Fig. 2.1
Schematic interaction of the effectors of innate and adaptive immunity. Abbreviations: TC tumor cell, DC dendritic cell, Th1 and Th2 T helper cells of types 1 and 2, NK natural killer cell, CTL cytotoxic T-lymphocyte
NK cells can regulate immune responses by activating DCs and promoting their differentiation into mature, high IL-12-producing type-1 polarized DCs (DC1) with enhanced capacity to induce Th1 and CTL responses, the response most desirable against cancer [3]. Conversely, the innate and effector functions of NK cells require close interactions with activated DCs. Cell membrane-associated molecules and soluble mediators, including cytokines and prostaglandins (PGs), contribute to the bidirectional cross talk between DCs and NK cells [4, 5].
NK cells use an array of innate receptors to sense their environment and respond to alterations caused by infections, cellular stress, and transformation. The activity of NK cells is controlled by balancing inputs from activating and inhibitory receptors. The most important ligands for inhibitory receptors are MHC-I molecules. Since normal cells express high levels of MHC-I, they are most often protected from NK cell killing. In contrast, target cells expressing downregulated levels of MHC-I are seen as “missing self” and killed [6, 7].
Three predominant superfamilies of NK cell receptors (NKRs) have been identified that can either inhibit or activate NK cell function: killer immunoglobulin (Ig)-like receptors (KIRs) that bind to classical class I MHC molecules, C-type lectin receptors that bind to nonclassical class I MHC molecules or “class I-like” molecules, and natural cytotoxicity receptors for which ligands are currently not well defined [8]. The different NK cell subsets show important differences in their cytotoxic potential, capacity for cytokine production, and responses to cytokine activation. The CD56bright NK cells are the major population of NK cells that produce immunoregulatory cytokines, including interferon-γ (IFN-γ), tumor necrosis factors (TNF-α and TNF-β), granulocyte-macrophage colony-stimulating factor (GM-CSF), and interleukins (IL-10 and IL-13) after monokine stimulation. On the other hand, immunoregulatory cytokine production by CD56dim NK cells is negligible even following specific stimulation [9].
The above-described characteristics and functions show that NKs are obviously a valuable source for adoptive antitumor immunotherapy and they can not only recognize and lyse transformed cells with no or low expression of MHC and tumor-associated antigens but also play an important role in regulation of immune reactions, which makes a rationale for combination of antitumor vaccines and NKs in immunotherapy approaches.
2.3 Adoptive IL-2/LAK (or CIK) Therapy of Cancer
IL-2 stimulation of lymphocytes results in generation of the so-called LAK cells. LAKs are a heterogeneous population of lymphocytes that include primarily NK, NKT, and T cells, which are cultured in vitro from peripheral blood mononuclear cells (PBMC) in the presence of IL-2. The major effector subset in the LAK population is NK cells, which are mechanistically regarded as peripheral blood NK cells but are more cytotoxic against tumor cells, including NK-resistant targets [10].
The first true clinical progress in immunotherapy was seen after the introduction of recombinant DNA technology used for production of immune-stimulating cytokines. Since 1985, several studies on combined IL-2 and LAK cell treatment have been performed and the results were published [11–15].
Such clinical trials have shown that high-dose IL-2 alone or in combination with LAK cells mediates objective tumor regression in 17–28 % of patients with metastatic renal cancer or metastatic melanoma, while prolonged remission was even observed in some patients with metastatic cancers [16]. Some authors have reported on clinical trials of the systemic treatment with high-dose IL-2 and tumor-infiltrating lymphocytes (autologous lymphocytes can be isolated from tumor-infiltrating cells, which presumably express tumor-specific TCRs) of patients with advanced cancer. Such treatment resulted in a 34 % objective response rate of patients with metastatic melanoma [17]. Although there was considerable clinical interest in LAKs for antitumor therapy by the end of the last century, LAK therapy has failed to obtain public support as a standard therapy for cancer patients. This was largely the result of limited response to immunotherapy when compared with those to chemotherapy or radiation therapy, and there were concerns about toxicity associated with the IL-2 infused simultaneously in order to maintain LAK activation. Another confounding factor was that most studies on immunotherapy used terminal-stage patients with virtually no remaining immune response capabilities, as they had failed to respond to previous conventional treatments [18].
