Fig. 16.1
NK cell-based therapies. (a). NK cells are purified from the peripheral blood of a healthy donor and activated in vitro with cytokines (IL-2 or IL-15) before being injected into the patient. The best responses are obtained when the donor does not express KIRs that recognize the patient’s HLA molecules, so that infused NK cells do not receive inhibitory signals from cancer cells. (b). NK cells are purified from the peripheral blood of the patient and genetically modified to express a chimeric receptor specific for a tumor antigen (CAR) or other molecules able to direct NK cells more efficiently against their targets. (c). Immune stimulatory cytokines are administered to patients to induce the activation and expansion of autologous NK cells. The cytokine that induces the strongest antitumor response is IL-2 but also causes significant side effects, such as regulatory T-cell (Treg) expansion and tissue inflammation that make it difficult to use
16.1.2 Gene Modification of NK Cells to Improve the Efficacy of Adoptive Immunotherapy
New gene therapy methods to enhance NK cell function against tumor cells have been developed and are under investigation in preclinical and clinical trials. A promising approach aims to enhance NK cell tumor specificity using chimeric antigen receptors (CARs) (Fig. 16.1b). Various preclinical studies are underway to explore the use of CAR-NK cells expressing a receptor specific for CD19 or CD20 in B-cell pathologies [23, 24]. Alternatively, NK cells retrovirally transduced to express NKG2D or TRAIL revealed improved tumor recognition and killing in preclinical studies [25, 26]. Another strategy is to protect NK cells from the immunosuppressive effect of TGF-β present in the tumor microenvironment using a dominant negative receptor II for TGF-β [27] or to improve the survival of NK cells in vivo by forcing the expression of IL-2 or IL-15 [28–30].
16.1.3 Infusion of Cytokines
Clinical studies are currently underway to test the efficacy and side effects of cytokines such as IL-2, IL-15, and IL-12 in several types of cancer (Fig. 16.1c). In an early study by Rosenberg et al., the combination of lymphokine-activated killer cells (LAK cells) and IL-2 resulted in a 20% response rate in patients with melanoma or metastatic renal cell carcinoma [31]. However, the major problem of this approach is the toxicity determined by an exacerbated activation of the immune system in a nonspecific way that leads to neuropathy, capillary leak syndrome, and renal failure. IL-15 represents an attractive alternative to IL-2 and has already been investigated in patients with metastatic melanoma and metastatic renal cell cancer [32].
16.1.4 Infusion of Tumor Antigen-Specific Antibodies to Induce NK Cell ADCC
A number of clinically approved therapeutic antibodies targeting tumor-associated antigens (such as rituximab or cetuximab) function at least partially by the initiation of NK-mediated ADCC [33, 34] (Fig. 16.2a). In order to improve the affinity of the activating receptor FcγRIIIA for the Fc region of IgG, different approaches have been tried, including insertion of mutations or glycosylation. This resulted recently in the approval of mogamulizumab [35], a low-fucose anti-CCR4 mAb for the treatment of T-cell lymphomas, and obinutuzumab [36], an anti-CD20 mAb for the treatment of chronic lymphocytic leukemia (CLL).


Fig. 16.2
Infusion of NK cell-specific mAbs. (a). Monoclonal antibodies directed against tumor antigens can also bind to the FcγRIIIA receptor expressed by NK cells and induce NK-mediated ADCC. (b). Infusion of mAbs directed against inhibitory NK cell receptors blocks the interactions with their ligands and allows NK cells to efficiently kill target cells
16.1.5 Infusion of Antibodies Directed Against Inhibitory NK Cell Receptors
The aim of this approach is to increase the reactivity of autologous NK cells by treating patients with mAbs that block the engagement of the inhibitory NK receptors (Fig. 16.2b). In the case of unmodified mAbs (such as rituximab), cytotoxicity mediated by NK cells is still under the control of inhibitory receptors [37]. By using antibodies that block the interaction of certain inhibitory receptors with their ligands, it is possible to potentiate the antitumor NK cell response in vivo. Although there is no truly specific molecule of NK cells, some surface receptors are mainly expressed by NK cells and play a major role in regulating their function [38]. These molecules activate negative signaling pathways and function as immune checkpoints in the control of cytotoxicity. In the next paragraph, we focus our attention on this approach as it represents a real revolution in cancer therapy.
