Three general approaches have been used to model myelodysplastic syndrome (MDS) in mice, including treatment with mutagens or carcinogens, xenotransplantation of human MDS cells, and genetic engineering of mouse hematopoietic cells. This article discusses the phenotypes observed in available mouse models for MDS with a concentration on a model that leads to aberrant expression of conserved homeobox genes that are important regulators of normal hematopoiesis. Using these models of MDS should allow a more complete understanding of the disease process and provide a platform for preclinical testing of therapeutic approaches.
A wide spectrum of model organisms has been used to investigate the biology of cancer. These model organisms include Drosophila melanogaster , Danio rerio , Rattus (several species), Mus musculus , and nonhuman primates. For a variety of reasons, including small body size, high fecundity, well-characterized physiology, and completely sequenced genome, the common laboratory mouse ( M musculus ) has been the model organism of choice for many cancer biologists. Three principal strategies have been used to model cancer in the mouse. Mice have been treated with known mutagenic or carcinogenic agents, most commonly small molecules or viruses. In addition, immunodeficient mice have been used in xenotransplant experiments in which primary human malignancies or cell lines are injected into the mouse. Finally, recently developed molecular genetic tools have made possible the generation of genetically engineered mice. All three of these approaches have been used to model myelodysplastic syndrome (MDS) in mice.
Nomenclature for hematopoietic disease in mice has been the topic of considerable debate. The hematopathology subcommittee of the Mouse Models of Human Cancer Consortium has developed a set of guidelines for the uniform description of hematopoietic malignancies in mice. These criteria are presented in Box 1 . It should be noted that most malignancies fulfilling the criterion for “cytopenia with increased blasts” are classified as acute nonlymphoid leukemias, and not myeloid dysplasia. A mouse that fulfills criteria 1 (cytopenias) and 2B (increased bone marrow [BM] blasts), along with splenomegaly and disseminated disease (as reflected by invasion of parenchymal tissues or >20% circulating blasts) is classified as “acute nonlymphocytic leukemia,” not MDS. Dyspoiesis in mice can be difficult to identify. Megaloblastic changes and multinucleate erythroblasts are signs of dysplasia in the erythroid lineage that are similar in both mice and humans, but ringed sideroblasts are rare in mice. Micromegakaryocytes, large megakaryocytes with unlobated nuclei, and large megakryocytes with bizarre hypersegmentation are signs of megakaryocytic dysplasia in mice. Neutrophil dyspoiesis may be evident in the form of pseudo Pelger-Huët cells with fine nuclear bridging and cells with lobated, as opposed to ring, nuclei.
- 1.
At least one of the following findings in peripheral blood
A. Neutropenia
B. Thrombocytopenia (in the absence of leukocytosis or erythrocytosis)
C. Anemia (in the absence of leukocytosis or thrombocytosis)
- 2.
Detection of a maturation defect in nonlymphoid hematopoietic cells manifested by at least one of the following
A. Dysgranulopoiesis, dyserythropoiesis, or dysplastic megakaryocytes, with or without increased nonlymphoid immature forms or blasts
B. At least 20% nonlymphoid immature forms or blasts in the bone marrow or spleen
- 3.
Disorder does not meet the criteria for a nonlymphoid leukemia
- 4.
If the disorder complies with
2A, then the subclassification is a myelodysplastic syndrome
2B, then the subclassification is cytopenia with increased blasts
Data from Kogan SC, Ward JM, Anver MR, et al. Bethesda proposals for classification of nonlymphoid hematopoietic neoplasms in mice. Blood 2002;100:238–45.
Xenotransplantation
Immunodeficient mouse models bearing human tumor xenografts have been established for the study of solid tumor biology, and for the study of hematopoietic malignancies and identifications of leukemia-initiating cells. Although the xenograft model is an attractive approach for investigating malignant disease, many human tumors are unable to be established in the mouse host. This could be caused by a variety of reasons, including toxicity from ex vivo manipulation of the malignant cells, a lack of necessary growth or survival factors in the tumor microenvironment, or an antitumor immune response generated by the host. Recently, immunodeficient mouse models for the study of leukemia-initiating cells have been subjects of careful scrutiny for the effects of the host immune system on xenografts, specifically sorted primary leukemia cells. In that study, the authors demonstrated that flow cytometric sorting of leukemic cells with a CD38 antibody led to an Fc-mediated clearance of the sorted leukemia cells in vivo. Some of these same challenges may have hindered efforts to establish xenograft models of MDS (perhaps to a further extent).
