© Springer Science+Business Media New York 2016
Marina Kurian, Bruce M. Wolfe and Sayeed Ikramuddin (eds.)Metabolic Syndrome and Diabetes10.1007/978-1-4939-3220-7_88. Obesity and Cancer
(1)
Department of Surgery, University of Michigan, 2210 Taubman Center-5334, 1500 E. Medical Center Drive, Ann Arbor, MI 48109-5334, USA
Keywords
ObesityRisk factorCancerSystemic inflammationSystemic hormonal milieuAdipose tissueAdipocytes8.1 Introduction
Obesity is a strong risk factor for multiple types of cancer. The mechanisms underlying this association are multiple and include increased systemic inflammation, increased alterations in the systemic hormonal milieu, and direct paracrine tumor-promoting effects of adipose tissue, adipose tissue stem cells, and mature adipocytes. These complex mechanisms generate a carcinogenic milieu in multiple tissues in obesity.
8.2 Epidemiology
The risk ratios for the incidence of and mortality from multiple cancers increase with increasing body mass index (BMI). Endometrial cancer demonstrates the strongest association with obesity, with a mortality risk ratio of 1.5 for overweight women (BMI 25–30), 2.5 for class 1 obesity (BMI 30–35), and over 6 in class 3 obesity (BMI > 40). Other cancers that are strongly associated with obesity include colon, renal, pancreatic, and esophageal adenocarcinomas. Overall, obesity is estimated to be a dominant causative factor in up to 20 % of all cases of cancer [1, 2].
Exceptions exist to the positive correlation between obesity and cancer, including lung cancer, squamous cell cancer of the esophagus, cancers of the head and neck [1–3], prostate cancer [4, 5], and premenopausal breast cancer [2], all of which demonstrate no clear correlation with obesity, or in some cases, a negative correlation. Body weight-independent risk factors such as hormonal status and smoking may explain these exceptions to the otherwise strong association between obesity and cancer. Obesity not only affects cancer incidence and long-term mortality, but also influences treatment outcomes. For example, despite conflicting data regarding obesity’s effects on prostate cancer incidence, data clearly demonstrate decreased survival among obese patients compared to lean patients who develop prostate cancer [6]. Obese patients with colon cancer, lymphoma, and breast cancer also suffer worse treatment outcomes compared with lean patients with similar cancers matched for stage. The effects of obesity on treatment efficacy may underlie some of these observations, including under-dosing of chemotherapy, reduced delivery of radiation therapy, and technical challenges associated with extirpative surgery. Biologic effects of adipose tissue may also interfere with cancer therapy; increased aromatase activity from adipose tissue may interfere with aromatase inhibitor therapy in obese breast cancer patients [7], for example.
In contrast to these data, in some cases obesity appears to exert a beneficial effect on survival in patients already diagnosed with cancer, a phenomenon termed the “obesity paradox.” While a strong risk factor for developing renal cell cancer, obesity predicts extended survival in those with a diagnosis of renal cell carcinoma, even after controlling for tumor stage [8]; similar albeit conflicting data exist in endometrial, head and neck, rectal, and esophageal cancers. The mechanisms underlying such protective effects are poorly defined and the associated epidemiologic data are complex and debated. Some argue that increased adipose tissue stores provide a protective “nutrient buffer” for obese patients undergoing cancer treatment. Along these lines, obesity appears to be associated with decreased chemo- and radiation-therapy toxicities which may contribute to increased treatment efficacy [9]. Alternatively, artifact may underlie observations that support the obesity paradox; for example, limitations in BMI as a metric of obesity, which most studies utilize, may mask the effects of increased adiposity on cancer in older patients (who generally have higher levels of adiposity at lower BMI due to decreased muscle mass) or the disproportionate effect of visceral adipose tissue on cancer pathogenesis; alternative measures of obesity such as waist circumference or more sophisticated measures of body fat may reveal hidden associations with cancer. Selection bias and other statistical confounders have also been suggested as explanations for the obesity paradox [10]. The obesity paradox therefore remains controversial.