16.2 Immune Checkpoint Inhibitors (ICI)
The role of the immune system in cancer patients has not been appreciated for several decades because tumors effectively suppress immune responses by activating pathways that normally regulate immune homeostasis. Immune checkpoint inhibitors (ICI), such as blocking antibodies, have been shown to be effective and to have manageable safety profiles for several cancer types (Fig. 16.3). Their use has resulted in long-term survival, for more than a decade, in some patients with cancers for which significant therapeutic advances have been made, such as metastatic melanoma and NSCLC. The identification of inhibitory pathways of immune responses has thus been a seminal series of discoveries leading to a tremendous increase in our understanding and the manipulation of immunity.


Fig. 16.3
Receptor/ligand inhibiting interactions at the NK/target cell interface. Several inhibitory receptors and co-receptors cooperate in the regulation of NK cell function. Combinations of blocking mAbs, defined on the basis of patient- and tumor-specific features, may allow to release the brake on NK cell activation
The majority of the inhibitory receptors contain one or more ITIM in their cytoplasmic domain which are phosphorylated and recruit protein tyrosine phosphatase (SHP-1/2 or SHIP). A bioinformatics research across the entire genome revealed the existence of more than 300 type I and type II integral membrane proteins that contain at least one ITIM domain [39]. Of these receptors, only a few are targeted in therapeutic approaches (Table 16.1).
Table 16.1
NK cell receptor/ligand targeting drugs in clinical development
Lymphocyte receptor | Tumor ligand | Drug | Status |
---|---|---|---|
KIR2DL1/2/3 | HLA-C | Lirilumab | Phase II |
NKG2A | HLA-E | Monalizumab | Phase II |
PD-1 | PD-L1/−L2 | BMS/ONO: nivolumab | Marketed (Opdivo) |
Merck: pembrolizumab | Marketed (Keytruda) | ||
Regeneron/Sanofi: REGN2810 | Phase II | ||
Novartis: PDR001 | Phase II | ||
AZN: MEDI0680 | Phase I/II | ||
Pfizer: PF-06801591 | Phase I | ||
Agenus/Incyte: INCSHR1210 | Phase I | ||
Janssen: JNJ-63723283 | Phase I | ||
PD-L1 | PD-1 | Roche: atezolizumab | Marketed (Tecentriq) |
AZN: durvalumab | Approved (Imfinzi) | ||
Pfizer/Merck KGa: avelumab | Approved (Bavencio) | ||
LAG3 | HLA-II | BMS: BMS-986016 | Phase II |
Novartis: LAG525 | Phase I | ||
Anaptys/Tesaro | Preclinical | ||
Merck | Preclinical | ||
Regeneron/Sanofi | Preclinical | ||
Macrogenics (PD-1 bispecific) | Preclinical | ||
IMP321 | Phase I/II | ||
Tim-3 | Gal-9 | Novartis: MBG453 | Phase I |
CEACAM1 | Anaptys/Tesaro: TS-022 | Phase I | |
Phosphatidylserine | Jounce/Celgene | Preclinical | |
LILRB2 | Roche | Preclinical | |
TIGIT | CD155, CD112 | Roche | Phase I |
Merck | Preclinical | ||
BMS | Preclinical | ||
VISTA | Unknown | Janssen: JNJ-61610588 | Phase I |
Igenica: IGN381 | Preclinical | ||
Unknown | B7-H3 | Enoblituzumab | Phase I |
16.2.1 KIRs
KIR2DL1, KIR2DL2, and KIR2DL3 are the inhibitory receptors that, upon binding to HLA-C on target cells, are involved in the control of NK cell cytotoxicity and cytokine production [40]. Evidence of the role of KIR receptors in the NK cell antitumor response was formally demonstrated in patients with acute myeloid leukemia (AML) undergoing haploidentical bone marrow transplantation [41]. Indeed, the subgroup of patients that received KIR/HLA-C mismatched bone marrow transplant had significantly lower rate of relapse without GVHD, which translated in follow-up studies into significantly better survival [42], suggesting that donor-derived alloreactive NK cells were capable of mediating safe and durable antitumor immunity. In order to mimic this strategy with a drug, a therapeutic mAb blocking the three inhibitory KIR receptors for HLA-C was generated: 1-7F9 is a fully human IgG4 that increases NK cell cytotoxicity against HLA-C-expressing tumor cells. This was demonstrated in vitro, by using patient-derived AML blasts targeted by autologous or heterologous NK cells and confirmed in a humanized AML mouse model [43]. The efficacy of this approach was also validated in a syngeneic tumor model where the blocking of Ly49C/I (the homologous receptor for KIR in the mouse) with a specific mAb could induce NK cells to selectively reject tumor cells while sparing normal tissues [44]. This translated in the clinical development of a hybridoma-derived product, IPH2101. It was tested in several phase I clinical trials [45–48] showing that KIR blockade is safe, with minimal side effects, deserving of further clinical investigations. Then, lirilumab (IPH2102, BMS-986015), a stabilized recombinant IgG4, with the same antigen specificity, was developed to increase stability and manufacturability of the mAb. It is currently tested in multiple phase I and II trials: as maintenance monotherapy in elderly AML patients (NCT01687387) or in combination with tumor-targeting mAb elotuzumab in multiple myeloma (NCT02252263) or DNA hypomethylating agent 5-azacytidine in AML (NCT02399917) and myelodysplastic syndromes (NCT02599649) or rituximab in CLL (NCT02481297). Interestingly, in vitro and in vivo studies showed that lirilumab increases the rituximab-induced ADCC [49].