More recently, a new cell-based immunotherapy utilizing activated lymphocytes has been suggested as an adjuvant regimen to radical surgery of cancer patients. Kimura and coauthors conducted a randomized trial of 174 patients with non-small-cell lung carcinoma comparing IL-2/LAK therapy in combination with chemotherapy vs. chemotherapy alone [19]. Patients had undergone curative resection of their lung carcinoma and received six to eight courses of IL-2/LAK therapy over 2 years. The authors reported an improvement in the 5- and 9-year survival rates of 21 and 28 %, respectively. Other studies involved cytokine-induced killers (CIKs) for adjuvant treatment of solid tumors. CIK cells are a heterogeneous subset of ex vivo expanded T lymphocytes presenting a mixed T-NK phenotype and have unrestricted MHC antitumor activity [20]. In the setting of hepatocellular carcinoma and gastric cancers, adjuvant infusions of autologous CIK cells after surgical resection resulted in a significant increase in disease-free survival [21–23].
To improve IL-2/LAK immunotherapy effectiveness, local and locoregional infusions were performed, allowing for the effective concentration of activated killers at the site of the lesion. The most significant clinical effects were achieved with intra-cavity infusions of IL-2 and LAKs in patients with malignant effusions (pleuritis, ascites, and pericarditis). Malignant effusion regression was seen in 70–95 % of cases, showing good tolerance and effectiveness in chemotherapy-resistant cancer types [24]. One of the advantages of adjuvant locoregional immunotherapy is that these low IL-2 immunostimulating doses cause no marked side effect, including immune- and/or myelosuppression, which are characteristic of high-dose cytokine therapy.
These LAK- and CIK-cell immunotherapy methods aim to stimulate the innate chain of antitumor immunity, which is a reasonable approach because most tumors express little to no MHC or tumor antigens. It is also necessary to consider the fact that T killers constitute an essential part of lymphoid cell populations and are responsible for a more specific mechanism of action – in these conditions, they are not involved in the antitumor defense function.
2.4 Tumor-Infiltrating Lymphocytes (TILs) in Cancer Immunotherapy
The basic stage of antitumor immunotherapy is the generation of lymphocytes that specifically recognize tumor cells. T cells recognize short peptides derived from proteins biodegradable in nuclear cells and presented in the context of MHC molecules on the cell surface. Adoptive cell transfer is a treatment strategy that allows activation and expansion of tumor-reactive T cells ex vivo for subsequent reinfusion to the autologous host. Hundreds of peptides restricted to presentation on different subclasses of MHC molecules and derived from tumors of different histological types have been identified over the last decades [25]. Tumor-associated antigens fall into several major categories: (1) overexpressed normal proteins (e.g., carcinoembryonic antigen (CEA) or nonmutated p53); (2) non-mutated differentiation antigens (e.g., MART-1, overexpressed in melanoma and found in normal melanocytes); (3) cancer-testis antigens (CTA), consisting of non-mutated genes expressed during fetal development, then silent in normal adults. The description of TILs derived from a variety of histological cancer types demonstrated that cellular immune reactions against established malignancies exist in humans. TILs are heterogeneous populations of mononuclear leukocytes, which include not only CD4+ and CD8+ T lymphocytes (as previously reported) but also a small and, in some cases, significant fraction of γδ T cells, with a prevalence of the Vδ1 subset [26] as well as macrophages. TILs that infiltrate melanoma can specifically recognize tumor-associated antigens [27]. Tissues reactivated in cancer cells across multiple malignancies (e.g., MAGE and NY-ESO); (4) mutated antigens, unique to a single tumor or shared by a group of tumors (e.g., BRAF with the V600E mutation in melanoma and other solid tumors, or EGFRvIII in glioblastoma) [28].
