CH incidence
Québec, Canada
Milan, Italy
Albert, NZa
Castanet, France
Period
1990–2009
1993–2010
1980–1998
TSH threshold (mU/L)
15- 15
15–5
20
12–10
15
Variable over time
Global
1: 2,900
1: 2,450
1: 2,654
1: 1,446
1: 3,850
1: 3,560
Dysgenesis
1: 4,200
1: 4,510
1: 4,740
1: 4,520
1: 6,250*1
1: 5,000
Dyshormonogeneis
1: 29,300
1: 37,800
NA
NA
1: 17,000
NA
Normal gland in situ
1: 22,250
1: 9,850
NA
NA
NA
NA
4.3 Initial Diagnostic Procedure and Follow-Up
NBS is only the start of a process that should lead to diagnosis, management, and outcome evaluation of CH [14]. First, the detection of a high TSH concentration on screening should be communicated quickly to the parents, and the newborn should be referred to experienced physicians to confirm the diagnosis and to start the treatment as early as possible [26]. Initial work-up should include (1) assessment of venous TSH and free T4, (2) an X-ray of the knee to assess the severity of intrauterine hypothyroidism by the presence or absence of femoral and tibial epiphyses, and (3) either thyroid scintigraphy or echography (or both) to establish the anatomical diagnosis (i.e., ectopy, athyreosis, normal gland, or goiter). Nowadays, it is possible to perform all these diagnostic procedures on the same day to avoid any delay in the initiation of the treatment.
A thorough diagnostic procedure will serve both the patient and the population. First, a clear diagnosis with explanation based on imaging will increase adherence to treatment [27], a point which is not negligible given the high proportion of poorly compliant CH patients, a proportion which may reach as much as 38 % after 36 months of treatment [28]. Second, an accurate diagnosis allows a better assessment of NBS performance and a better assessment of the possible causes of incidence variations [1, 21, 23, 24]. This said, even if advantages of thyroid imaging for the diagnosis of CH are obvious, the diagnosis of all cases of thyroid dysgenesis (even the subclinical cases of thyroid ectopy or hemiagenesis) should not become the new goal of NBS for CH. Indeed, one should keep in mind that some patients with ectopic thyroid maintain normal serum thyroxine throughout life [29] and may not need LT4 treatment. Therefore, it is acceptable that some cases with thyroid ectopy are missed by CH [1]. Immediately after the diagnostic procedures, LT4 should be started. An optimal treatment of CH is essential for neurodevelopment, so reliable L-T4 preparations are crucial [30]. The treatment should be started no later than 2 weeks after birth and an initial dose of L-T4 of 10-15 µg/kg per day is recommended [14]. This practice of early and high dose treatment is based on observational studies [26, 31] and only one randomized controlled trial [32]. However, calls for additional RCTs on this question [33] raise ethical issues. Considering the controversies regarding the reliability of generic L-T4 [34, 35], brand rather than generic L-T4 tablets should be used [14] and switching brands (with inherent variable bioequivalence) should be avoided, as it requires additional blood draws to measure TSH levels [30]. The first follow-up visit should take place 2–3 weeks after initiation of L-T4 treatment, at which time the TSH level should be normalized. The patients should then, be followed every 1 to 3 months until 1 yr of age, and every 2 to 4 months until 3 yrs of age; during these visits, assessment of global development is important, and we suggest that infants with severe CH should be referred to the audiologist given the high proportion of hearing impairment in CH patients (see paragraph below) [36]. Thereafter, evaluations should be carried out every 6 to 12 months until growth is completed.
4.4 Continuous Health Burden of CH
Since the 1970s, NBS for CH followed by appropriate treatment allows most affected children to attain their full intellectual potential, with one tablet of thyroid hormone daily. However, intellectual disability may still be observed, especially in severely affected children, and this is reflected, in some cohorts, in a mean loss of 10 IQ points compared to controls drawn from the general population [37]. Although milder than in the prescreening era, such mean loss of intellectual potential has significant social and economic impact when aggregated across hundreds of individuals [15, 38, 39]: given that (1) thyroid ectopy and athyreosis account for 60 % of CH cases and that (2) each IQ point raises worker productivity by 1.7 to 2.3 % (for a lifetime earning of 700,000$), we estimate (for a country of 35 million inhabitants such as Canada) a global economic benefit of 1–1.3 million $ per year for each IQ point gained in patients suffering from severe CH, i.e., thyroid ectopy and athyreosis [38]. Even early high-dose thyroxine treatment cannot fully prevent neurocognitive deficit in the most severe cases [40]. Moreover, a fourfold increase of hearing impairment is observed in the French national cohort of CH patients [36, 39]. Consistent with the neurocognitive deficit reported in the Swiss cohort [37, 40], Leger et al. reported that male patients with athyreosis and with low socioeconomic background have an increased likelihood of not graduating from high school [39]. Of note, even if CH prognosis has improved considerably over time, some patients diagnosed in the early years of screening displayed comorbidity and mortality due to various neurodevelopmental disorders and associated malformations [41]. Finally, lower fertility has recently been reported in women (but not in men) suffering from the most severe form of CH [36].
