of transcript, find the start and end sites of genes to locate where transcriptions occur, and understand the posttranscriptional modifications, enabling to decode all the RNA sequences in order to map and quantify it [46].
Gene Expression
Gene expression is the study of the activity of a gene that can be quantified by the measurement of DNA transcription into mRNA and mRNA into proteins [12]. Knowing oncogene expression provides additional information about the genetic processes that drive cancer. Various high-throughput technologies enable the measurement and classification of the transcriptome as different types of microarrays (cDNA and oligonucleotide microarrays) and next-generation sequence techniques [46].
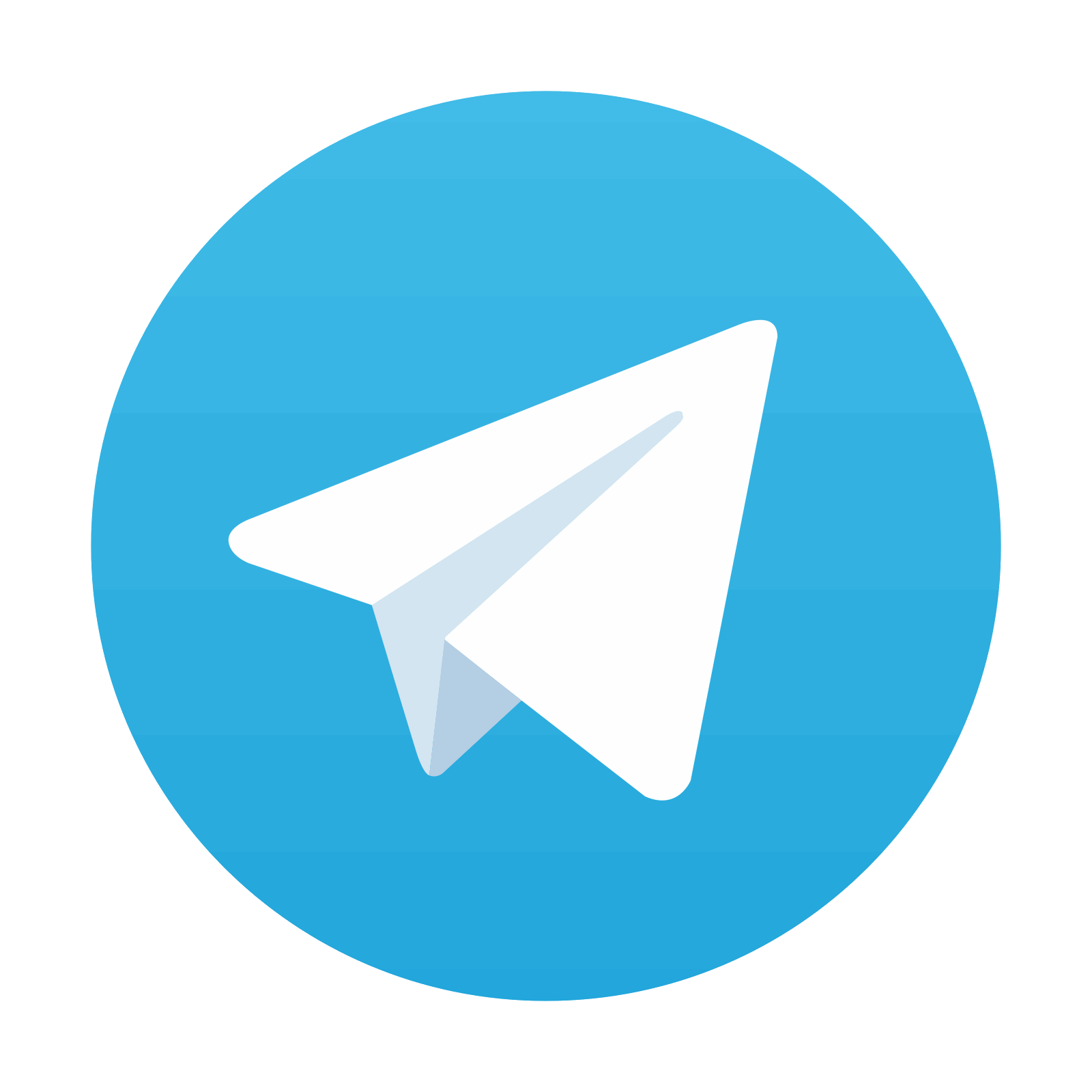
Stay updated, free articles. Join our Telegram channel

Full access? Get Clinical Tree
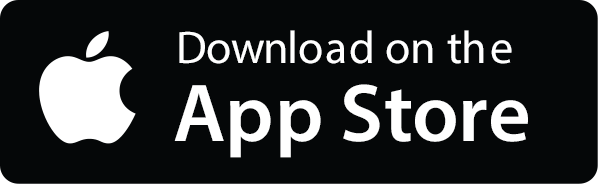
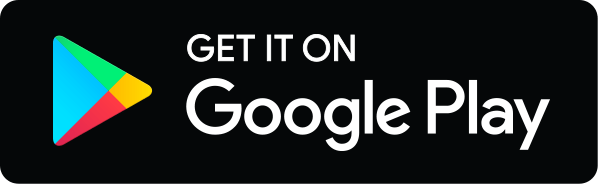