Some authors presented early results in patients with metastatic melanoma treated with the adoptive transfer of autologous TILs selected for antitumor activity – expanded in vitro and then reinfused into patients along with IL-2, following a lymphodepleting preparative regimen [29–32].
In clinical trials with increasing lymphodepletion prior to infusion of autologous TILs, objective response rates between 49 and 72 % were seen for patients with metastatic melanoma [33]. Limitations of TIL therapy, including the requirement for surgery to isolate the tumor and the need to consistently generate T cells with antitumor activity, have led to novel strategies for redirecting normal T cells to recognize tumor-associated antigens (e.g., NY-ESO-1, CEA (carcinoembryonic antigen), anti-CD20) using genetically engineered tumor antigen-specific TCRs or chimeric antigen receptor genes. As an alternative to TIL therapy, highly avid TCRs can be cloned from naturally occurring T cells, and then gene transfer vectors can be used to introduce these into the patient’s lymphocytes. In this manner, large numbers of antigen-specific T cells can be rapidly generated, in comparison with the long-term expansion required for TILs. These highly reactive T-cell clones are able to recognize and effectively lyse target tumor cells [34–36].
Recently, several clinical trials have reported clinical efficacy and benefit of gene-modified T cells for treatment of different cancers, including melanoma, colorectal and synovial cell cancers, neuroblastoma, and lymphoma. In patients with synovial cell cancer, the measurable response rate was 66 %, compared to 45 % in melanoma patients [37–39]. However, though a number of studies showed effective TIL therapy, the complicated methodology of lymphocyte isolation from tumors and generating a purified appropriate TIL culture still remains a strong limitation. This laborious method is mainly applied in melanoma treatment because this tumor type provides a sufficient number of lymphocytes. Besides, to achieve TIL’s effect, lymphodepletion by means of chemotherapy or radiotherapy is needed, which is considered to extend the TIL’s active period. Therefore, TIL therapy has a number of essential limitations resulting from the necessity to obtain an appropriate tumor sample and then isolate lymphocytes, as well as the necessity of chemotherapy or radiation therapy for lymphodepletion.
On the other hand, a promising area of TIL implication is the treatment of malignant effusions (pleuritis, ascites, and pericarditis). TILs from such metastatic material are available in large numbers and may be easily expanded ex vivo in the presence of IL-2 or INFs.
We performed a clinical trial on evaluation of the effectiveness of intrapleural IL-2/LAK immunotherapy in 85 patients with malignant effusions—primary tumor types included lung cancer, breast cancer, mesothelioma of pleura and other cancer localizations. Autologic LAKs were generated from TILs—lymphocytes of the patient’s pleural effusions. Prior to IL-2/LAK therapy, most patients (56 %) with malignant effusions received radiation and chemotherapy including intrapleural infusion of cytostatics, which had no clinical effect.
Before the beginning of the immunotherapy 500–2,800 ml of serous or serous hemorrhagic liquid was evacuated from pleural cavity. Cytological examination of pleural effusion was performed in all cases.
In most cases, one-sided pleuritis developed with equal frequency from the right or left side. In 7.7 % of cases, two-sided accumulation of pleural effusion was registered; such patients had drainage firstly in one pleural cavity, then if clinical effect was achieved, the other one was drained.
Intrapleural infusion of IL-2 and LAKs (generated from autologous TILs) achieved clinical effect in 88 % of patients of whom 60 patients had complete remission and 10 experienced partial reduction of effusion (Fig. 2.2a, b). Recurrence of effusion occurred in 10 (11.8 %) patients after 1.2–2.5 months of the treatment completion. However, repeated one to two courses of IL-2/LAK therapy resulted in regression of malignant effusion. It is important to emphasize that delay or cessation of effusion was achieved only in cases which pleural liquid contained essential number of activated lymphoid cells including immunoblasts and mitoses.