Several laboratories have attempted to engraft immortal human cell lines established from MDS patients. Until proved otherwise, by recapitulating MDS in a host, these cell lines should not be regarded as MDS cell lines, but rather as acute myeloid leukemia (AML) cell lines that have evolved, in vivo or in vitro, from MDS patients. To provide the growth factors that increase the likelihood of engraftment of F-36P, a cell line derived from a patient with MDS, severe combined immunodeficient (SCID) mice were engineered to express the human forms of granulocyte-macrophage colony–stimulating factor and interleukin-3. Despite the proliferation of F-36P in the transgenic mice, engraftment was only achieved following neutralization of natural killer cells. These mice also developed osteolytic lesions, a rare occurrence in MDS patients.
More recently, the nonobese diabetic (NOD)-SCID mouse has been used in xenograft studies of primary cells from MDS patients, because these mice are defective in complement and natural killer cell activities, in addition to B- and T-cell deficiencies. In one study, hematopoietic cells from seven MDS patients with a 5q deletion were injected into NOD-SCID mice. One of the seven mice showed evidence for low-level (12%) engraftment, and whereas CD45+CD15+ cells contained the 5q deletion, no evidence for clinical disease was found in the recipient mice. Similarly, BM from MDS patients with trisomy 8 was unable to reconstitute lymphoid and myeloid compartments in mice. In a second study, BM from patients with MDS was injected into sublethally irradiated NOD-SCID mice, with or without the delivery of human cytokines. Although subfractions of human CD45+ cells (eg, CD34-CD38+ and T cells) were detected in mice transplanted with MDS BM, decreased percentages of cells from the MDS patients engrafted compared with BM from healthy controls. Additionally, abnormal karyotypes detected in BM from MDS patients (eg, del[5q]) before the transfer of cells to mice were not identified after transplantation, suggesting that most of the human cells that had engrafted were derived from normal bone marrow elements, as opposed to the MDS clone. The authors attribute the lack of observed engraftment of clonal MDS cells to several possibilities, including decreased proliferative potential, adverse microenvironmental conditions, and susceptibility to immune attack.
To evade immune responses, and provide human cytokines in the context of a xenotransplantation assay, Thanopoulou and colleagues designed a study using NOD-SCID mice that lacked β2 microglobulin (β2m −/− ) and expressed human interleukin-3, granulocyte-macrophage colony–stimulating factor and Steel factor (c-kit ligand). The authors reported that MDS cells from 9 of 11 patients successfully engrafted, and that four of the five samples that had a clonal cytogenetic marker, such as trisomy 8 or del 5q, engrafted the MDS clone. Engraftment level of the MDS clone was typically quite low, however, less than 1% of nucleated cells, and the mice did not develop clinical MDS. Kerbauy and colleagues have recently reported the successful engraftment of human MDS clonal cells using the NOD-SCID-β2m −/− model. The percent of MDS cells in the engrafted mice was small (0.14%–4%), however, and the mice did not develop clinical disease. Taken together, these studies demonstrate that human MDS cells have the capability to engraft in immunodeficient mice, but do not produce clinical disease. The challenge for future work is to improve the engraftment of the MDS clone in sufficient numbers as to generate clinical disease in the engrafted mice.
Use of genetically engineered cells
In general, two approaches have been used to generate mouse hematopoietic cells that express (or in the case of putative MDS tumor suppressors genes do not express) genes thought to be relevant for the development of MDS. In the first approach, murine BM nucleated cells are harvested and infected in vitro with a retroviral construct that expresses the gene of interest. The infected BM nucleated cells, including hematopoietic stem and progenitor cells, which now express the gene of interest, are then transplanted into a lethally irradiated, syngeneic host mouse. The second general approach involves modification of the mouse germline, either by pronuclear injection of DNA into fertilized ova to generate transgenic mice, or by homologous recombination of mouse embryonic stem cells, which can then be injected into blastocysts, leading to generation of chimeric mice. Experiments can be designed such that a gene of interest is deleted (knocked out), or so that a gene of interest is introduced (knocked in). In either case, this second general approach leads to mice that have genetically modified germline genomic DNA. More recently, a variety of approaches using conditional promoters (eg, a tetracycline inducible promoter) or tissue-specific transgenes have given investigators the opportunity to control expression in both a temporal and spatial fashion.