All these data should remind us that, 40 years after the first implementation of routine screening, the health burden of CH has not disappeared, perhaps because the cause of the severe forms of CH (i.e., mainly thyroid ectopy and athyreosis) remains mostly unknown. This represents a critical barrier to further improving the outcome of CH. It is therefore important to consider the role of possible genetic markers in making an even earlier diagnosis of these most severe forms of CH.
4.5 New Persepctives: Emergence of Molecular Diagnosis and Personalized Medicine for Patients with CH?
The genetic causes of dyshormonogenetic goiters are well described, and these conditions follow classical Mendelian models of inheritance (reviewed in [19]). Those of functional CH are being unraveled and have significant clinical implications: the demonstration of a heterozygous mutation in TSHR may justify stopping treatment with thyroxine, on the basis that the persistent hyperthyrotropinemia overcomes the TSH resistance [13]. The finding of mono- or even biallelic inactivation of DUOX2 also justifies testing treatment withdrawal, since CH may be transient [42].
On the other hand, the majority of cases of thyroid dysgenesis (thyroid ectopy and athyreosis being the most frequent and severe) have no identified genetic cause. Underscoring the lack of clear genetic determinants is the fact that thyroid dysgenesis is predominantly not inherited (98 % of cases are sporadic [43]); thyroid dysgenesis also has a high discordance rate (92 %) between monozygotic (MZ) twins and a female and ethnic (Caucasian) predominance [20, 44]. Germline mutations in the thyroid-related transcription factors NKX2.1, NKX2.5, FOXE1, and PAX-8 have been identified by candidate gene screening in at most 3 % of patients with sporadic thyroid dysgenesis [19, 45]. Linkage analysis has excluded these genes in rare multiplex families with thyroid dysgenesis [46]. Moreover, evidence of nonpenetrance of mutations in genes such as NKX2.5 in close relatives of patients [47] suggests that modifiers, possibly additional germline mutations such as copy number variants (CNVs) and/or somatic mutations, are associated with thyroid dysgenesis. Indeed, a higher rate of CNVs is expected in congenital disorders [48], and Thorwarth et al. found a high rate of CNVs in thyroid dysgenesis but exclusively in athyreosis and thyroid hypoplasia [49]. Even though this study did not find recurrent CNVs and did not show any functional analyses, it provides the “proof of concept” that differences in gene copy number could account for the small heritable component of thyroid dysgenesis and shows that it is reasonable to use a higher-resolution platform to screen for CNVs and single-nucleotide variants (SNVs) in thyroid dysgenesis. Given the unprecedented high sensitivity of these “omics” methods, functional studies are now necessary to assess the pathogenicity of the genetic variants identified, and in this respect, the zebrafish model has proven its utility [50, 51].
Conclusion
Intellectual disability, health impairment, and increased mortality may still be observed in a subset of patients with CH despite the universal NBS and early treatment of this condition [36, 37, 39, 41]. To better treat children with thyroid dysgenesis, we need to (1) diagnose them even earlier, (2) better predict the severity and extent of potential neurocognitive deficits as well as the presence of additional developmental abnormalities unrelated to CH per se, and (3) propose even better treatment avoiding under- and overtreatment [52]. It is therefore crucial to better understand the cause of thyroid dysgenesis that future studies focus on the biological mechanisms responsible for thyroid dysgenesis (i.e., the severe forms of CH for which data clearly show that early treatment provides neurodevelopmental benefits) and for which NBS was originally implemented. Hopefully, this will generate novel therapeutic modalities for children affected with severe CH.
References
1.
2.
3.