The relationship between obesity and cancer risk is complex and multiple variables impact on this association. Multiple factors indirectly related to obesity, including gender, ethnicity, dietary factors, the presence of metabolic diseases including diabetes, and differences in body habitus, including subcutaneous and visceral adiposity, all contribute to cancer risk independent of BMI and confound epidemiologic analyses. Nonetheless, despite these complexities, obesity is clearly a dominant independent risk factor for cancer.
8.3 Inflammation
Inflammation is a dominant mechanism underlying the pathogenesis of cancer. Inflammatory bowel disease, hepatitis, and pancreatitis among others are all associated with an increased risk of cancer in involved tissues independent of body weight. Immune leukocytes elaborate multiple cytotoxic molecules to carry out immune responses, all of which have potentially mutagenic effects; these include reactive oxygen species, free radicals, antibodies, and cytolytic proteins. Cytokine expression is also a fundamental component of immune and inflammatory responses; cytokines potentiate leukocyte activation and proliferation, propagating inflammatory responses via autocrine and paracrine effects. Cytokines exert multiple effects on nonimmune cells as well, including controlling endothelial cell function, angiogenesis, and proliferation and apoptosis in multiple cell types. Conceptually, the combination of increased elaboration of mutagenic inflammatory weapons combined with increased expression of pro-proliferative cytokines creates an environment predisposed to carcinogenesis.
Obesity is associated with a state of chronic systemic low-grade inflammation that has its genesis in adipose tissue and afflicts all tissues. This inflammatory state is a direct consequence of failure of adipocyte nutrient buffering in the obese state. With progressive obesity, adipocyte nutrient storage capacity is overwhelmed. Adipocytes increase in size to a diameter beyond 100 μm, the diffusion distance of oxygen, which is thought to establish a state of cellular hypoxia within adipose tissue. This hypoxic state, in parallel with direct, hypoxia-independent effects of nutrient toxicity, triggers a series of cell stress responses in adipocytes, including endoplasmic reticulum and oxidative stresses, which in turn lead to adipocyte apoptosis and necrosis. These events generate an inflammatory response designed to scavenge apoptotic and necrotic cells. This inflammatory response is characterized by a diverse leukocyte infiltrate that includes macrophages, T-cells, B-cells, NK cells, eosinophils, and other immune cell subtypes, within which adipose tissue macrophages play a central role, mediating local (adipose tissue-based) and systemic insulin resistance and elaborating multiple pro-inflammatory cytokines. Adipose tissue inflammation is further amplified as a result of direct effects of excess nutrients on leukocytes. Free fatty acids, glucose, and downstream metabolites such as diacyglycerol, ceramides, and advanced glycation end-products, directly trigger inflammation by binding and activating Toll-like receptors (TLR) and receptors for advanced glycation end-products (RAGE) expressed by leukocytes, creating a positive feedback loop that perpetuates adipose tissue inflammation. TLR and RAGE ligands thus represent direct molecular links between metabolism and inflammation.
Early in the development of obesity, adipocyte hypertrophy is modest and nutrient excess, cell stress, and inflammation remain confined to adipose tissue. With progressive obesity, however, adipocyte hypertrophic limits and nutrient storage capacity are exceeded, adipose tissue dysfunction progresses, and excess nutrients and metabolites overflow from adipose tissue into the systemic circulation. As other tissues not as well adapted to nutrient processing and storage as adipose tissue are exposed to excess nutrients and metabolites, the same cell stress processes that initially unfold in adipose tissue “metastasize” to other organs, establishing a low-grade inflammatory state in all tissues that underlies the pathogenesis of malignant and nonmalignant metabolic disease. Adipose tissue overflow and involvement of non-adipose tissues in inflammatory and cell stress processes form the basis of systemic metabolic disease.