Recently, a phase II clinical study that tested the efficacy of lirilumab as monotherapy in patients with multiple myeloma was prematurely discontinued due to lack of efficacy [50]. According to the authors, this could be due to a reduction of NK responsiveness that selectively affected KIR2D+ cells, accompanied by a loss of KIR2D expression on the cell surface. However, this contraction has not been detected in other clinical trials, and this data needs to be confirmed. These results may suggest the requirement, at least in some malignancies, of adapted therapeutic regimens (intermittent blocking) or combined therapy in order to induce an effective antitumor response. For example, given the clinical success of anti-PD-1 blocking mAbs and their ability to induce immune-stimulating cytokines like IFNγ that may boost NK cells, lirilumab is also explored in association with nivolumab in order to combine the functional restoration of both NK and T cells, in solid tumors (NCT01714739) and hematological malignancies (NCT01592370). In two phase I clinical trials in patients with advanced solid tumors, this combination showed no additional toxicity compared to nivolumab monotherapy (Segal N.H. et al., unpublished). More recently, a phase I/II trial has shown the efficacy of the treatment with lirilumab in combination with nivolumab in patients with advanced and chemically resistant head and neck carcinoma (Leidner R. et al., unpublished). This was a single-arm study, so there was no control population, but there are well-established historical control data for head and neck cancers to judge the outcomes. The study revealed that lirilumab was well tolerated for the head and neck cancer population. Although the follow-up and maturation of the data are ongoing, what was observed from an efficacy point of view was encouraging, but the results have to be understood in the context of a single-arm, fairly small study. The overall survival was 90 and 60% at 6 and 12 months, respectively, for patients treated with the lirilumab and nivolumab combination therapy as compared to 55.6 and 36% for nivolumab alone which has been recently FDA approved for this indication. These preliminary clinical data suggest a very exciting tail of the curve for head and neck cancer patients in a way analogous to what has been reported in other cancer indications, such as melanoma treated with a PD-1 or CTLA-4 immune checkpoint inhibitors. An in-depth evaluation of the immuno-correlative biomarkers would be very useful.
The results of a randomized, double-blind, placebo-controlled phase II clinical trial (EffiKIR) evaluating the efficacy of lirilumab as a single agent in elderly patients with AML have been recently released (NCT01687387). Although the study did not meet the primary efficacy endpoint, it confirmed the safety profile of lirilumab as a monotherapy. In more details, two arms of the trial tested single agent lirilumab at different doses and treatment intervals (0.1 mg/kg every 3 months or 1 mg/kg every 4 weeks), whereas in the third arm, patients received placebo. There was no statistically significant difference between either lirilumab arms and the placebo arm on the leukemia-free survival (LFS) or on other efficacy endpoints. The 1 mg/kg q 1month arm of the trial was discontinued as the objective of achieving a superior LFS in this arm compared to placebo could not be reached. There was no concern with tolerance. Data analyses are ongoing and will complete the understanding of the results of this trial.
Lirilumab is still being investigated in six trials, across a range of solid and hematological cancer indications in combination with other agents, including nivolumab (see on clinicaltrials.gov).