Dussault JH, Laberge C (1973) Thyroxine (T4) determination by radioimmunological method in dried blood eluate: new diagnostic method of neonatal hypothyroidism? Union Med Can 102:2062–2064PubMed
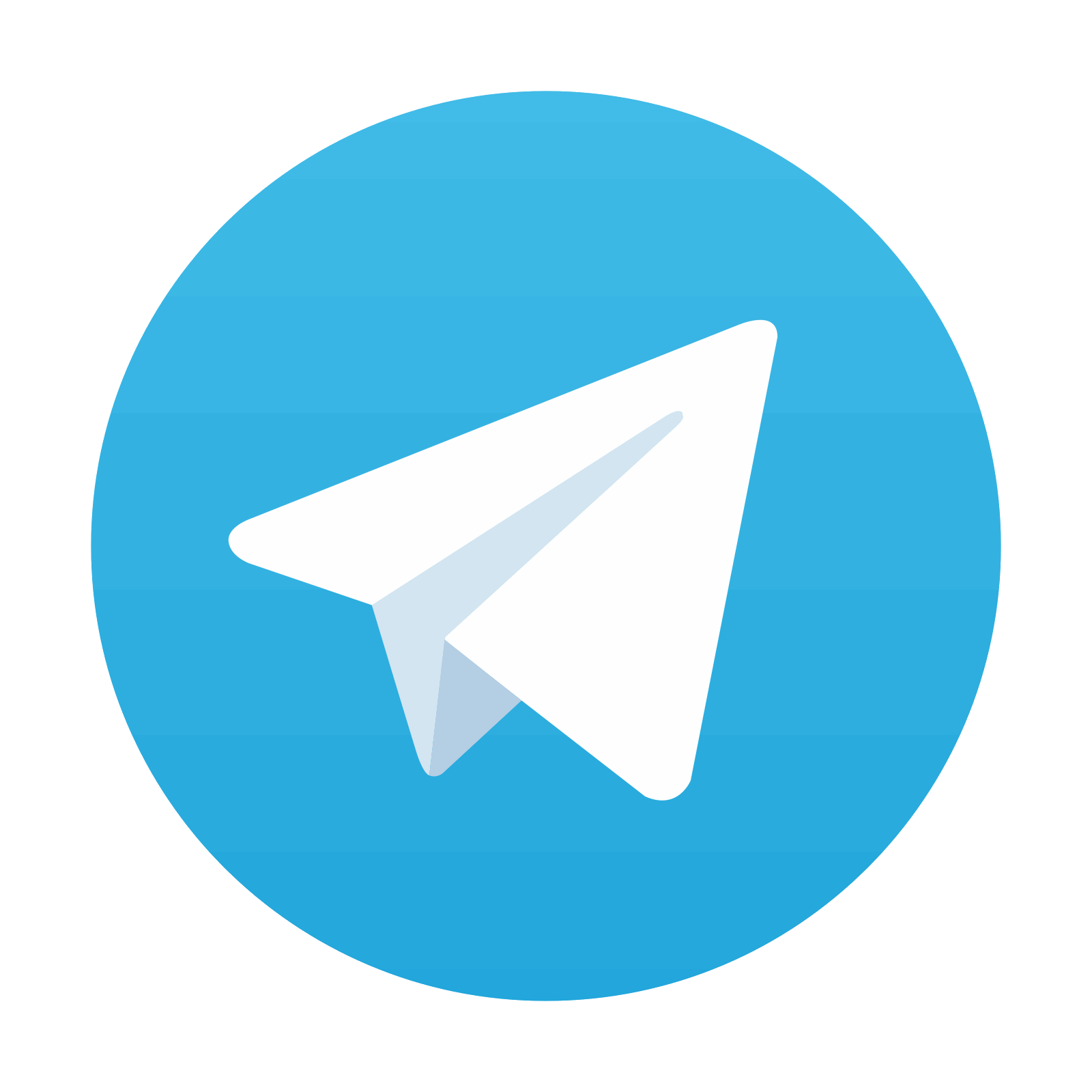
Stay updated, free articles. Join our Telegram channel

Full access? Get Clinical Tree
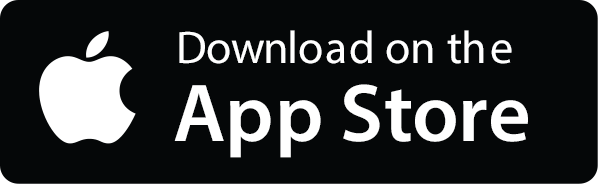
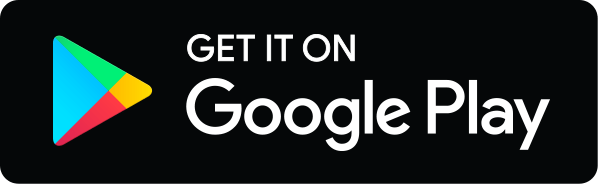