Pten/Ship
Because of the influence that Ship (src homology 2- containing 5′ phosphoinositol phosphatase) and Pten (phosphatase and tensin homolog) have on the regulation of second messenger PIP3 and the fact that Ship-deficient mice develop a lethal myeloproliferative condition, Pten+/−Ship−/− mice were developed. Although hypothesized that these mice would develop leukemia, instead they displayed features consistent with aberrant hematopoiesis. Pten+/−Ship−/− mice survived only about 5 weeks and during that time, anemia, leukocytosis, and thrombocytopenia were observed. There was no evidence of leukemia; however, extramedullary hematopoiesis in the liver was found. Although increased numbers of neutrophils were present in the peripheral blood, decreases in myeloid progenitors were revealed following methocellulose culture of the BM. The BM from Pten+/−Ship−/− mice failed to reconstitute the peripheral blood of irradiated wild-type mice in a transplantation assay.
Evi1 Overexpression
Transgenic Evi1 mice were developed by Louz and colleagues by using the Sca-1 promoter for expression in hematopoietic stem cells. The expression of Evi1 in the hematopoietic tissues of these mice resulted in a gender-specific response in one of the transgenic lines whereby females remained healthy during an 18-month period in contrast to their male littermates who manifested reduced colony-forming unit erythroid formation. These male mice became severely ill because of impaired erythropoiesis and died within 7 to 11 weeks. Although these mice did not develop MDS or leukemia, the potential for Evi1 to collaborate in tumor formation was demonstrated by the ability of retrovirally infected transgenic Evi1 mice to develop myeloid leukemia more quickly than infected wild-type mice.
Expression of Evi1 in a BM transduction-transplantation model resulted in a progressive pancytopenia, however, which was inevitably fatal by 12 months. On closer examination, necropsy revealed splenomegaly in Evi1 -positive mice with an increase in erythroid precursors, iron deposition, and activated caspase-3 compared with spleens from control animals. Similar to clinical MDS, the BM displayed hyperplasia of erythroid cells and megakaryocytes. In contrast to MDS in humans, the Evi1 -induced MDS did not transform to leukemia in this study. Before the onset of overt disease, Evi1 -positive mice at 4 months had low levels of EpoR and c-Mpl in BM cells, which correlates with the defect in erythropoiesis and platelet generation observed in moribund mice months later. Follow-up studies observed that binding of Evi1 to Gata1 resulted in impaired expression of the EpoR , whereas Evi1 association with PU.1 altered myelopoiesis.
Npm1 Deletion
Npm1 was of interest for study because of its frequent mutation in AML patients (35%), location on chromosome 5q, and ability to regulate tumor suppressor genes One allele of Npm1 was inactivated in mouse embryonic stem cells by homologous recombination that produced a deletion of exons 2 through 7. After generation of chimeric mice and germline transmission, the investigators noted that Npm1 −/− ( Npm1 knockout) mice were not viable. The Npm1 −/− mice died between embryonic day E11.5 and E16.5 because of severe anemia and aberrant organogenesis. Npm1 +/− mice were viable and demonstrated some features that were consistent with MDS. Most mice aged 6 to 18 months presented at least one blood abnormality commonly associated with MDS. Npm1 +/− mice often demonstrated an elevated mean corpuscular volume and had a wider range of platelet counts, both increased and decreased, than that of wild-type mice; however, the hemoglobin levels did not differ between groups. Approximately two thirds of the Npm1 +/− mice had morphologic evidence of erythroid or megakaryocytic dysplasia, including binucleated erythroblasts and hypolobated megakaryocytes. In a follow-up study, prolonged (up to 24 months) monitoring of Npm1 +/− mice revealed an increased incidence of malignancy compared with wild-type mice, including lung and liver tumors, myeloproliferative neoplasms, AML, B-cell lymphoma, and T-cell lymphoma.
Dido1 Deficiency
Dido1 is a gene that was initially identified in a screen for genes whose expression was up-regulated during the initial stages of apoptosis. Homozygous deletion of Dido1 in mice led to a hematopoietic disease manifested by a variable degree of mild anemia and a variable degree of granulocytosis, in combination with decreased hematopoietic colony formation in vitro. Dysplastic cells in the spleen and BM were reported, but not characterized in detail, and progression to acute leukemia was not noted. The disease was thought to be consistent with a diagnosis of myelodysplastic syndrome/myeloproliferative disease (MDS-MPD) and it was transplantable to recipient mice, indicating that it was cell-autonomous.
