Roberta Diaz Brinton
Neuroendocrinology of Aging
Neuroendocrine aging is a multifactorial process that typically spans years and is characterized by sequential phase transitions.1 These transitions are progressive in nature and are typified by stages of system-level changes in function, followed by compensatory adaptations. Furthermore, phase transitions are typically nonlinear processes that are more consistent with a step function from one state to another. Transition states are separated by intervening periods of apparent stability, during which the neuroendocrine system undergoes systematic dismantling and is often followed by the activation of compensatory adaptive responses. Adding to the complexity is individual variability derived from genetic, environmental, and experiential factors that alone and in concert can influence the rate of neuroendocrine aging.
Neuroendocrine aging is an illustrative example of the integrated coordination of chronologic and endocrine aging programs.2 Both these programs of aging are modifiable by endogenous and exogenous modifiers (see Chapter 23).3 Increasingly, the aging of neuroendocrine systems is recognized as a fundamental modifier of chronologic aging. For example, the development of insulin resistance at any age adversely modifies the trajectory of chronologic aging in women and men. This brief review focuses on two illustrative broad and interrelated aspects of neuroendocrine aging, reproductive senescence and metabolic dysfunction in women and men. Aging of both these neuroendocrine systems provides specific examples of fundamental principles of neuroendocrine aging in women and men, including phase transitions, adaptive compensatory responses, and biologic resilience.
Neuroendocrine Aging in Women
Typically, the first indication of neuroendocrine aging in women is the transition to reproductive senescence, which usually begins during the mid-40s and ends in the early to mid-50s.4,5 For some women, however, reproductive senescence can commence during the third decade of life.4 The female reproductive axis is comprised of the hypothalamic-pituitary-ovarian-uterine axis and undergoes accelerated aging relative to other systems, which are otherwise healthy.1,5
Reproductive senescence in women is defined by oocyte depletion, which begins at birth and proceeds as a continuum until menopause has been undergone. A woman is endowed at birth with a finite number of oocytes that are arrested in prophase I of meiosis. Reproductive aging consists of a steady loss of oocytes through ovarian follicular atresia or ovulation, which does not necessarily occur at a constant rate.6 The relatively wide age range for menopause in normal women (42 to 58 years) seems to indicate that women are endowed with a highly variable number of oocytes or the rate of oocyte loss varies greatly.1 Menopause occurs at an average age of 51.4 years, with a Gaussian distribution from 40 to 58 years.1 In the United States, approximately 1.5 million women reach menopause each year, and more than 45 million women will be older than 55 years in 2020.7
Perimenopause and Postmenopause
Perimenopause
Characteristics inherent to the perimenopausal transition have been extensively documented and chronicled.4,5 The Stages of Reproductive Aging Workshop (STRAW) criteria,4 an international collaborative effort, along with the Study of Women Across the Nation (SWAN), based on an ethnically diverse U.S. population,8 have contributed to the classification of the perimenopausal transition while simultaneously identifying the complexity of symptoms and ethnic diversity of perimenopausal phenotypes. Normal reproductive aging in women is characterized by three distinct phases that can span years—perimenopause (also known as the menopause transition), menopause, and postmenopause.5 According to STRAW, reproductive senescence can be subdivided into three distinct stages—perimenopause, menopause, and postmenopause.4 Variable cycle length, variable intervals between cycles, vasomotor symptoms (hot flushes), and wide variations in steroid hormone levels characterize the menopausal transition.4 One year of amenorrhea is considered to be the end of the perimenopause stage and onset of menopause. The end of the amenorrheic year commences the onset of the postmenopausal stage, which is also subdivided into early and late components. Early postmenopause is defined as 4 years since the last menstrual period and is followed by late-stage postmenopause, which thereafter defines the neuroendocrine state.4,5
Perimenopause is characterized by menstrual and endocrine changes in the hypothalamic-pituitary-ovarian-uterine axis that lead inexorably to reproductive senescence.1 During perimenopause, ovulation occurs irregularly as a result of fluctuations in hypothalamic and pituitary hormones.4,5 In late-phase perimenopause, nearly half of the cycles are anovulatory.4,5 In the ovulatory cycles, follicle-stimulating hormone (FSH), luteinizing hormone (LH), and 17β-estradiol levels increase with progression of the stage of reproductive aging and, in the luteal phase, the serum progesterone level is decreased. The early-cycle (ovulatory and anovulatory) inhibin B level decreases steadily across the STRAW stages and is largely undetectable during elongated ovulatory and anovulatory cycles during the transition. The decline of inhibin B levels during early perimenopause results in an increase in follicle-stimulating hormone (FSH) levels, with no significant change in inhibin A or estradiol levels.4 FSH levels may rise during some cycles but return to premenopausal levels in subsequent cycles.1 Further complicating the determination of FSH concentration is the pulsatile pattern of secretion. The variability in hormone levels creates difficulties in interpreting a single laboratory test result9 but an increase in FSH levels continues to be a clinical marker of ensuing menopause and postmenopause.4