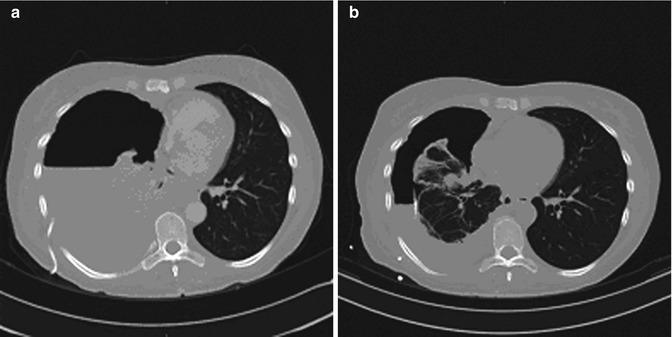
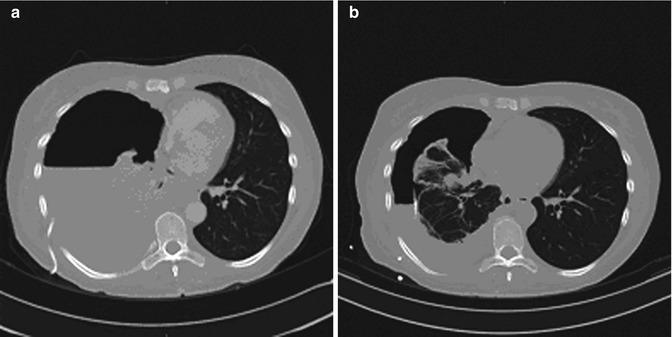
Fig. 2.2
CT of the chest during the course of IL-2/LAK immunotherapy of malignant pleural effusion. Patient Sh. Lung cancer (the right lung), right-sided pleuritis. (a) Prior to IL-2/LAK intrapleural immunotherapy; (b) Two months after the immunotherapy. Partial effect
Eight patients had repeatedly several immunotherapy courses due to encapsulated pleuritis. The second course was performed after 1 month interval, and IL-2 intrapleural infusion was accurately administered into small (up to 150 ml) residual cavities; clinical effect was registered in all these cases.
Plasmic part of effusion after elimination of tumor cells if necessary may be reinfused intravenously to maintain homeostasis of cancer patients. Indications to such reinfusions are determined by the severity of the patient’s performance status, edemas due to lack of proteins or hypoalbuminemia. Reinfusion of plasmic effusion part to ten patients was totally satisfactory, and no side effect was noted. For reinfusion purposes, plasmic part was additionally centrifuged at 6,000 r/min during 30 min in order to eliminate cellular fractions, and after that it was carefully examined in cytological, bacterial, and biochemical tests and then reinfused intravenously to the patients.
In some cases along with immunologic pleurodesis, there were registered decreased indexes of tumor markers and reduced size and density of metastatically modified supraclavicular lymph nodes. Elimination of effusion accumulation opens a new opportunity to treatment that was started before effusion onset: One patient had a successful radiation therapy and fifteen patients underwent chemotherapy due to non-small-cell lung cancer. Other patients had a dynamic follow-up during 2 months to 2 years. Course of disease within this period demonstrated other symptoms of cancer process, including disease progression but free from malignant effusion.
Analysis of autologous LAK immunophenotype showed that after cultivation of lymphocytes derived from effusion during 3–5 days in the presence of IL-2, the number of СD4+/СD25+ cells may increase, which may occur due to lymphocyte transformation into activated cells triggered by IL-2. Infusion of high doses of IL-2 can also stimulate functions of natural subpopulation of regulatory CD4+/CD25+/Foxp3+ T cells (T-reg), which play their role in immunologic tolerance and suppress antitumor activity of NK and T cells [40, 41].
Our data showed no increase of CD4+/CD25+/Foxp3+ Т-reg in LAK population even during long-term incubation of peripheral blood lymphocytes of healthy donors or cancer patients in the presence of IL-2. Only if generating LAKs from lymphocytes of the pleural effusion with enhanced initial T-reg subset the number of suppressive T-reg subpopulation might increase [42].
2.5 Autologic Vaccines on the Base of Dendritic Cells (DC Vaccines)
Dendritic cells (DCs) are the antigen-presenting cells (APC) with a unique ability to induce primary immune response. DCs both prime naive cytotoxic T cells and activate memory cells thus playing an important role in adaptive immunity.