NUP98-HOXD13
Over 20 chromosomal translocations involving the NUP98 gene associated with hematopoietic malignancy have been identified. Pineault and colleagues described a NUP98-HOXD13 mouse model in which primary murine BM cells were transduced with a retrovirus carrying a NUP98-HOXD13 fusion gene, which was initially identified in a patient with MDS. These cells were subsequently transplanted into irradiated recipient mice. Significant decreases in white blood cells were observed in mice expressing the NUP98-HOXD13 fusion, which was further characterized by a reduction in B220+ and CD4+/CD8+ lymphocytes. In contrast, significant increases in myeloid BM cells ( Gr1+/Mac1+ ) were identified. Results from colony-forming unit spleen assays with NUP98-HOXD13 –transduced BM cells indicated a decrease in erythroid precursors (identified by the Ter119 cell surface marker), an increase in myeloid Gr1+ cells, and an overall increase in the number of colony-forming unit spleen colonies at day 12. Although some of these mice developed a myeloproliferative disease as early as 4 weeks following transplantation, they did not transition to AML except when mice were engineered to simultaneously express Meis1.
In comparison, NUP98-HOXD13 ( NHD13 ) transgenic mice that used Vav 1 regulatory elements to direct expression of the NHD13 transgene in hematopoietic tissues developed anemia, neutropenia, and lymphopenia at 4 to 7 months. These mice also had a variable degree of thrombocytopenia and macrocytosis; however, these differences were not statistically significant. Despite the peripheral blood cytopenias, these mice had hypercellular or normocellular BMs, indicating ineffective hematopoiesis. Morphologically, the NHD13 mice showed dysplasia in the form of binucleate erythroblasts, megaloblastosis, pseudo Pelger-Huët cells, and micromegakaroyctes. Similar to the progression pattern observed in patients with MDS, approximately half of the NHD13 mice with MDS developed an acute leukemia, typically at 10 to 14 months of age. A variety of leukemic phenotypes were identified, most commonly AML. Some animals developed pre–T-lymphoblastic leukemia-lymphoma (pre–T-LBL), however, which is rarely seen in secondary leukemia that evolves from patients with MDS. Although the development of pre–T-LBL was somewhat surprising, it was not completely unexpected given that NUP98 fusion genes have been associated with both T-cell and myeloid malignancies. Of note, although the initial studies of the NHD13 fusion used FVB/N mice, the entire study was subsequently repeated with C57Bl/6 mice. The results were remarkably similar in terms of peripheral blood cytopenias, dysplastic morphology, and progression to acute leukemia, demonstrating that the effect of the transgene was not mouse strain-dependent. There are both similarities and differences between the transgenic and retroviral transduction-transplantation models. Both lead to anemia, lymphopenia, and neutropenia initially; however, the retrovirally transduced model quickly transforms to a myeloproliferative disease, whereas the transgenic mice transform more slowly, usually to an AML. The differences between the transgenic and retroviral transduction models could be caused by differences in mouse strain, ex vivo manipulation of cells, effects from BM reconstitution, or differential susceptibility of the BM stem and progenitor cells by the NUP98-HOXD13 retrovirus.
SALL4 Overexpression
SALL4B is a zinc finger transcription factor expressed early during embryonic development, and is involved in the transcriptional regulation of Oct4 and Nanog . SALL4B was constitutively expressed in mouse tissues under the control of the cytomegalovirus promoter. These mice developed some classic features of MDS within a few months of life, including peripheral blood leukopenia and dysplasia, and a mild anemia. BM from these mice was hypercellular and showed an increased number of Mac1+/Gr1+ cells, and increased apoptosis when compared with wild-type counterparts. Half of the transgenic mice transformed to AML by an average of 14.5 months of age and the disease was transplantable. In addition to the hematopoietic abnormalities, most of the transgenic mice were also diagnosed with polycystic kidneys and a minority was identified as having cerebral spinal fluid accumulation in the brain that affected motor function.
BCL-2 and NRAS
To study the process of MDS transformation to AML, overexpression of an inducible BCL-2 transgene along with simultaneous expression of an NRAS mutant ( NrasD12 ) were selected for examination in a mouse model. In collaboration with the increased proliferative effects attributed to mutant NRAS , it was predicted that a complementary mutation, induced by overexpression of antiapoptotic BCL-2 , would induce MDS or AML. The authors found that mice that expressed BCL-2 and mutant NRAS developed a mild leukocytosis and thrombocytopenia, without anemia. The mice also displayed hepatosplenomegaly, dysplastic neutrophils, and increased BM blasts (mean of 15%). Serial transplantations of the lin neg but not the lin pos cells from mice with MDS into immunodeficient mice were successful.
