Fig. 1
Dried kokum plums (amsool)
In the following, an overview is presented of the miscellaneous biological activities of garcinol as obtained from preclinical evaluations concerning the treatment and prevention of cancer and metastases.
2 Chemical Aspects of Garcinol
Garcinol (also called camboginol, 1) is a benzophenone derivative with five isoprenyl side chains attached to the central phloroglucinol ring (Fig. 2). Chemically, it belongs to the class of type B polycyclic polyprenylated acylphloroglucinols (PPAPs) (Ciochina and Grossman 2006). To date, there are more than 100 known PPAPs subdivided into three different types A, B, and C depending on the position of the acyl group. Other natural products of type B PPAPs isolated from Garcinia and Clusia species are xanthochymol (2), the guttiferones A–E (3), and the clusianones (4, 5). For comparison purposes, hyperforin (6) from St. John’s wort (Hypericum perforatum) features a type A PPAP with a well-documented antidepressant and anxiolytic activity. Nemorosone (7, from Clusia species) and garsubellin A (8, from Garcinia subelliptica) are further important type A PPAPs with antimicrobial and anti-inflammatory activity. The group of type C PPAPs is rather small and represented by garcinielliptones K, L (9), and M from Garcinia subelliptica (Ciochina and Grossman 2006).
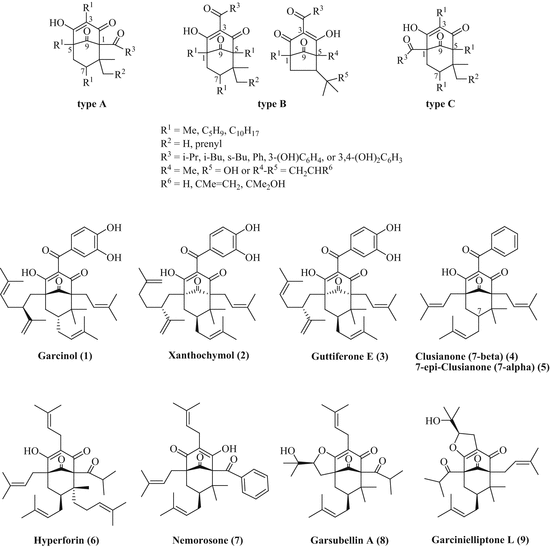
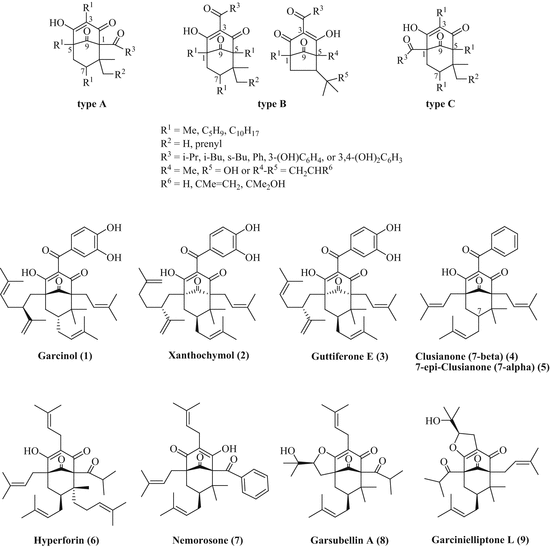
Fig. 2
Structures of natural type A, B, and C PPAPs
From the biosynthetic point of view, it was initially assumed that garcinol/camboginol and its close analogues might be derived from the benzophenone maclurin (2,3′,4,4′,6-pentahydroxybenzophenone) which is considered as a precursor for several xanthones in higher plants (Rao et al. 1980; Locksley et al. 1967). Indeed, there is evidence that PPAPs are offsprings of the less complex monocyclic polyprenylated acylphloroglucinols (MPAP) which are mainly discovered in plants of the Myrtaceae and Cannabinaceae families (e.g., in hops, Humulus lupulus) (Zhao et al. 2005). A polyketide-type biosynthesis involving one acyl-CoA (10) and three malonyl-CoA molecules was elucidated as to labelling and enzymologic experiments (Fig. 3). The formed tetraketide 11 undergoes Dieckmann cyclisation to acylphloroglucinol 12 (catalysed by benzophenone synthase in case of benzoyl derivatives), followed by enzyme-catalysed prenylation/geranylation with prenyl/geranyl pyrophosphate to MPAPs (13). Attack of one of the geminal prenyl groups of the MPAP molecule to another prenyl pyrophosphate generates a carbocation (14) which is ready to undergo cyclisation to type A (after C1 attack) or type B (after C5 attack) PPAP derivatives (Ciochina and Grossman 2006). In contrast to that, formation of the rare PPAPs of type C probably requires a quaternary centre adjacent to the acyl group (at C1).