Mature DCs for antitumor vaccines are typically generated from CD14+ monocytes according to a well-known two-stage methodology. The initial stage is cultivation for 6–7 days in the presence of granulocyte-macrophage colony-stimulating factor and IL-4 in macrophage-conditioned medium [43].
The second stage – DC maturation – may proceed in the presence of various factors, such as bacteria (live or dead), bacterial products, lipopolysaccharide, viruses, two-strand RNA or its analog poly-I:C, proinflammatory factors and their combinations (IL-1β, tumor necrosis factor-α, IL-6, prostaglandin Е2 [PGE2]), and СD40 ligand (CD40L). During maturation, DCs lose their ability for endocytosis and antigen processing [43, 44]. Early studies on the use of DCs involved only small groups of patients, but reported potentially promising results [45, 46].
To date, over 200 clinical trials have assessed DC-based vaccines, yet their clinical effectiveness and expedience for the use in cancer patients become more and more doubtful. Rosenberg SA et al. argued that early optimism for DC vaccines relied rather on dubious surrogate end points, which lacked robustness, than on evidence-based proof of antitumor effects. One trial, conducted at the Surgery Branch of the National Cancer Institute on 440 patients, yielded an overall objective response rate of only 2.6 %. This was comparable to the 4.0 % response rate reported in 40 other smaller studies involving a total of 756 patients [47]. More recent studies showed partial or complete regression rates of 4.0–12 % in patients with advanced cancer [48].
2.6 Advantages of Combined Implication of DC Vaccines and Activated Lymphocytes
Experimental studies in vitro showed that co-incubation of DCs and activated lymphocytes results in enhanced antigen-presenting function of DCs and increased cytotoxic lymphocyte activity [49, 50]. When DCs pulsed by tumor lysate (TL) are cultured with activated lymphocytes, they can induce a specific and strong immune response against renal carcinoma cells (RCC) and prostate cancer cells [51]. On the basis of their initial in vitro experiments, other authors planned and conducted a randomized controlled trial to evaluate the efficacy of adjuvant immunotherapy with autologous TL-pulsed DCs co-cultured with CIK cells for treating cancer patients. The described cell culture was used for immunotherapy against localized and locally advanced RCC. The authors mentioned that nearly 20–40 % of patients with clinically localized RCC will develop metastases after nephrectomy or nephron-sparing surgery [52]; therefore such patients need effective adjuvant therapy. A recent randomized controlled trial of adjuvant combined immunotherapy by TL-DC-CIK cells showed that all patients tolerated the TL-pulsed DC-CIK cells immunotherapy very well, and side effects in the DC-CIK group were less than in the IFN-α group. The metastasis and recurrence rates were significantly decreased after TL-pulsed DC-CIK cells or IFN-α immunotherapy compared with the control group [53]. Effectiveness of TL-DC-CIK cells immunotherapy was shown in combination with chemotherapy in patients with breast cancer, advanced non-small-cell lung cancer, and multiple myeloma [54, 55]. There are ongoing clinical studies on evaluation of the effectiveness of TL-DC-CIK cell immunotherapy in patients with hepatocellular and pancreatic carcinomas [56, 57]. The authors consider combined DC-CIK cell immunotherapy as a novel strategy for treatment of cancer patients which improves effectiveness of antitumor vaccines and activated lymphocytes.