Cytokines are central mediators of the inflammatory state in obesity and dominant effectors of carcinogenesis on the context of overweight and obesity. TNF-α was one of the first cytokines implicated in obesity. Bruce Spiegelman’s laboratory demonstrated in 1993 that adipose tissue in obesity was a primary source of TNF-α, and furthermore, that TNF-α played a central role in mediating insulin resistance in the context of obesity [11]. This discovery was one of the first demonstrations of an immunoregulatory role for adipose tissue and a mechanistic link between inflammation and metabolism. While other cytokines have been implicated in obesity-related inflammation, TNF-α remains a central player. TNF-α levels are elevated in adipose tissue and in serum in obese mice and humans. Independent of obesity, TNF-α also plays an important role in the pathogenesis of multiple types of cancer. TNF-α has been implicated in cancers of the skin, liver and lymphoid system in murine models; TNF-α knockout mice are protected from chemically induced skin and colon cancers [12, 13]. Similar murine models implicate other inflammatory cytokines including IL-1, IL-6, and IL-8 in carcinogenesis, and expression of these cytokines is also elevated in obesity. Human data confirms a role for cytokines in cancer pathogenesis: for example, IL-6 and IL-8 gene polymorphisms are linked to gastric, colorectal, and esophageal cancers [14, 15]. Adipose tissue macrophages are a dominant source of inflammatory cytokines in obesity, although other cells within adipose tissue, including T-cells, B-cells, and adipocytes, also express inflammatory cytokines.
The specific mechanisms by which inflammatory cytokines contribute to carcinogenesis are multiple. Cytokines activate inducible nitric oxide synthase, and the resultant increase in nitric oxide levels has been shown to promote tumor proliferation, metastasis, and angiogenesis in multiple models [16]. Cytokines trigger NFκB signaling, a central cell signaling pathway in carcinogenesis that promotes inflammation and regulates cancer cell proliferation and apoptosis to provide a growth advantage to cancer cells [17, 18]. Cytokines are also tropic factors, promoting cell proliferation in most non-transformed and transformed cells via multiple signaling pathways that include not only NFkB, but JAK/Stat, Akt, AMPK, and mTOR signaling. For example, TNF-α promotes proliferation of stromal and endothelial cells, potentiating fibrosis and angiogenesis within the tumor microenvironment. IL-1 is similarly required for angiogenesis and tumorigenesis in multiple animal models [19, 20]. IL-6 and IL-8, also increased in expression in obesity, demonstrate a pro-proliferative action on a broad range of cell types. Finally, virtually all cytokines play important roles in cell adhesion, chemotaxis, and migration, which along with other inflammatory chemokines, may contribute to tumor metastasis.
Type II diabetes spans the interface between metabolism, inflammation, and carcinogenesis, and is an independent risk factor for multiple cancers, with the highest risk ratios associated with pancreatic and liver cancers. Inflammation is a major contributor to the pathogenesis of diabetes independent of cancer. Aspirin, a potent anti-inflammatory agent, was shown to ameliorate diabetes as early as 1901 [21], and modern anti-inflammatory drugs such as salsalate, a salicylate-derivative, as well as anti-TNF-α and anti-IL-1 antibodies are currently in clinical trials as therapy for diabetes [22–24]. Parallel data demonstrate that long-term therapy with aspirin and non-steroidal anti-inflammatory drugs reduce cancer risk [25, 26]. These observations demonstrate that inflammation, metabolism, and carcinogenesis are intertwined, and that inflammation-based therapy for metabolic disease has potential as cancer therapy.
8.4 Endocrine Causes of Obesity-Related Cancer
Nutrient excess induces marked alterations in the systemic hormonal milieu characterized by increased expression of multiple anabolic growth factors, including insulin, IGF, steroid hormones, adipokines, and gut hormones. This hormonal environment promotes proliferation of pre-neoplastic and neoplastic cells.