16.2.2 NKG2A
NKG2A is an inhibitory receptor expressed by the majority of NK cells and part of cytotoxic T lymphocytes which recognizes HLA-E molecules in humans and Qa-1 in mice. Unlike classical HLA class I molecules, HLA-E expression is retained, or even increased, in 50–80% of patients with solid tumors or leukemias/lymphomas [6, 47, 51, 52]. A study in NSCLC showed that infiltrated NK cells had low expression of activating receptors and KIRs, while the expression of NKG2A was not affected by tumor microenvironment [6]. At the same time, HLA-E was highly expressed on tumor cells and negative on surrounding epithelial cells. The expression of NKG2A on tumor-infiltrated NK and T cells has also been confirmed in breast and cervical cancer and appears to be related to IL-15 and TGF-β secreted by tumor cells [51, 53]. A study in melanoma patients demonstrated that the effector functions of tumor antigen-specific CD8+ T cells that expressed NKG2A were inhibited by this receptor [54]. Accordingly, tumor-infiltrating CD8+ T cells were a favorable prognostic factor in NSCLC only in patients whose tumors maintain the expression of classical HLA class I molecules and do not express HLA-E [52]. Moreover, increased HLA-E expression (documented in 20% of patients with colorectal carcinoma) was associated with a poor clinical outcome in the presence of massive CD8+ T-cell infiltration [55].
All of these results suggest that NKG2A is an important checkpoint to block in order to increase the antitumor immune response, acting directly on the infiltrated lymphocytes. In a preclinical trial, a humanized mAb specific for NKG2A (Z270) showed its efficacy in releasing the cytotoxicity of NK cells against leukemia and lymphoma cells, both in vitro and in vivo [56]. This antibody was then developed for its clinical use under the name monalizumab (IPH). It is currently used in phase II clinical trials as a single agent for the therapy of gynecologic malignancies (NCT02459301) and in the preoperative setting in squamous cell carcinoma of the oral cavity (NCT02331875) or in combination therapy with cetuximab (an anti-EGFR mAb) in head and neck cancer (NCT02643550), with ibrutinib (a BTK inhibitor) in CLL (NCT02557516), and with durvalumab (an anti-PD-L1 mAb) in various solid tumors (NCT02671435).
16.2.3 PD1
PD-1 is an inhibitory receptor that binds PD-L1 and PD-L2, its specific ligands expressed on several tumor or infected cells but also by professional antigen-presenting cells (APC) present in inflammatory foci. Initially described on T, B, and myeloid cells [57], more recent papers reported the expression of PD-1 on NK cells from patients affected by multiple myeloma and ovarian carcinoma [58–61]. Studies in vitro demonstrated that the engagement of PD-L1 expressed by tumor cells can inhibit their lysis by NK cells, while PD-1 blocking mAbs restore NK cell function and favor the migration of NK cells toward the tumor site. During the last few years, several antibodies blocking PD1/PD-L1 interactions have been developed (Table 16.1) and are currently employed for treatment of advanced solid tumors. Among them, nivolumab has been shown to induce in T lymphocytes the expression of genes typically involved in cytotoxicity and NK cell function, such as IFNγ and granzyme B [62]. These data suggest that blocking this checkpoint can rescue the antitumor immune response by acting on the same molecular pathway in both T and NK cells. Although the blocking of PD-1 has long been known to reestablish an antitumor response in mice [63, 64], clinical trials now provide important results in cancer patients. Clinical responses to monotherapy have been observed in a wide range of solid and hematologic cancers [65, 66]. Importantly, the responses are often durable and without serious toxicity in most people. Nevertheless, only a minority of people treated with antibodies specific for PD-1 or PD-L1 have a strong response, with a rapid rate of reduction of the tumor ranging from 10 to 40%, depending on the patient [66]. In order to explain this variability, various tumor biopsies collected from patients prior to treatment were examined from a histological point of view, and their composition was related to the grade of response observed in patients. The results showed that it is possible to distinguish three immune profiles that are correlated with the efficacy of anti-PD-L1/PD-1 therapy [65]. The first profile, the “inflamed” phenotype, is characterized by the presence in the tumor parenchyma of numerous immune cells positioned in proximity to the tumor cells. In these samples, the infiltrated immune cells may exhibit staining for PD-L1. This profile suggests the presence of a preexisting antitumor immune response, which may have been stopped by immunosuppression in the tumor. Indeed, clinical responses to anti-PD-L1/PD-1 therapy occur most often in patients with inflamed tumors. The second profile is the “immune-excluded” phenotype, which is also characterized by the presence of immune cells, but they are retained in the stroma that surrounds the tumor cells and do not penetrate the parenchyma. The third profile, the “immune-desert” phenotype, is characterized by a shortage of hematopoietic cells in either the parenchyma or the stroma of the tumor. This phenotype probably reflects the absence of preexisting antitumor immunity, and it is not surprising that these tumors rarely respond to anti-PD-L1/PD-1 therapy [67]. In contrast, not all tumors characterized by an immune-inflamed profile respond to anti-PD-L1/PD-1 treatment. In these cases, probably other immune checkpoints are responsible for inhibiting the antitumor response [68].