In late-stage perimenopause, concentrations of 17β-estradiol are highly variable; levels can be persistently low, as might be expected, but can also be abnormally high.1,4 A high 17β-estradiol concentration is associated with increased vulnerability to neurodegenerative insults and neuronal cell death,10 but does not reduce the persistently high FSH and LH levels.11,12 Remarkably, the cyclicity of progesterone appears to remain generally intact, whereas the level of progesterone can vary from a normal value to a high spike of progesterone to an undetectable level.13 When considering relationship between steroid exposure and neurologic symptoms of menopausal transition, plasma levels of ovarian hormones are not predictive of brain concentrations of steroids.2
The hallmark symptom of the menopausal transition is the hot flush, also referred to as hot flashes. Although the hot flush is evidenced by vasodilation, the signal to vasodilate is neurologic.1 The neural mechanisms underlying the signature symptom of menopause remain unclear. Hot flushes are most likely to occur during late-stage perimenopause and early-stage postmenopause.4,14 The prevalence of hot flushes increases substantially in early perimenopause, reaches a maximum in late-stage perimenopause, remains high into the early postmenopausal stage, and returns to a low but persistent prevalence in late-stage postmenopause.14–16 The prevalence of hot flushes can range from 30% to 80%, depending on ethnicity, with African American women experiencing the greatest frequency and longest duration.17 Women aged 40 to 60 years who have had a hysterectomy and oophorectomy are at high risk for hot flushes.4 In most women, hot flushes are transient. Hot flush frequency and severity abate within a few months in 30% to 50% of women and resolve in 85% to 90% of women within 4 to 5 years. However, 10% to 15% of women continue to have hot flashes into late-stage postmenopause.17
Although the mechanism underlying hot flushes remains unknown, the resemblance to heat dissipation responses has led to a focus on thermoregulation by the anterior hypothalamus. However, the exact role of estrogen in the pathogenesis of hot flushes remains unresolved. Increasingly, evidence has linked metabolic dysregulation to the occurrence of hot flashes.1,18,19 Estrogen levels do not differ substantially between postmenopausal women who have hot flushes and those who do not, but the withdrawal of estrogen can induce hot flushes in women with gonadal dysgenesis who have undergone estrogen therapy that was subsequently discontinued, suggesting that estrogen withdrawal plays a role in the cause of hot flashes.1 In SWAN, a large U.S. multicenter cohort study, higher levels of FSH were the only hormonal measure independently associated with flushing after adjustment for levels of estradiol and other hormones.5 Women undergoing pharmacologic therapy to antagonize estrogen receptor activation by selective estrogen receptor modulators (SERMs) or inhibit estrogen synthesis using 5α-reductase inhibitors for breast cancer experience a significant increase in the frequency of hot flashes.20
Postmenopause
Like perimenopause, postmenopause is separated into early and late stages. Early postmenopause is defined as 4 years since the final menstrual cycle.7 Levels of FSH continue to be high during early menopause and remain elevated throughout the late stage of postmenopause.5,7 During the early postmenopause phase, there is a significant decline in ovarian hormones to a permanently low level, which is associated with accelerated bone loss.5,21,22 Postmenopausal women undergo two phases of bone loss, whereas aging men undergo only one.22 In women, menopause initiates an accelerated phase of predominantly trabecular bone (also known as cancellous and spongy bone) loss that declines over 4 to 8 years; this is followed by a slow phase that disappears after 15 to 20 years, when severe depletion of trabecular bone stimulates counterregulatory forces that limit further loss.22 The accelerated phase results from the loss of the direct repressive effects of estrogen on bone turnover, which is mediated by estrogen receptors in osteoblasts and osteoclasts. During menopause, bone resorption increases by 90%, whereas bone formation markers increase by only 45%. In the ensuing slow phase, the rate of trabecular bone loss is reduced, but the rate of cortical bone loss can be increased.22 Bioavailable serum estrogen and testosterone levels decline in aging men, and bioavailable estrogen is the major predictor of their bone loss. Thus, both sex steroids are important for developing peak bone mass, but estrogen deficiency is the major determinant of age-related bone loss in both genders.22 Trabecular bone has low density and strength but a very high surface area and fills the inner cavity of long bones. The external layer of trabecular bone contains red bone marrow, in which hematopoiesis occurs and most of the arteries and veins of bone organs are found.