RUNX1 Mutations
Watanabe-Okochi and colleagues infected mouse BM with retroviral constructs that encoded one of two different RUNX1 (also know as AML1 ) gene mutations that were initially identified in patients with MDS or AML. The D171N mutation is a missense mutation involving the runt homology domain, and the AML1-S291fsX300 mutation is a frameshift mutation that results in truncation of the C-terminal region of the AML1 protein. Most mice that expressed the D171N RUNX1 mutation developed MDS with an increased red blood cell mean corpuscular volume, dysplasia, anemia, leukopenia, and progression to AML ( Table 1 ). Of note, a fraction of the RUNX1 -D171N mice had integrations of the retroviral vector used to express the RUNX1 mutant at the Evi1 locus, and transplantation of BM cells coinfected with RUNX1 -D171N and Evi1 reduced the latency of the disease to 3 to 5 months. This study provides support for the cooperation of RUNX1 mutants and Evi1 for inducing MDS with progression to AML.
Genes | Technique | Findings | Reference |
---|---|---|---|
Pten/Ship | Pten haploinsufficent Ship knockout | Leukocytosis, neutrophilia, anemia, thrombocytopenia, hepatosplenomegaly, extramedullary hematopoiesis. Lethal by 5 weeks of age, no leukemia. | |
Evi1 | Retroviral transduction | Anemia, thrombocytopenia, variable leukopenia, hypercellular BM, BM dysplasia, uniformly fatal (14 mo), no leukemia. | |
Npm1 | Npm1 haploinsufficent | No anemia, variable thrombocytosis or thrombocytopenia, BM dysplasia and hypercellularity. Lung, liver tumors, AML, lymphoma at advanced age. | |
Dido | Knockout | Variable anemia, variable leukocytosis, variable splenomegaly, BM dysplasia. Survival 55% at 26 mo, no leukemia. | |
NUP98-HOXD13 | Transgenic (Vav promoter) | Anemia, macrocytosis, leukopenia, variable thrombocytopenia, hypercellular-normocellular BM, dysplasia. Survival 5% at 14 mo, 60% leukemic transformation (AML and ALL). | |
SALL4B | Transgenic (CMV promoter) | Mild anemia, mild neutropenia, variable thrombocytosis, BM dysplasia and hypercellularity. Survival 12% at 24 mo, 50% leukemic transformation (AML). | |
BCL2/ NRASD12 | Transgenic(tTA, MRP8 promoters) | No anemia, mild leukocytosis, mild thrombocytopenia, BM dysplasia, hepatosplenomegaly, survival and leukemia not reported. | |
RUNX1 | Retroviral transduction (S291fs) | Anemia, macrocytosis, leukopenia, thrombocytopenia, hypercellular-normocellular BM, dysplasia. Survival 10% at 14 mo, 60% leukemic transformation. | |
Arid4a | Knockout | Mild anemia, leukopenia, lymphopenia, thrombocytopenia, hypercellular-normocellular BM with fibrosis. 12% AML at 12–22 mo. | |
Polg | Knock-in of mutant ( Polg A/A ) | Anemia, lymphopenia, BM dysplasia, mitochondrial dysfunction. Lethal by 16 mo because of severe megaloblastic anemia. |
Arid4a Deficiency
ARID4A and ARID4B (also known as “RBBP1” and “RBBP1L1”) are proteins that contain an AT-rich interaction domain (ARID). ARID4A, but not ARID4B, interacts with RB and recruits histone deacetylases to E2F-dependent promoters. Arid4a deficient ( Arid4a−/− ) mice were identified by a gene trap mutagenesis screen. Young (2–5 month old) Arid4a−/− mice developed lymphopenia, neutropenia, thrombocytopenia, and a mild anemia, with a normocellular BM. The peripheral blood cytopenias described previously became progressively more severe, with a marked increase in monocytes, as the mice aged. These changes were accompanied by BM fibrosis with increased reticulin fibers and hepatosplenomegaly secondary to a compensatory extramedullary hematopoiesis. These features are reminiscent of a chronic myelo-monocytic leukemia (CMML) that has evolved from a MDS/MPD. In 12% of the mice, the disease transformed to a frank AML, with high peripheral blood white blood cell, at ages between 12 and 22 months. BM from the Arid4a−/− mice had decreased levels of several Hoxb cluster genes ( Hoxb3 , 5 , 6 , and 8 ) that are normally expressed in BM, but levels of Hoxa7 , Hoxa9 , and Meis1 , which are up-regulated in some MDS cases in mice and patients (see previously), were normal. Although mice that had deleted both Arid4a and Arid4b were not viable ( Arid4a−/− Arid4b−/− ), Arid4a −/− Arid4b+/− developed AML with a higher frequency (83%) at an early age (7–15 months).