16.2.4 LAG3
Lymphocyte-activation gene 3, also known as LAG3, is a cell surface molecule that belongs to immunoglobulin superfamily, expressed by activated T lymphocytes, NK cells, B cells, and plasmacytoid dendritic cells, with diverse biologic effects. LAG3 is a ligand for MHC class II molecules, to which it binds to very high affinity. It is an immune checkpoint receptor that negatively regulates cellular proliferation, activation, and homeostasis of T cells and has been reported to play a role in Treg suppressive function [69]. Early after its discovery, experiments in LAG3-deficient mice have suggested that LAG3 was crucial for NK cell function [70]. But, since LAG3 was also found to be expressed on tumor-infiltrating CD8+ T cells and to inhibit their proliferation and IFNγ production [71], thereafter, studies have been mostly focused on its role in regulating adaptive immunity. LAG3 is now the target of various drug development programs by pharmaceutical companies seeking to develop new treatments for cancer and autoimmune disorders. The predicted mechanism of action for LAG3-specific mAbs is to release the negative regulation of NK and T cells. MHC class II molecules are generally expressed only by APCs, but some cancer cells have been reported to express them as well. LAG3 interaction with MHC class II molecules expressed by melanoma cells has been shown to protect them from FAS-mediated apoptosis [72]. LAG3 also encodes an alternative splice variant that is translated to a soluble form of LAG3 (sLAG3), which exhibits immune adjuvant activity [73]. Although sLAG3 is not the intended target of clinical trials using LAG3-specific mAbs, it may be informative to bear in mind its role. Interestingly, sLAG3 is thought to bind only to MHC class II molecules present in lipid raft micro-domains on a minor subset of APC. Interestingly, a clinical-grade soluble form of LAG3 protein (LAG3-Ig fusion protein, IMP321), a physiological high-affinity MHC class II binder, has been shown to induce IFNγ and TNF production by NK cells and CD8+ T cells in short-term ex vivo assays [74]. IMP321 is currently employed as immune-stimulating drug in phase I and phase II clinical trials, as adjunctive to standard chemotherapy or in combination to anti-PD-1 therapy, respectively. BMS-986016, an anti-LAG3 mAb, is currently in phase II clinical testing. A number of additional LAG3 antibodies are in preclinical development. LAG3 may be a better checkpoint inhibitor target than PD-1 since it can both activate T and NK effector cells and inhibit Treg suppressive activity [75]. A therapy targeting LAG3 in combination with nivolumab (NCT01968109) is currently investigated in subjects with select advanced (metastatic and/or unresectable) solid tumors.
16.2.5 Tim-3
Tim-3 is a type I glycoprotein expressed by innate and adaptive immune cells. It was first identified as a molecule selectively expressed on IFNγ–producing CD4+ T helper 1 (Th1) and CD8+ cytotoxic T cells. Tim-3 is also constitutively expressed by functional and mature NK cells. Upon activation, it functions as an inhibitory receptor on NK and T cells by reducing cytotoxicity and cytokine production [76, 77]. Tim-3 is also found on T cells in patients with advanced melanoma, NSCLC, and follicular B-cell non-Hodgkin lymphoma. In these three cancers, all Tim-3+ T cells co-expressed PD-1 and exhibited defects in proliferation and cytokine production [78]. A recent study showed that Tim-3 expression was increased on circulating NK cells in advanced melanoma patients and that this correlated with their exhausted phenotype [79]. Accordingly, blockade of Tim-3 signaling with mAbs resulted in the increased cytotoxicity and IFNγ production of peripheral NK cells from patients with lung adenocarcinoma [80]. Thus, Tim-3 expression in NK cells can function as a prognostic biomarker in human malignancies. Anti-Tim-3 blocking mAbs are now tested in phase I clinical trials, and upcoming evidences suggested their use in combination with anti-PD-1 therapy. Indeed, in the setting of anti-PD-1 therapy, treatment failure is associated with upregulation of alternative immune checkpoints (most notably Tim-3), while resistance to anti-PD-1 therapy was prevented when an anti-Tim-3 mAb was administered together with an anti-PD-1 agent [68].