A wide range of pharmacologic agents are available to prevent and treat osteoporosis, including antiresorptive estrogen, SERMs, bisphosphonates, calcitonin, and anabolic therapies, including parathyroid hormone (PTH—PTH1-34 or PTH 1-84) and agents with an as yet undetermined mechanism of action, such as strontium ranelate.23,24 Corrections in general deficiencies in calcium, vitamin D, or both are first-line therapeutic interventions.23,24
Therapeutic Horizons
Therapeutic intervention for endocrine aging-related symptoms continues to evolve, with increased attention to timing of intervention, dose of intervention, route of administration, and treatment regimen. Adverse outcomes of hormone therapy in late-state postmenopausal women, in particular conjugated equine estrogens plus medroxyprogesterone acetate, have led to a reevaluation of its use and delineation of factors regarding efficacy versus harm.5 Hormone therapy for women has evolved to a greater extent than for men, for whom many of the same issues found with hormone therapy for women have been emerging in the clinical use of androgen therapy in men. As in women,5 the efficacy of hormone therapy for men is age-sensitive, health status–sensitive and dose-dependent, with health risks associated with dosage and duration of use.25
Multiple pharmacologic and nonpharmacologic interventions have been used to treat hot flushes, with the most common pharmacologic intervention being estrogen or hormone therapy.5,26–28 Multiple types of estrogen, doses, and routes of administration have been developed. Each formulation targets cessation of hot flashes and prevention of osteoporosis with near equal efficacy, depending on the dose. A position statement from the North American Menopause Society (NAMS) has supported the initiation of estrogen or hormone therapy around the time of menopause to treat menopause-related symptoms.29 The use of hormone therapy was supported to treat or reduce the risk of certain disorders, such as osteoporosis or fractures and hot flashes in select postmenopausal women. Analysis of the risk-benefit ratio of hormone therapy for menopausal women has indicated a favorable benefit close to menopause but decreased benefit with aging and longer time since menopause in previously untreated women.29 The attendant risks and side effects of estrogen or hormone therapy are substantial, which has led to behavioral and alternative therapies. However, there is no convincing evidence that acupuncture, yoga, Chinese herbs such as dong quai, evening primrose oil, ginseng, kava, red clover extract, or vitamin E relieve hot flushes.30–35 Clinical trials of soy phytoestrogens have been inconsistent in showing benefit, with most randomized double-blind clinical trials indicating no significant benefit for the treatment of hot flashes.36