DNA Polymerase Gamma (Polg) Mutations
Although controversial because of the inexorable accumulation of mitochondrial DNA mutations with age, some studies have suggested that MDS patients have an increased frequency of mitochondrial DNA mutations. DNA polymerase gamma (Polg), a protein that is encoded by the nuclear genome, is responsible for replication of mitochondrial DNA. Polg has two enzymatic activities: a C-terminal polymerase and an N-terminal “proofreading” exonuclease. Mice with targeted instability of the mitochondrial genome were produced by selective elimination of the N-terminal proofreading activity through gene-targeted creation of a missense mutation ( Polg D257A); the mutant allele is designated Polg A . Homozygous mutant mice (designated Polg A/A ) show an increase in the frequency of mitochondrial DNA mutations and exhibit features of premature aging, including hair loss, thymic involution, testicular atrophy, and loss of intestinal crypts. In addition, the mice showed a progressive, fatal severe megaloblastic anemia and impaired lymphopoiesis, but there was no evidence for transformation to acute leukemia. Of note given the loss of intestinal crypts in the Polg A/A mice, the megaloblastic anemia was demonstrated to be caused by mitochondrial defects in the hematopoietic compartment, as opposed to malabsorption, by transplantation of Polg A/A cells in wild-type recipient mice.
Clinical Implications of Modeling MDS in Mice
Mouse models are developed to study, in detail, questions that cannot easily (or are impossible to) be addressed in the clinic. A useful animal model for MDS would accurately represent genetic aberrations in patients, provide similar microenvironmental conditions for reproducing hematopoietic phenotypes (eg, impaired differentiation and increased apoptosis), recapitulate patient symptoms and responses to existing treatment regimens, and progress to AML with similar kinetics and features as seen in the clinic. Although a model that satisfies all of these criteria may not exist, cancer biologists have several different models to choose from, and can select an available animal model, being cognizant of its caveats. Once an MDS model is developed and validated, it can be used to advance the understanding of the molecular biology of the disease and provide a platform for cultivation of new therapeutic approaches. This section focuses on mice with NUP98-HOX fusions, because there have been a number of follow-up studies performed with these mice beyond the initial description, providing examples for the types of additional studies that are possible with these animal models.
Although rare, many of the NUP98 translocations, including NUP98-TOP1 , NUP98-DDX10 , and NUP98-HOX fusions, have been seen in patients with MDS, especially younger patients. Furthermore, the NUP98-HOXA9 and NUP98-HOXD13 translocations both lead to overexpression of HOXA9 in mice; marked (10-fold–1000-fold greater than normal BM or CD34+ cells) overexpression of HOXA9 is a common finding in patients with AML and MDS. NHD13 mice develop a variety of leukemias including myeloid, erythroid, megakaryocytic, and undifferentiated; however, although unexpected, about 15% to 25% of NHD13 mice developed pre–T- or pre–B-LBLs. This is in contrast to reports of human MDS transformation to lymphoid leukemias, which are rare. Despite this difference between MDS patients and mouse models of the disease, 60% of NHD13 mice developed acute leukemias and 20% died because of severe MDS, statistics that are similar with patient progression.
Additional studies based on the NHD13 translocation have sought to describe the implications of this mutation and to understand its mechanistic role in MDS. The NHD13 protein can inhibit maturation of the K562 cell line, suggesting that NHD13 expression plays a role in hematopoietic precursors by impairing their differentiation. To study the impaired differentiation attributed to the NHD13 protein, colony-forming cell assays were performed with BM from the NHD13 transgenic mice. Although the number of colonies formed by plating whole BM from wild-type and NHD13 mice were similar, 10-fold fewer total colonies were observed from the NHD13 lin neg BM population (which contains the stem and progenitor cells) than from the wild-type lin neg BM cells. Lin neg cells from NHD13 mouse BM also showed dramatic increases in apoptosis following treatment with hematopoietic cytokines that promote survival and differentiation of wild-type murine hematopoietic stem and progenitor cells. Moreover, most of the surviving NHD13 cells were undifferentiated lin neg cells. Taken together, these findings indicate that the NHD13 fusion impairs differentiation of hematopoietic stem and progenitor cells and predisposes these cells to apoptotic death; these findings are reminiscent of the maturation arrest and increased apoptosis seen in patients with MDS.