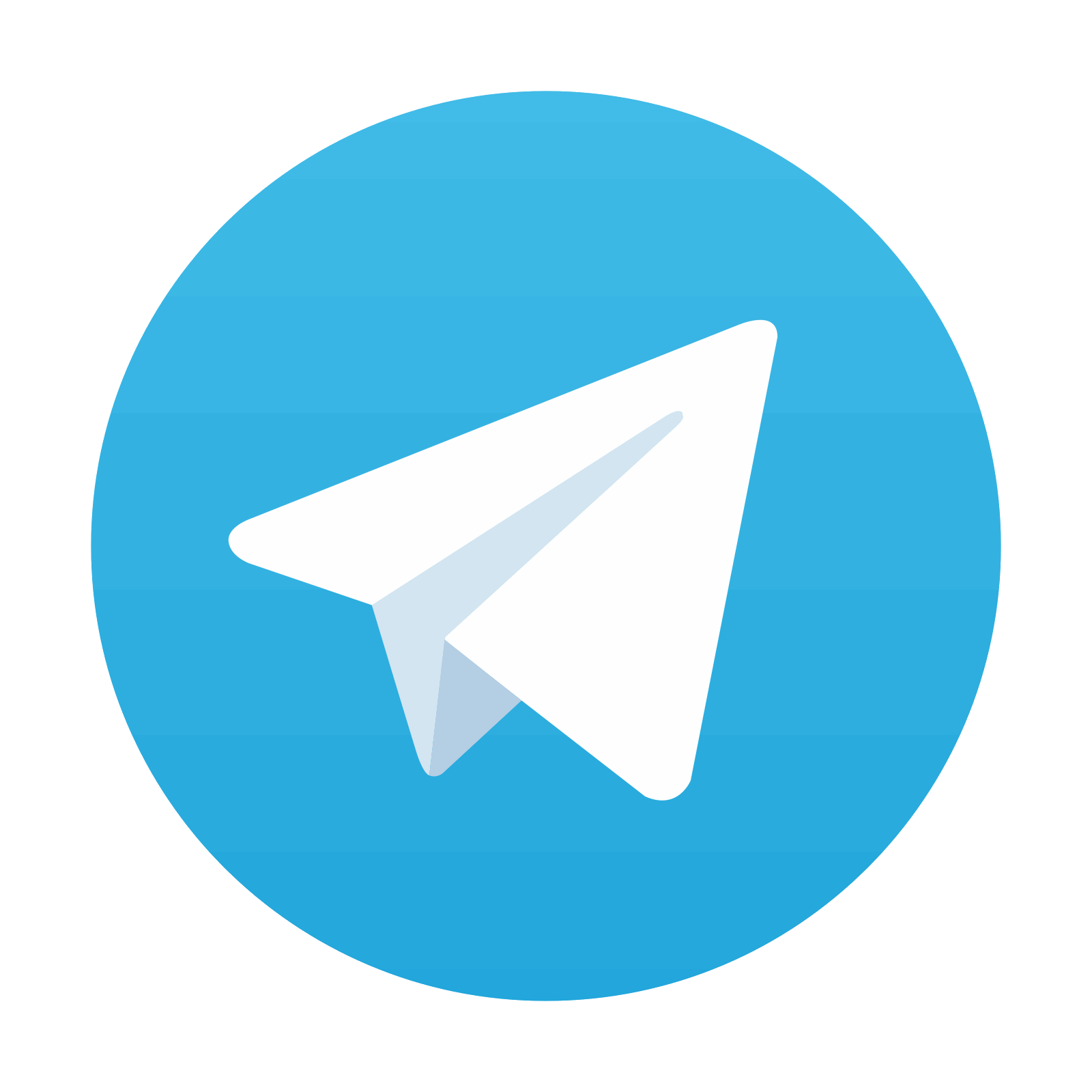
Stay updated, free articles. Join our Telegram channel

Full access? Get Clinical Tree
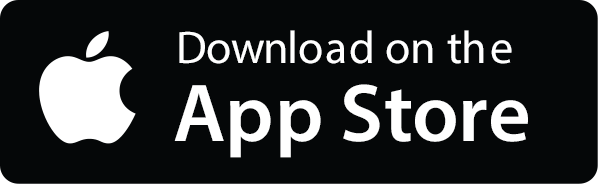
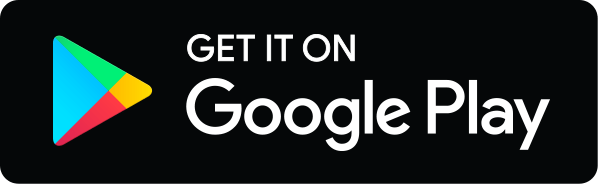