Introduction
In diabetic neuropathy (DN), symptoms and signs depend on the severity of impairment of sensory, autonomic, and motor nerves, clinically presenting in a symmetrical or asymmetrical pattern . In up to 50% of patients with long-term diabetes the bilateral and symmetrical presentation, termed diabetic sensorimotor polyneuropathy (DSPN), can be found, and since it is by far the most common presentation of DN, the literature often uses DSPN synonymously with DN . During the course of DSPN, the clinical presentation is dominated by symptoms and signs of sensory nerve damage, and consequently a prevalent belief has been that the motor system is involved only in advanced cases of DSPN . Leaving aside DSPN, motor involvement is frequently found in other types of DN such as diabetic lumbosacral radiculoplexus neuropathy and several mononeuropathies . With that being said, due to the much less common presentation of DN types unrelated to DSPN, other peripheral nerve disorders should be considered in patients with diabetes with a clinical presentation predominated by motor impairment, for example, immune-mediated neuropathies, where significant improvement can be seen following treatment with immunoglobulins . Interestingly, in contrast to the predominant sensory symptomatology in DSPN, in nerve conduction studies (NCS), motor and sensory nerves are impaired to a similar degree . Minimal F-wave latency, being a motor conduction parameter, has in fact been found to be the most sensitive conduction parameter to detect peripheral polyneuropathies, including DSPN . These findings indicate that the predominance of sensory symptoms is not primarily the consequence of less severe degeneration in motor nerves. Resprouting of motor nerves and collateral reinnervation of motor units are believed to be the compensatory mechanisms alleviating the consequences of muscle denervation, forming the basis for why motor symptoms and deficits are primarily observed later in the course of DSPN . This proposition is supported by recent studies by Kristensen et al. reporting decreased motor unit numbers and increased motor unit size in patients with DSPN . Accelerated muscle weakness in diabetes is attributed to the degree of DSPN, following denervation and progressive muscular atrophy . Atrophy and weakness of the foot muscles can initially be observed; however, this often goes unnoticed by patients contributing to the fact that motor dysfunction can remain unrecognized until later stages of DSPN . Recent studies applying imaging modalities such as magnetic resonance imaging (MRI) suggest that fat infiltration and atrophy may be detectable in skeletal muscles of the lower extremities even in earlier stages of DSPN . Interestingly, MRI studies suggest a less obvious distal–proximal gradient of muscular abnormalities regardless of the distribution of DSPN, with a larger decrease in