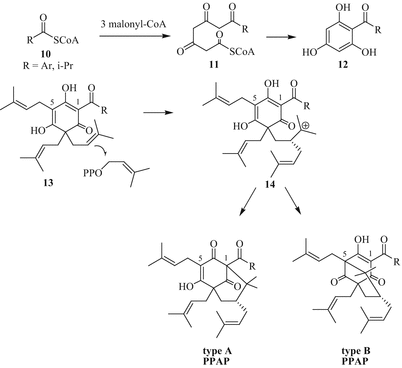
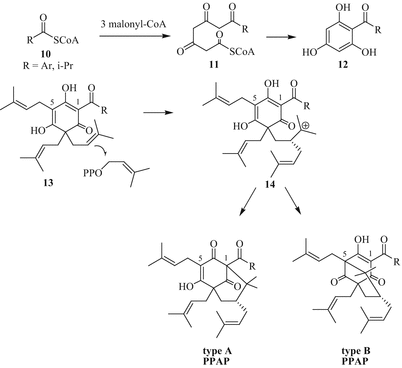
Fig. 3
Biosynthesis of type A and B PPAPs
Interestingly, optically active garcinol/camboginol (1, [α]30 −132.9°C, 1 % in CHCl3) was first described upon isolation from the latex of another Garcinia species, Garcinia cambogia (thus, the name camboginol is also casually applied for garcinol) (Rao et al. 1980). Despite this, garcinol is very abundant in the rinds of kokum plums and can be easily isolated from dried kokum/Garcinia indica fruits (also called amsool) in reasonable amounts yielding, for instance, up to 5 g of pure garcinol from 500 g of dried kokum fruits. In brief, commercially available dried kokum plums are chopped and extracted with methanol by stirring a methanolic kokum suspension with a mechanical stirrer. After filtration and removal of the solvent, water is added and the aqueous phase extracted with ethyl acetate. Purification of the ethyl acetate extract by column chromatography (silica gel 60, ethyl acetate/n-hexane 1:2) gives crude garcinol which is finally recrystallised from n-hexane as a pure yellow solid. The colorless pyrano-isomer isogarcinol (15, cambogin) is easily obtained from garcinol under acidic conditions and features the (−)-enantiomer ([α]30 −209.9°C, 1 % in MeOH) of the natural product isoxanthochymol (16, Fig. 4). An efficient thermal conversion of garcinol to isogarcinol (at 200 °C for 5 min) was likewise reported (Rao et al. 1980).
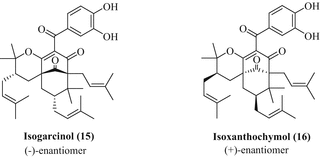
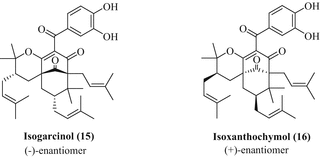
Fig. 4
Structures of the enantiomers isogarcinol (15) and isoxanthochymol (16)
Garcinol (1) possesses significant radical-scavenging properties due to its structure (catechol and β-diketone fragments). The oxidation products of garcinol have been identified and possess themselves significant biological properties. Mechanistically, the treatment of garcinol with AIBN or DPPH leads to abstraction of a hydrogen radical from garcinol forming a conjugated garcinol radical (17, Fig. 5). This garcinol radical converts to isogarcinol (15), to a hydroperoxide metabolite (18), or to xanthone derivatives garcim-1 (19) and garcim-2 (20) (Sang et al. 2001, 2002; Hong et al. 2007).