Insulin plays a dominant role in promoting a carcinogenic hormonal milieu in obesity. As adipose tissue buffering capacity is exceeded, free fatty acids spill into the systemic circulation, inducing systemic lipotoxicity in peripheral tissues. Skeletal muscle and the liver are primary targets. These tissues respond to excess lipids by shifting energy utilization from glucose to fatty acid oxidation. This shift in cellular energy homeostasis is the result of a transcriptional program that involves decreased expression of insulin receptors, glucose transporters, and insulin signaling molecules and increased expression of enzymes involved in fatty acid catabolism. These changes underlie skeletal muscle insulin resistance and lead to systemic hyperglycemia, to which pancreatic islet beta cells respond with a compensatory increase in insulin secretion. As diabetes progresses, pancreatic beta cell exhaustion eventually occurs as a result of chronic over-secretion of insulin, leading to end-stage insulin-dependent diabetes. Prior to this, however, cells throughout the body are exposed to elevated insulin levels. These responses underlie the development of peripheral insulin resistance and hyperinsulinemia, central metabolic features of obesity.
Hyperinsulinemia in obesity has broad effects beyond glucose homeostasis. Insulin is a potent growth factor that promotes proliferation and inhibits apoptosis in virtually all benign and malignant cells. Insulin has also been shown to induce tumorigenesis in multiple in vitro and in vivo models. Increased insulin levels in humans are independently associated with multiple cancers, including those most strongly associated with obesity, such as colon, endometrial, pancreas, and breast [27–29]. Insulin receptor expression is increased in multiple cancers, including breast, prostate, hepatocellular, and leukemic cancers [30, 31]. Insulin also promotes the expression of insulin-like growth factor-1 (IGF-1), a hormone secreted by the liver that stimulates the growth of numerous cancers. IGF-1 mediates its tropic effects by binding its own receptor as well as the insulin receptor, both of which are over-expressed in many tumors [30]. IGF-1 also induces angiogenesis which has been linked to cancer progression [32]. Serum IGF-1 levels are increased in many cancers in humans, and murine studies demonstrate a growth-promoting effect of IGF-1 on the growth of multiple types of cancer [27, 29].
Steroid hormones also contribute to obesity-related cancer. Steroid hormones are important contributing agents in multiple cancers independent of obesity, including breast, endometrial, and ovarian cancers, and serum levels of steroid hormone levels correlate directly with the risk of these cancers in humans [33, 34], while numerous in vitro and in vivo experimental models support a role for steroid hormones in tumor progression. A number of distinct mechanisms contribute to increased steroid hormone levels in obesity. Estrogen levels are increased as a result of increased adipose tissue mass, a site of conversion of androgens to estradiol by aromatase which is expressed in white adipose tissue. In postmenopausal women, adipose tissue becomes a dominant source of estrogen as ovarian estrogen secretion diminishes; in obese premenopausal women, adipose tissue may also represent a dominant source of estrogen. Increased adipose tissue mass leads to increased expression of TNF-α, IL-6, and leptin, all adipose tissue products, and these cytokines and adipokines induce aromatase expression by adipocytes, exacerbating adipose tissue steroidogenesis [35]. Furthermore, insulin, IGF-1, and glucose, all increased in obesity, inhibit expression of sex hormone-binding globulin in the liver, increasing systemic steroid bioavailability [36]. Finally insulin induces ovarian and adrenal androgen synthesis, increasing androgen levels as well as estradiol levels by providing androgen substrate for adipose tissue aromatase. Serum levels of steroid hormones correlate positively with BMI [37], and are decreased with weight loss, with a reduction in cancer risk [38, 39]. Induction of obesity with high-fat diet in animals is associated with increased incidence and growth of steroid hormone-sensitive tumors. Steroid-sensitive breast cancer is strongly associated with obesity, which is more often associated with estrogen receptor (ER)+ breast cancers [40, 41]. Furthermore, while ER+ breast cancers generally have a more favorable prognosis than ER− tumors, this may not be true in the obese, as mortality associated with ER+ tumors is substantially higher in obese patients compared with lean patients [42], suggesting that elevated circulating estrogen in obesity may stimulate ER+ breast cancer growth.