The association between hormone therapy and an increased risk of neoplasias in women’s reproductive organs (see Chapter 86) has led to the development of SERMs in attempt to activate the beneficial effects of estrogen selectively while the reducing risks of therapy. In recent years, an increasing number of estrogen receptor ligands and novel SERMs have been identified from nature, and others have been designed and synthesized de novo in academia and the pharmaceutical industry.26–28,37 The U.S. Food and Drug Administration (FDA)–approved indications for hormone therapy in women is for the treatment of hot flushes and prevention of osteoporosis, whereas SERMS have been approved for breast cancer prevention and treatment of osteoporosis. Thus, pharmaceutical industry efforts have focused on FDA-approved indications, along with antineoplastic actions in the breast and uterus. The oldest and most studied SERM is tamoxifen (TMX), a triphenylethylene derivative, nonsteroidal, first-generation SERM.37 A minor metabolite of TMX is 4-hydroxytamoxifen (OHT), which has a shorter half-life but binds to estrogen receptors (ERs) with a binding affinity 20 to 30 times greater than that of TMX and equivalent to that of 17β-estradiol.37 Tamoxifen functions as an ER antagonist in breast, but can act as an ER agonist activity in bone, liver, and uterus.37
Since 1971, TMX has been used to treat breast cancer in premenopausal and postmenopausal women and, in 1999, TMX was recommended for use in breast cancer prevention.37 Another nonsteroidal SERM for the treatment of osteoporosis is raloxifene (RAL), a benzothiophene derivative, nonsteroidal, second-generation SERM. Similar to TMX, RAL has a mixed pharmacologic profile, acting as an ER agonist and antagonist in a tissue-specific manner. In the breast and uterus, RAL acts as a typical antiestrogen to inhibit the growth of mammary or endometrial carcinoma, whereas in nonreproductive tissues, it acts as a partial estrogen agonist to prevent bone loss and lower serum cholesterol levels, with a pharmacologic profile similar to that of 17β-estradiol in ovariectomized rats and postmenopausal women.37,38 An increasing number of novel SERMs have now been identified and developed in academia and the pharmaceutical industry.37,38 With enhanced efficacy, specificity, and antineoplastic action in the breast and uterus, novel SERMs have demonstrated more potential clinically therapeutic uses for the prevention or treatment of menopause-related symptoms, such as hot flushes and osteoporosis. Increasingly, combination therapy of a SERM plus estrogen therapy is being used to promote estrogenic action in nonreproductive organs while inhibiting proliferation in reproductive organs such as the breast, uterus, and ovaries.26–28,38,39
Unresolved Issues in Neuroendocrine Aging in Women