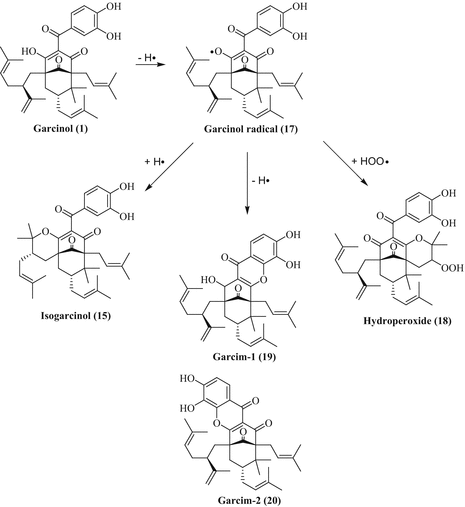
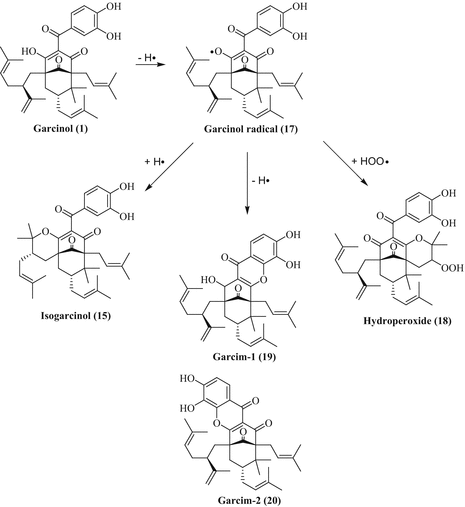
Fig. 5
Radical reaction of garcinol (1)
Although garcinol is readily available from its biological sources, total synthetic approaches toward PPAPs are generally of importance in order to obtain less abundant derivatives and for the design of new PPAP compounds with improved biological properties. The first total syntheses of PPAPs were accomplished for garsubellin A, a type A PPAP, by Shibasaki (2005) and Danishefsky (2006) (Kuramochi et al. 2005; Siegel and Danishefsky 2006). Meanwhile, total syntheses of additional PPAPs are available, including type B derivatives such as clusianones and guttiferone A. Simpkins and coworkers have disclosed the total synthesis of racemic clusianone in 2006 using an Effenberger cyclisation key step to generate the bicyclic trione fragment (Fig. 6) (Rodeschini et al. 2006; Schönwälder et al. 1984). Starting from the vinylogous ester 21 repeated prenylation gave a mixture of enol ethers 22 and 23, which underwent Effenberger cyclisation forming the bicycle[3.3.1]nonane-2,4,9-trione 24. Regioselective lithiation reactions led to the final product, racemic clusianone (4). A similar approach was applied by Marazano and coworkers starting from an enol silyl ether which was reacted with malonyl chloride under Effenberger conditions (Nuhant et al. 2007).
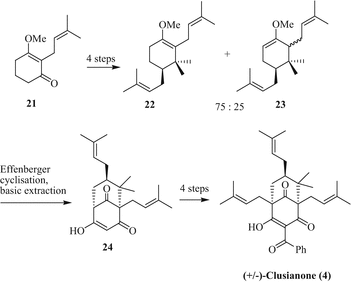
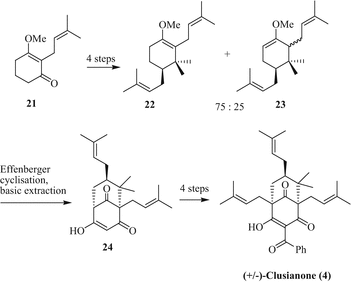
Fig. 6
Total synthesis of (+/−)-clusianone (4) by Simpkins
In contrast to that, Danishefsky and coworkers applied a iodonium-catalysed carbocyclisation to build up the bicycle 27 starting from commercially available 3,5-dimethoxyphenol (25, Fig. 7). Though the yield of the cyclisation step is low, the isolated side products can be recycled to the precursor 26. After introduction of the allyl and benzoyl groups, cross-metathesis of 30 with 2-methylpropene using a Grubbs second-generation catalyst and final methyl ether cleavage with aq. NaOH gives clusianone (4) (Tsukano et al. 2007).