Subsequent studies in the lymphoid system of NHD13 mice revealed impaired B-lymphocyte differentiation at the pro-B to pre-B transition and impaired differentiation of T lymphocytes during the transition from double negative (DN) DN2 to DN3 thymocytes. Hoxa7 , Hoxa9 , and Hoxa10 were overexpressed in the thymus of NHD13 mice compared with that of wild-type mice, an association that has previously been observed with impaired thymocyte differentiation.
Additional studies have identified a long-term repopulating MDS-initiating cell by demonstration of successful engraftment of lin neg NHD13 -expressing BM cells into primary and secondary lethally irradiated wild-type recipients. Importantly, mice that received the NHD13 BM developed signs and symptoms of MDS, including peripheral blood cytopenias caused by ineffective hematopoiesis, morphologic dysplasia, and transformation to acute leukemia. These studies also demonstrated that, in a competitive repopulation assay, the NHD13 BM cells were able to out-compete normal wild-type BM cells, even when a 10-fold excess of wild-type BM was transplanted. Finally, limiting dilution experiments identified the frequency of the MDS-initiating cell to be similar to the frequency of hematopoietic stem cells from wild-type mice (approximately 1 per 20,000).
In addition to elucidating the role of NHD13 in MDS, its contribution to the development of leukemia is also of interest. In the context of the recently developed two-class mutation hypothesis for AML, the NUP98-HOX fusions can be classified as Type II, or differentiation-impairing, mutations. Infection of primary BM cells by replication-competent retroviral particles predisposes mice to develop leukemia through a process known as “retroviral insertional mutagenesis.” This happens because of integration of the retrovirus, with the retroviral LTR serving as a powerful promoter-enhancer, near to cellular proto-oncogenes. Activation of two or more collaborative proto-oncogenes leads to leukemia or lymphoma in the infected mice. When NHD13 mice were infected with the MOL4070LTR retrovirus, the time from MDS to leukemic transformation was shortened by 6 months, providing further support that a second event is needed in collaboration with the NHD13 fusion for progression to leukemia. Potential collaborators with NHD13 identified by retroviral insertional mutagenesis include Meis1 , Mn1 , Gata2 , miR29a , and miR29b1 .
To identify spontaneous mutations that might collaborate with NHD13 as the MDS converts to an AML, genes frequently mutated in human AML were resequenced in leukemic tissue from NHD13 mice that had developed AML. In NHD13 mice with AML, 32% of mice assessed were found to have a Nras or Kras mutation of codon 12. Interestingly, no codon 13 or 61 mutations were found, although a codon 61 Nras mutation was identified in a NHD13 mouse with a pre–T-LBL. One mouse was found to have a mutation in Cbl , specifically an exon 8 deletion. These results, along with the lack of mutations observed in Npm1 and Runx1 (both classified as Type II, or differentiation-blocking mutations), are again consistent with NHD13 being a Type II mutation. Given that KIT mutations are most commonly found in patients with core-binding factor translocations, it may not have been surprising that Kit mutations were not identified in the NHD13 AMLs, because the NHD13 fusion does not seem to affect the core-binding factor pathways. It was curious that no Flt3 mutations were identified, however, because Flt3 mutations are commonly associated with lesions (eg, fusions of the MLL gene) that up-regulate HOXA cluster genes.
In addition to verifying that mutations (eg, Runx1 point mutations or NUP-HOX gene fusions) found in MDS patients are important in the disease process, mouse models provide a platform that can be used for preclinical testing. In addition to testing small molecules, singly or in combination, these animal models can be used to study the mechanisms by which allogeneic hematopoietic stem cell transplant is curative for patients with MDS. Hematopoietic stem cell transplant is the only known definitive treatment for MDS patients, and is thought to be effective by a combination of myeloablative radiochemotherapy and adoptive immunotherapy leading to a graft-versus-tumor effect. Inbred strains of mice with complete or partial major histocompatibility complex mismatches afford the ability to test the relative contributions of these two effects. In addition, these animal models may also be useful for examining possible autoimmune contributions to MDS initiation as proposed when immunosuppressive therapy resulted in positive responses in certain patient subsets.