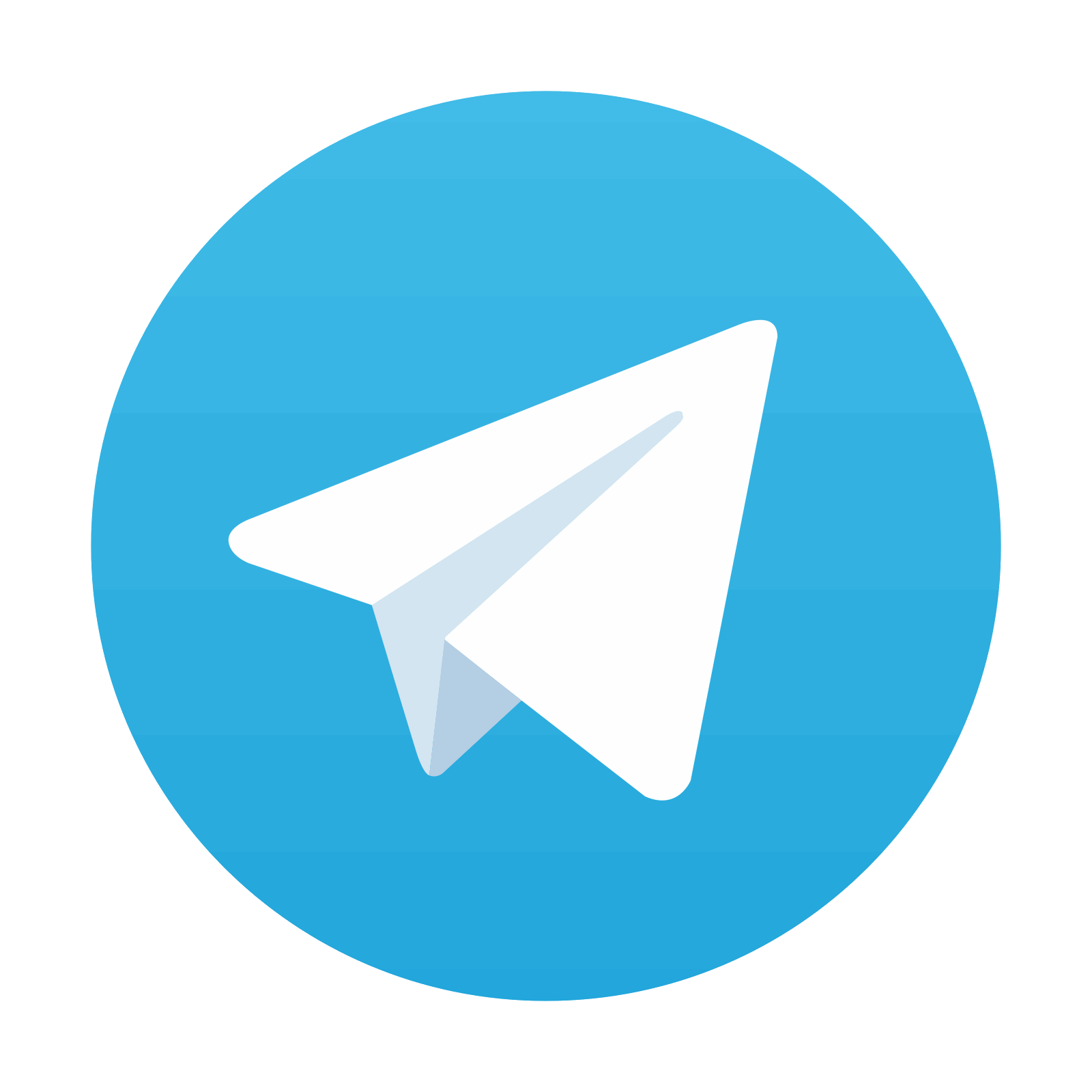
Stay updated, free articles. Join our Telegram channel

Full access? Get Clinical Tree
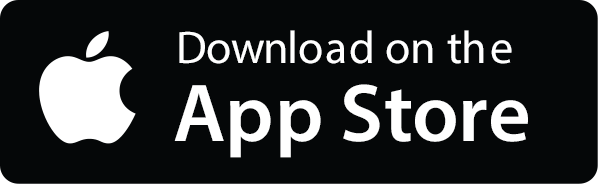
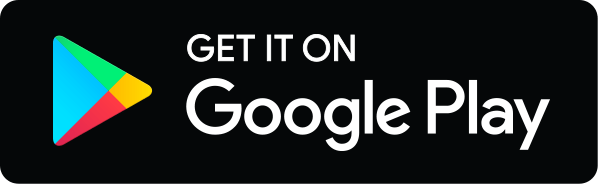