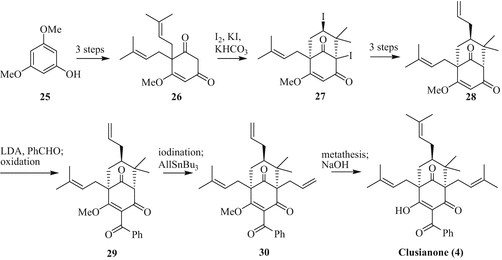
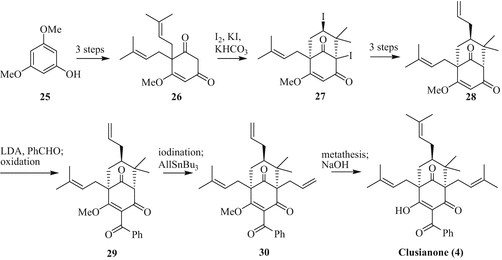
Fig. 7
Total synthesis of clusianone (4) by Danishefsky
Recently, Boyce and Porco Jr. have disclosed a six-step asymmetric synthesis of (−)-clusianone (4) from 5-methoxyresorcinol via benzophenones 31 and 32 using a biomimetic cationic cyclisation procedure (Boyce and Porco 2014). Allylation and Claisen rearrangement forms diallyl benzophenone 32 which is alkylated with the chiral alkyl O-triflate 33 to intermediate 34 (Fig. 8). Cationic cyclisation under acidic conditions generates the precursor (−)-35, which is converted to (−)-clusianone (4) via cross-metathesis using a Grubbs II catalyst. The same group has recently reported a quick route to PPAPs (both type A and type B) by Pd-catalysed dearomative conjunctive allylic alkylation of allyl deoxyhumulones (Grenning et al. 2014).
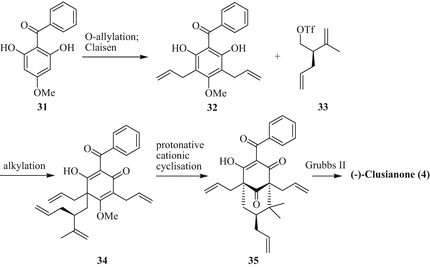
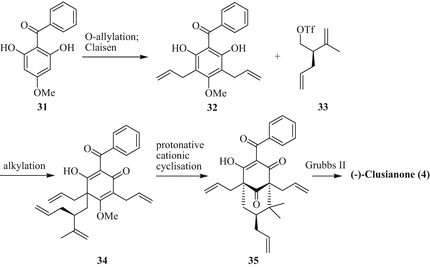
Fig. 8
Asymmetric total synthesis of (−)-clusianone (4) by Porco Jr
A different route was chosen by Plietker and coworkers to accomplish the total synthesis of the type B PPAPs epi-clusianone (5), oblongifolin A (40a), hyperpapuanone (40b), and hyperibone L (40c) (Biber et al. 2011). Starting from acetylacetone 36 the ketones 37 were prepared (Fig. 9). After Michael addition and Knoevenagel condensation followed by alkylation the cyclohexenones 38 were obtained. Subsequent alkylation (cuprate addition) gives the cyclohexenones 39 which were converted to the acylated bicyclo-nonatriones 40a–c and 5 via Dieckmann condensation and acylation in one step. Overall yields of this synthetic method ranged from 6 to 22 %. Meanwhile, the same group has disclosed the total synthesis of guttiferone A in a similar way (Horeischi et al. 2014).