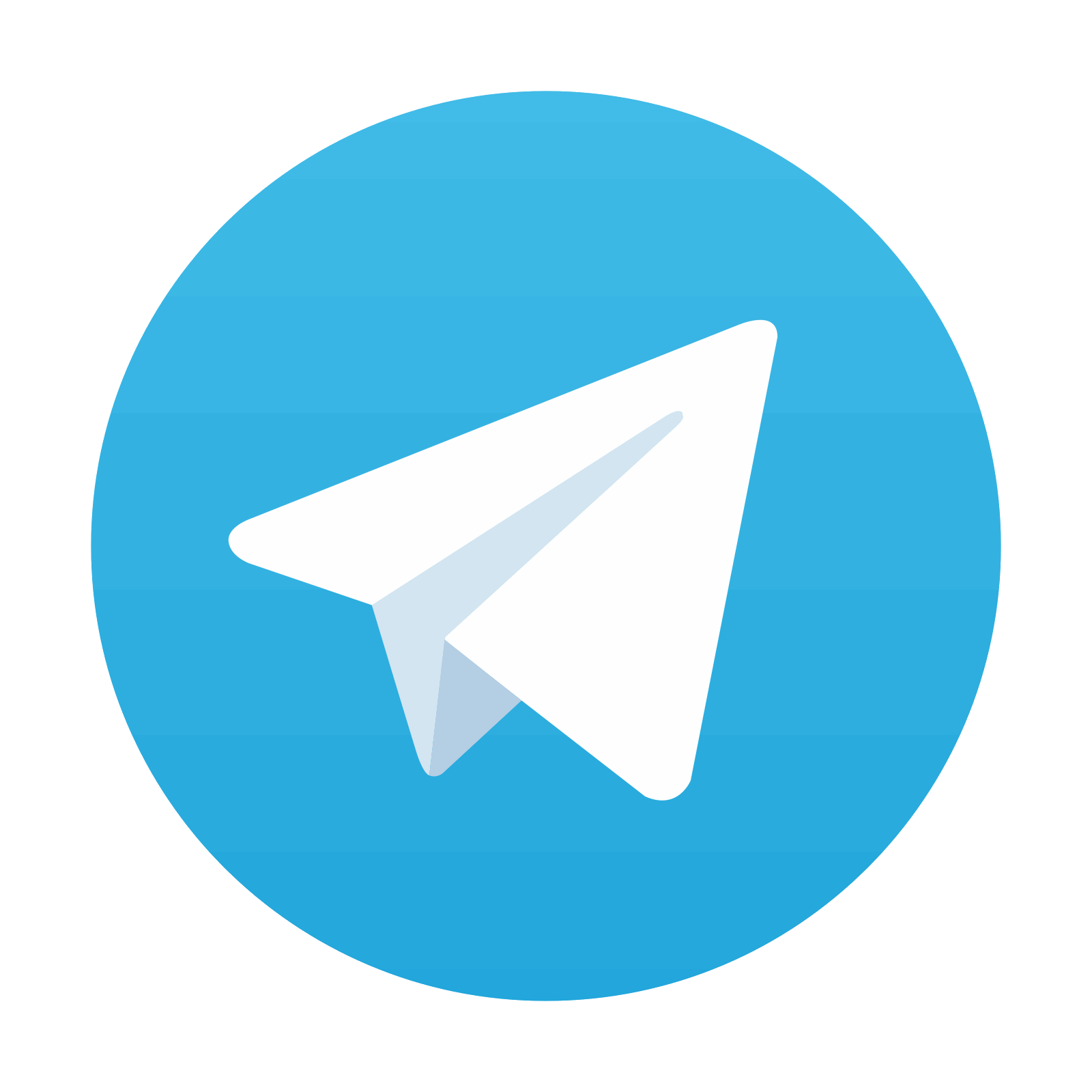
Stay updated, free articles. Join our Telegram channel

Full access? Get Clinical Tree
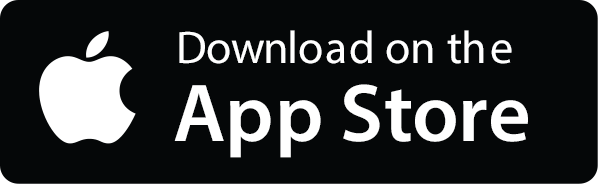
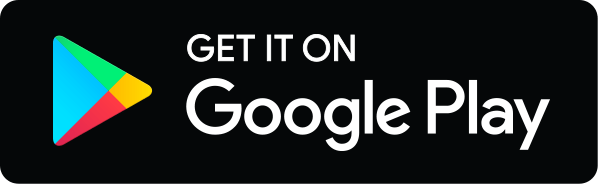