Currently, there has been no pharmacogenomic strategy for identifying women appropriate for hormone therapy and, if so, for determining the hormone therapy most likely to be most efficacious. Genomic strategies to date have identified ER polymorphisms associated with an increased risk of cognitive impairment in older women. However, several single-nucleotide polymorphisms (SNPs) on ERα (ESR1) and ERβ (ESR2) genes have been associated with a range of hormone-sensitive diseases, such as breast cancer and osteoporosis. ER genetic variations may also influence cognitive aging and was investigated in a cohort of 1343 women (mean age, 73.4 years) and 1184 men (mean age, 73.7 years).40 Among women, two of the ERα SNPs (reference SNP cluster identification, rs—rs8179176, rs9340799)40,41 and two of the ERβ SNPs (rs1256065, rs1256030) were associated with a likelihood of developing cognitive impairment.40 In men, one of the ERα SNPs (rs728524) and two of the ERβ SNPs (rs1255998, rs1256030) were associated with cognitive impairment. These findings suggest that ER genetic variants may play a role in cognitive aging. Tailoring hormone therapy for women and men based on their ER SNP profile remains uncharted territory.
The timing of hormone therapy intervention is of critical importance, and several studies have attempted to determine the impact of timing of hormone therapy. Evidence has supported a critical window of therapeutic opportunity42 that is related to the healthy cell bias hypothesis of estrogen action.43,44 This hypothesis predicts that estrogen therapy, if initiated at the time of perimenopause to menopause, when neurologic health is not yet comprised, will be of benefit, manifested as reduced risk for age-associated neurodegenerative diseases such as Alzheimer and Parkinson diseases.43,44
Major challenges for optimal estrogen and hormone therapy remain. Beyond the timing issue,42,43 the real and perceived risks of hormone therapy remain and were amplified by results of the Women’s Health Initiative trial and Women’s Health Initiative Memory Study.45–47 It is clear that many but not all women could potentially benefit from estrogen or hormone therapy intervention.1 Biomarkers to identify women appropriate for this type of therapy and to determine which type of hormone regimen is most appropriate remains largely undeveloped beyond the treatment of hot flashes.1 Hormone therapy interventions that selectively target the benefits of estrogen while avoiding untoward risk factors remain an unmet need in women’s health. Estrogen alternatives that activate estrogen mechanisms in the brain but not in the breast or uterus, such as NeuroSERMs and PhytoSERMs, are promising strategies for sustaining the benefits of estrogen in the brain to prevent age-associated neurodegenerative disease.44
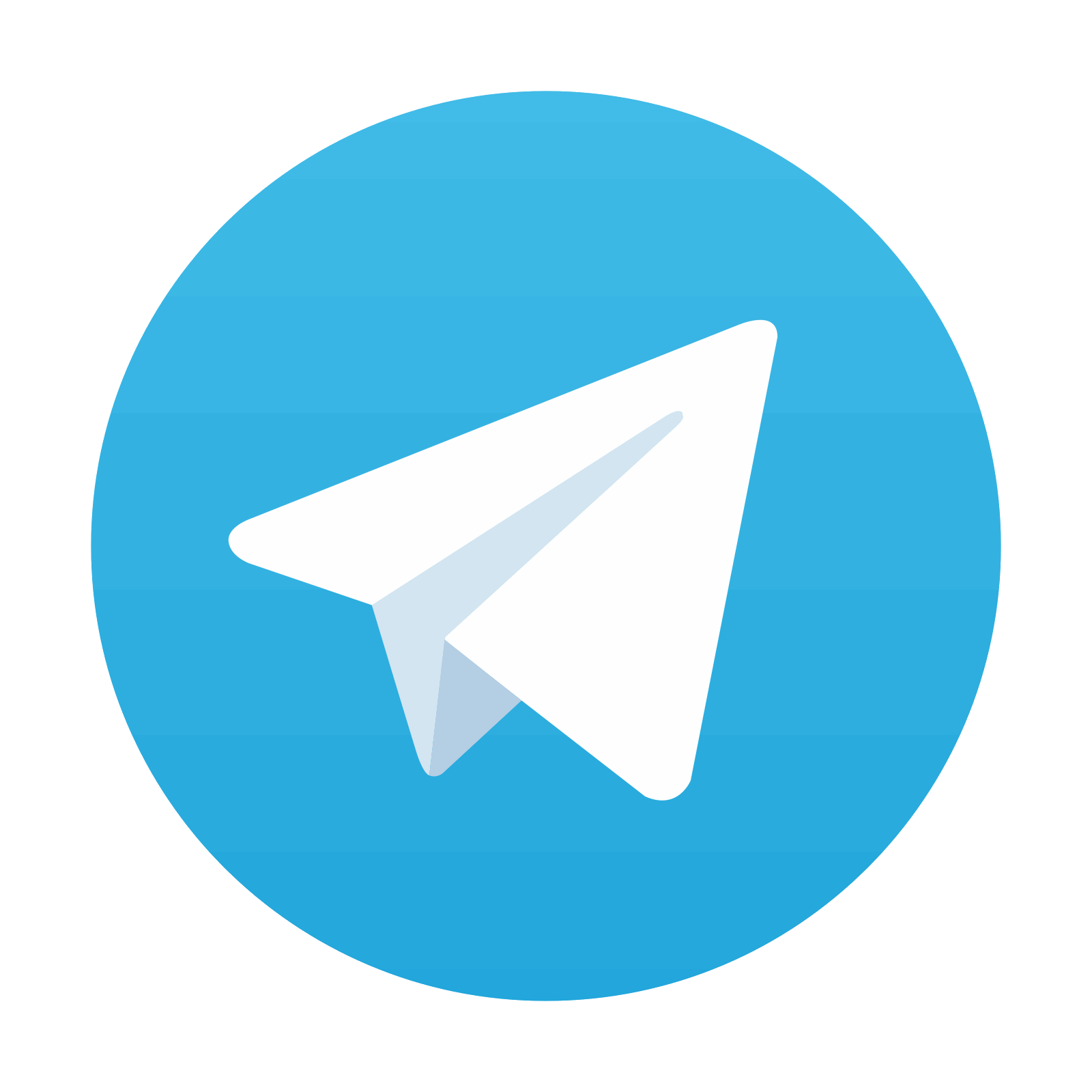
Stay updated, free articles. Join our Telegram channel

Full access? Get Clinical Tree
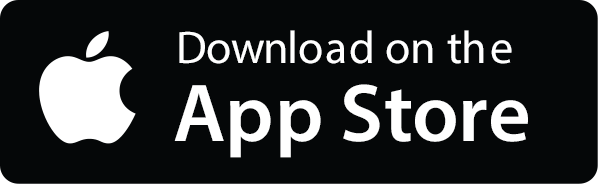
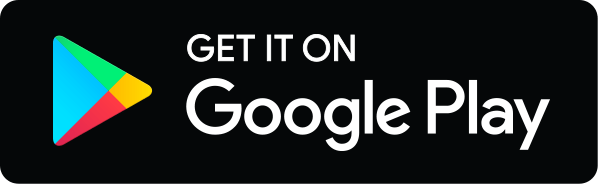