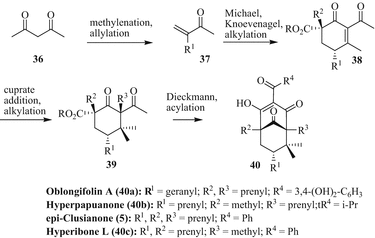
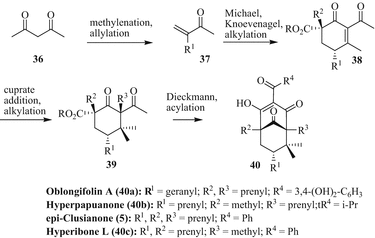
Fig. 9
Total syntheses of oblongifolin A (40a), hyperpapuanone (40b), epi-clusianone (5), and hyperibone L (40c) by Plietker
3 Garcinol, Inflammation, and Cancer
Much of the chemopreventive activity of garcinol (1) can be assigned to its radical scavenging properties against superoxide anions, hydroxyl radicals, and methyl radicals and, thus, protection of cellular components from lethal damage. Garcinol showed superoxide anion scavenging activity comparable with DL-α-tocopherol, a threefold higher 2,2-diphenyl-1-picrylhydrazyl (DPPH) scavenging activity than DL-α-tocopherol, and saved indomethacin-treated rats from acute ulceration (Yamaguchi et al. 2000). In addition, garcinol also inhibited protein glycation (Yamaguchi et al. 2000). F344 rats were protected from azoxymethane (AOM)-induced colonic aberrant crypt foci (ACF) by treatment with garcinol which caused decreased proliferating cell nuclear antigen (PCNA) index in ACF and increased liver glutathione S-transferase (GST) and quinone reductase (QR) activities (Tanaka et al. 2000). The expression of iNOS and COX-2 enzymes was significantly downregulated in the ACF of the garcinol cohort. Hence, pre-neoplastic progression in rat colons is efficiently suppressed by garcinol. Recently, Tsai and coworkers have shown that garcinol inhibits inflammation-associated colon carcinogenesis in mice induced by dextran sodium sulphate and azoxymethane by downregulation of iNOS, COX-2, and PCNA basing on inhibition of ERK1/2, PI3K/Akt, and Wnt/β-catenin signalling (Tsai et al. 2014). Oral carcinogenesis caused by 4-nitroquinoline-1-oxide (4NQO) was likewise silenced in F344 rats by feeding with garcinol which reduced cell proliferation rate and COX-2 levels in affected tongue tissues (Yoshida et al. 2005).
Neuroprotective effects of garcinol were also observed as well as reduction of nitric oxide (NO) radical levels in astrocytes treated with lipopolysaccharides (LPS) (Liao et al. 2005a). Indeed, formation of NO radicals seems to play a key role concerning inflammation and carcinogenesis processes. LPS-stimulated macrophages (murine RAW264.7 cells) and intestinal cells treated with garcinol revealed reduced arachidonic acid metabolism and NO synthesis (Hong et al. 2006). While treatment with garcinol after LPS stimulation might be conferred to inhibition of cPLA2 and ERK1/2 which are involved in arachidonic acid release from membrane phospholipids, garcinol treatment before macrophage stimulation with LPS might inhibit toll-like receptors. Again, suppression of COX-2 and of NF-κB proteins was observed which feature crucial factors of inflammation processes (Hong et al. 2006; Liao et al. 2004). In fact, blocked NF-κB signalling is involved in induction of apoptosis by garcinol in highly metastatic MDA-MB-231 breast cancer cells as well as in prostate and pancreatic cancer cells (Ahmad et al. 2010, 2011). The close garcinol analogue isogarcinol (15) also displayed anti-inflammatory activity by downregulation of iNOS, COX-2, and NF-κB and suppressed xylene-induced mouse ear edema and collagen-induced arthritis in vivo (Fu et al. 2014).
5-Lipoxygenase (5-LOX) and microsomal prostaglandin E2 synthase-1 feature additional important targets of garcinol (Koeberle et al. 2009). Suppression of 5-LOX and PGE2 was observed in human neutrophils and interleukin-stimulated human lung cancer cells after garcinol treatment. Inhibition of 5-LOX activity seems to be a particularly efficient strategy to combat metastases, and COX-2 catalysed PGE2 formation is often related with enhanced metastasis by upregulation of matrix metalloproteinases such as MMP-2 (Flavin 2007; Ito et al. 2004). 5-LOX inhibition by topical garcinol treatment suppressed 7,12-dimethylbenz[a]anthracene (DMBA)-induced hamster cheek pouch carcinogenesis by inhibition of leukotriene B4 (LTB4) biosynthesis (Xin et al. 2012). Inflammation and carcinogenesis in the oral epithelium were efficiently prevented by garcinol.