Dysregulated adipokine expression and activity contributes to the pro-carcinogenic state in obesity. Leptin, secreted primarily by adipocytes, and adiponectin, secreted by both adipocytes and adipose tissue stromal cells, are dominant adipokines with pleiotropic and opposing effects. Leptin expression is increased in obesity, and while conflicting functional data exist, for the most part leptin appears to promote metabolic disease, insulin resistance, and inflammation. Adiponectin in contrast is expressed at lower levels in obesity compared to lean states, and manifests effects that oppose leptin, attenuating metabolic disease, insulin resistance, and inflammation. Leptin and adiponectin manifest similar opposing effects on neoplastic growth, as serum levels of leptin correlate directly, and adiponectin levels correlate indirectly, with the risk of multiple cancers, including breast, colon, prostate, endometrial, gastric, colorectal, and leukemic cancers [43–46]. In vitro and in vivo models are also consistent with this dichotomy, with leptin promoting and adiponectin inhibiting proliferation, growth, angiogenesis, and invasion of multiple types of cancer cells and tumors in in vitro and in vivo models. Cancer cells mutate to take advantage of the effects of adipokines on tumorigenesis: hepatocellular and breast cancers up-regulate expression of the leptin receptor, a phenomenon that associated with worse prognosis [47]. Numerous adipokines in addition to leptin and adiponectin are dysregulated in obesity, contribute to metabolic disease, and are implicated in cancer pathogenesis, including visfatin, resistin, and apelin. This family of proteins regulates diverse aspects of physiology that contribute to cancer progression, and targeting adipokine signaling holds promise as cancer therapy. Leptin antagonist peptides and adiponectin agonists are under study in preclinical models as potential therapeutic agents for breast endometrial and cancers [48, 49].
8.5 Adipocyte-Tumor Cross Talk
Emerging data demonstrate that adipose tissue, adipose tissue stem cells, and mature adipocytes directly promote cancer initiation and progression. Histologic data from human cancers support a role for adipocytes in cancer progression: steatosis in the liver and the pancreas has been linked to hepatocellular and pancreatic cancers, while adipocyte infiltration of pancreas and breast tissues is associated with increased disease progression and aggressiveness of cancers in these organs [50–52]. Data from in vitro cell culture models as well as in vivo murine models of cancer demonstrate that adipocytes potentiate proliferative and invasive capacities and in vivo progression and metastasis of multiple cancers [53–56].
The anatomic basis of adipocyte-tumor cross talk is complex, and adipocytes and other adipose tissue components influence pre-neoplastic and neoplastic cells via multiple endocrine and paracrine mechanisms. Adipokines and steroid hormones secreted directly from canonical adipose tissue depots, as well as secondary endocrine effects of increased adipose tissue mass (e.g., hyperinsulinemia), exert tropic endocrine effects on multiple target tissues and have been implicated in carcinogenesis. Many tumors associated with obesity reside near anatomic adipose tissue depots, including renal, pancreatic, hepatic, and colon, and are thus also subject to paracrine effects of adipokines and other adipocyte products. Renal and pancreatic cancers arise in tissues surrounded by retroperitoneal and visceral fat and demonstrate strong correlations with BMI. In addition to the endocrine effects of formal anatomic adipose tissue depots on remote tissues, adipocytes are central components of the stromal microenvironment of multiple tissues in which tumors arise, and thus exert paracrine pre-neoplastic and neoplastic cells. Finally, provocative data demonstrate that adipose tissue stem cells are recruited to tumor tissues from remote sites, and migrate to tumors in response to tumor-secreted chemotactic factors and differentiate into adipocytes, fibroblasts, and endothelial cells that contribute to the tumor stromal microenvironment [57].