Hong and coworkers have shown that garcinol-based anti-inflammatory activity was observed due to inhibition of Janus kinase/signal transducer and activator of transcription (JAK/STAT)-dependent pathways in LPS-stimulated macrophages (Hong et al. 2006). Recently, a direct interaction of garcinol with the transcription factor STAT-1 was disclosed as well as inhibition of cytokine signalling by blocking STAT-1 transfer into the nucleus and STAT-1 binding to DNA (Masullo et al. 2014). STAT-3 features another STAT protein known for its cross talk with NF-κB in cancers leading to increased tumour progression and dissemination (Grivennikov and Karin 2010; Bollrath and Greten 2009). Thus, inactivation of STAT-3 has become a promising target for cancer therapy. There are already some curcuminoid derivatives which are able to inhibit the phosphorylation of the SH2 domain of STAT-3 by binding to the SH2 domain and, thus, suppress the dimerisation of STAT-3 to functional transcription factors (Bill et al. 2012; Lin et al. 2011). Due to the structural similarity to curcuminoids, it is not surprising that garcinol has exhibited similar effects. Ahmad and coworkers disclosed STAT-3 inhibition by garcinol in metastatic breast cancer as well as in prostate and pancreatic cancer cells (Ahmad et al. 2012a). STAT-3 inhibition was also documented in vivo from tumour remnants after oral application of garcinol (5 mg/kg/day) which likewise reduced MDA-MB-231 breast tumour growth significantly. Recently, garcinol treatment led to STAT-3 inhibition and suppressed in vivo tumour growth in human head and neck squamous cell carcinoma (HNSCC) xenografts and human hepatocellular carcinoma (HCC) xenografts, in the latter case also by inhibition of STAT-3 acetylation (see next Chapter “Effects of Garcinol on Chromatin-Regulating Proteins”) (Li et al. 2013; Sethi et al. 2014).
The chaperone Hsp90 (heat-shock protein 90) is involved in inflammation and cancer since it is critical for the stability of its client proteins such as IKK and iNOS. Recently, the Hsp90 inhibitory activity of garcinol was identified by a high-throughput screening of natural product libraries and the effects of garcinol on NF-κB activity and NO formation can be partially mediated via Hsp90 inhibition as well (Davenport et al. 2014). However, further tests are necessary to validate the initial experiments on Hsp90 inhibition by garcinol.
Apoptosis induction and interference with mitosis in proliferating cancer cells have been reported for garcinol featuring additional hallmarks of garcinol-mediated anticancer activity. In HL-60 leukaemia cells, garcinol induced apoptosis via cytochrome c release from mitochondria and activation of caspase-9, caspase-3, and caspase-2 and surpassed the pro-apoptotic activity of curcumin (Pan et al. 2001). PARP cleavage and DNA fragmentation were observed from garcinol-treated cells as well as upregulation of proapoptotic Bad and Bax. In garcinol-treated hepatocellular carcinoma cells, formation of ROS led to mitochondrial apoptosis induction and significant increase in the Bax/Bcl-2 ratio (Cheng et al. 2010). Autoxidation of garcinol to xanthones with enhanced anticancer activity was also observed; the derivatives garcim-1 (19) and garcim-2 (20) (Fig. 5) revealed an even higher activity than garcinol in colon cancer cells (Hong et al. 2007). Garcinol appears to be a selective anticancer agent. Distinctly stronger caspase-3-dependent apoptosis induction was discovered in intestinal cancer cells than in nonmalignant cells (Hong et al. 2007). A similar effect was observed in breast cancer cells (Ahmad et al. 2010). Interestingly, at low doses below 1 μM garcinol promotes cell growth, and, thus, the cell killing effect of garcinol depends on its dosage (Hong et al. 2007). Ahmad and coworkers have disclosed that apoptosis induction by garcinol in cancer cells is supported by blocked NF-κB signalling leading to reduced activation of anti-apoptotic Bcl-2 (Ahmad et al. 2008, 2010). Suppression of TGF-activated kinase 1 (TAK1) and inhibitor of IκB kinase (IKK) by garcinol seems to play a role for NF-κB downregulation by garcinol (Ahmad et al. 2010; Li et al. 2013). In addition, garcinol is able to activate the death receptors DR4 and DR5 and, thus, to enhance apoptosis induced by TRAIL, a cytokine in advanced clinical trials, solely in malignant cells (Prasad et al. 2010). Suppression of anti-apoptotic proteins such as survivin, XIAP, and cFLIP was observed after garcinol treatment, while garcinol-mediated apoptosis induction or sensitisation to TRAIL could not be detected in nonmalignant cells, which underscores the tumour-selective character of garcinol once again.
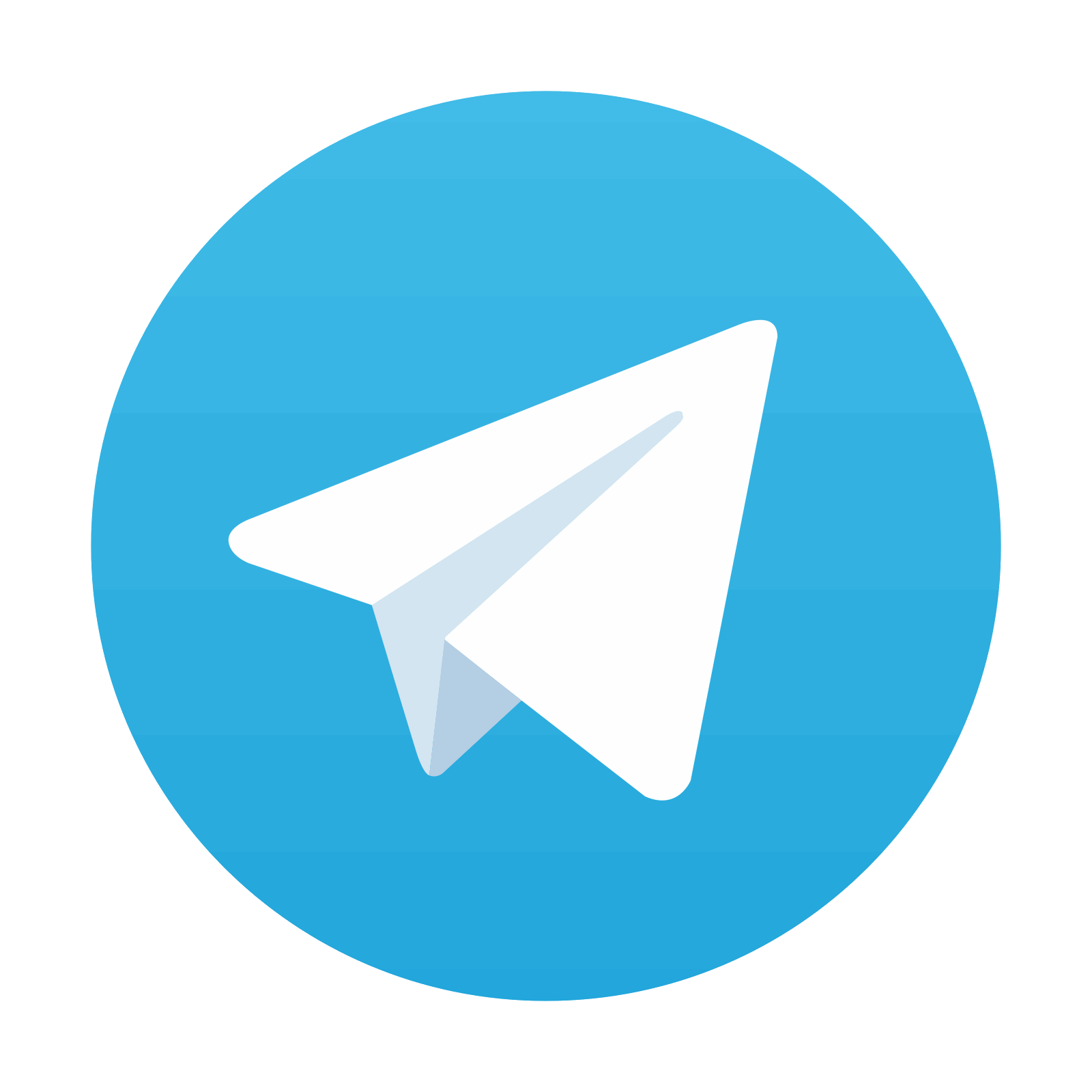
Stay updated, free articles. Join our Telegram channel

Full access? Get Clinical Tree
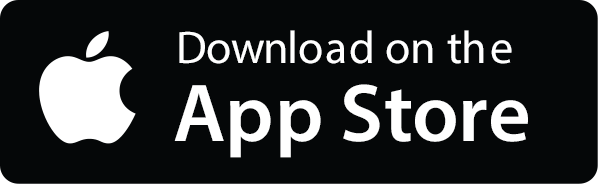
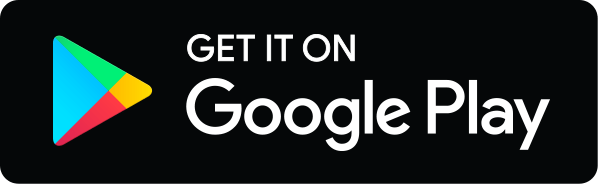