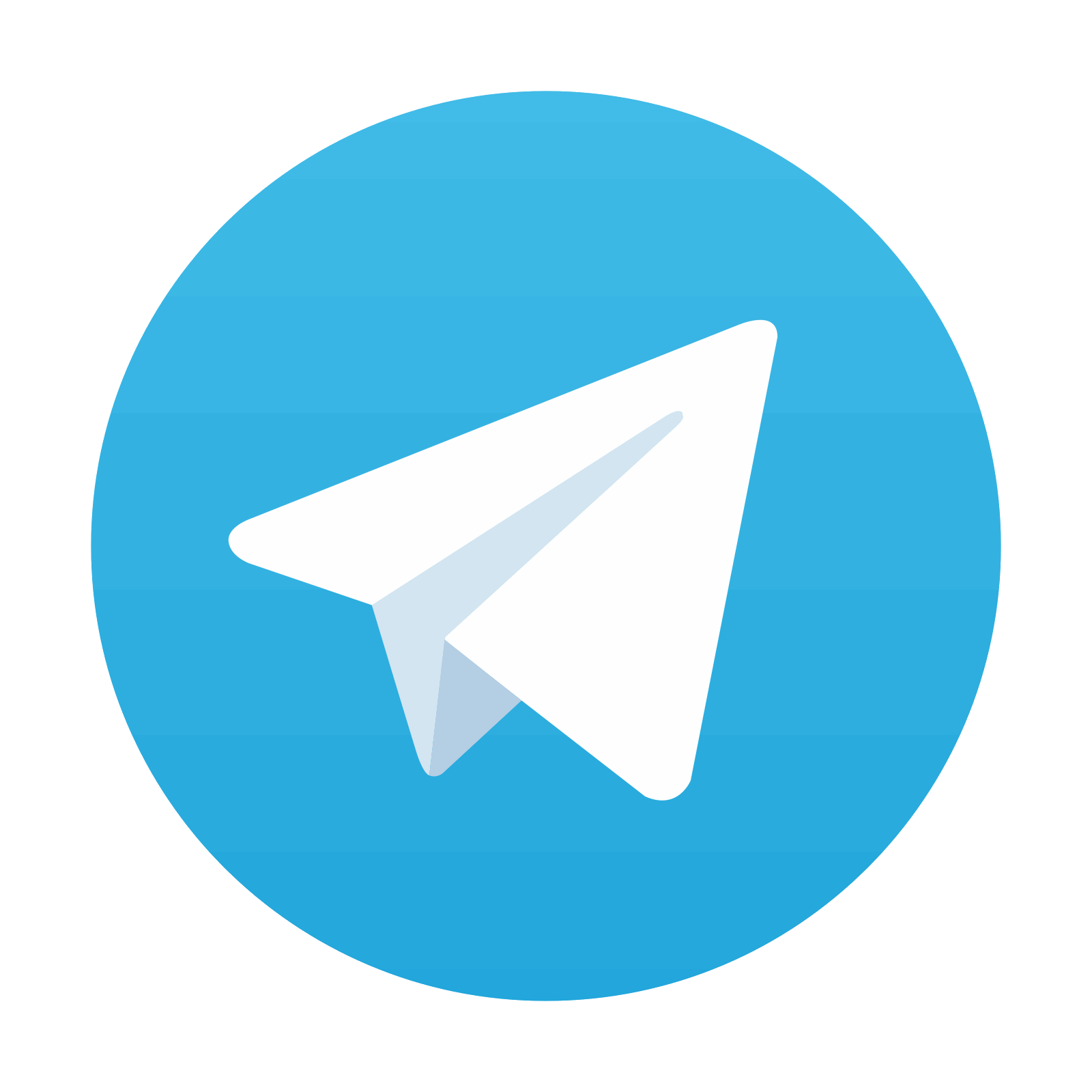
Stay updated, free articles. Join our Telegram channel

Full access? Get Clinical Tree
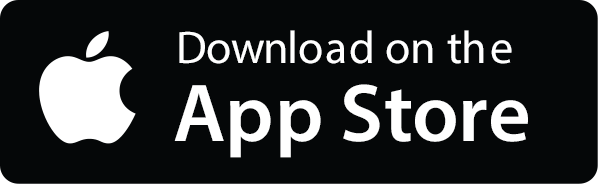
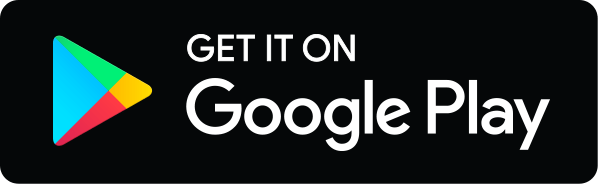