proximal muscle strength and fat infiltration than previously believed . It is well established that DSPN results in distal atrophy and MRI signal abnormalities within skeletal muscles. Still, the understanding of muscle dysfunction in patients with diabetes is incomplete, and emerging evidence also suggests the presence of a general skeletal muscle dysfunction in patients with diabetes, termed diabetic myopathy, related to both neuropathic and metabolic deficits . Fig. 9.1 illustrates the proposed combined neuromuscular and functional muscular deficits observed in relation to diabetes and DSPN.
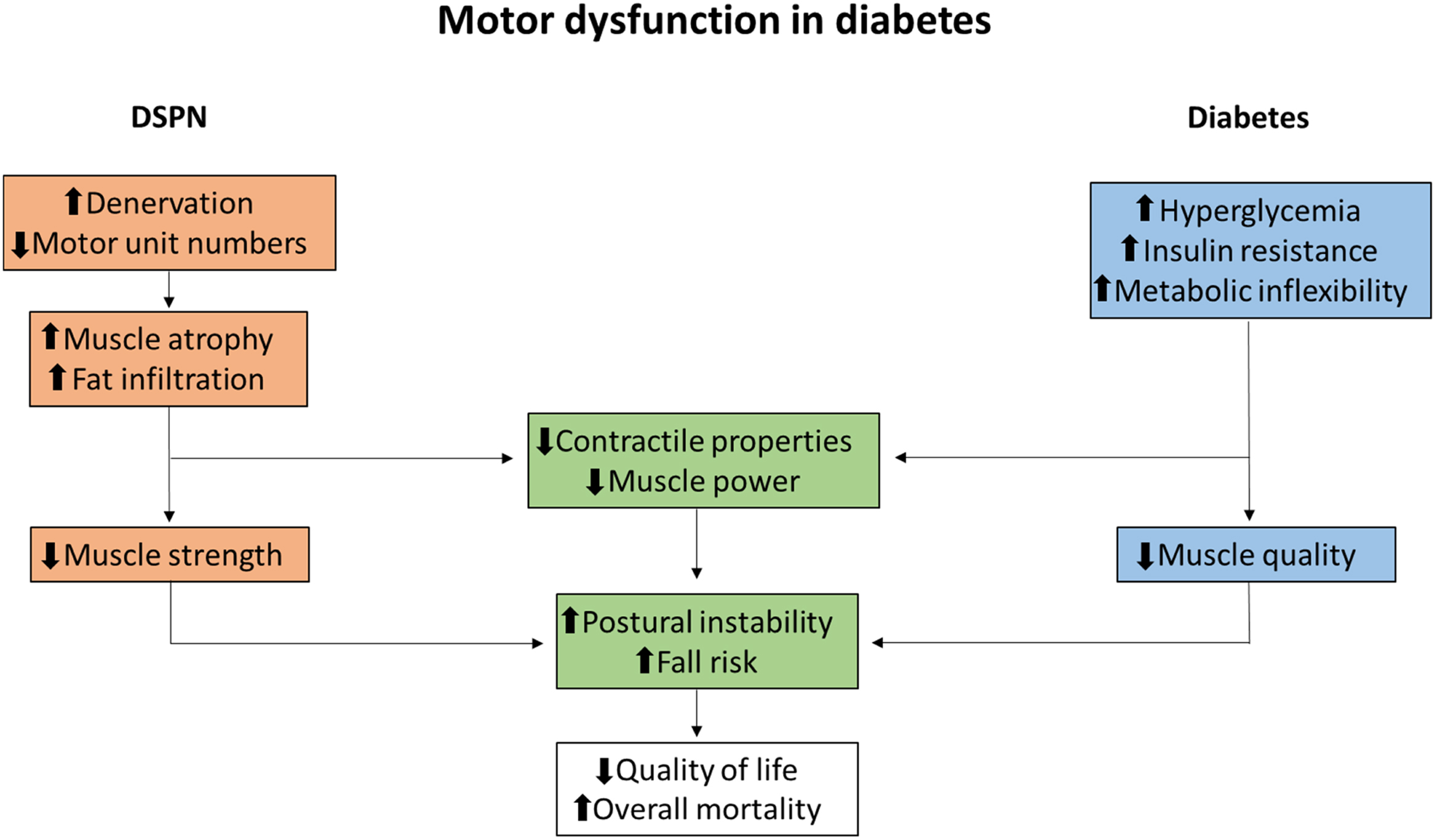
In this chapter, we will deal with motor dysfunction in diabetes, including peripheral motor neuropathy in DSPN, muscle weakness and functional deficits, and compositional and structural changes. Finally, we will consider the management of motor deficits in DSPN and diabetes, including the effects of exercise interventions.
Motor nerve dysfunction
Electroneuronography
Pathological changes in DN include axonal degeneration and demyelination of the peripheral nerves, which can be observed in motor as well as in sensory nerves. Histological studies with findings of decreased nerve fiber density are consistent with the clinical and electrophysiological impairments, in line with the belief that nerve fiber loss is the primary reason for the neurological deficits seen in diabetes . Slowing of nerve conduction and decreased response amplitudes of motor and sensory nerves are frequent neurophysiological findings . Consensus of electrodiagnostic criteria for the presence of DSPN follows the guidelines of Dyck requiring at least one abnormal electrodiagnostic finding in two separate nerves, one of which is required to be the sural nerve . Standard examination follows the guidelines of the American Association of Neuromuscular and Electrodiagnostic Medicine (AANEM) suggesting to start with the examination of the peroneal and sural nerves on one leg, and in the case where neurophysiological measures are normal, no further action is recommended . However, recent studies implicate that DSPN may go unnoticed using this strategy, since measures of the tibial nerve have proven to be more sensitive to motor involvement than peroneal measures . In addition, Tankisi et al. observed that peroneal nerve abnormalities were more common in participants without peripheral neuropathy, suggesting abnormalities in the peroneal nerve to be less specific. Despite frequent abnormalities in motor nerves, the sural nerve remains the most specific NCS measure and should be included in the criteria to rule out other peripheral motor nerve disorders and identify patients without DSPN . Both the peroneal and tibial nerve may show abnormalities in subjects without peripheral polyneuropathies, which may be due to a larger variation in the conduction of motor nerves in healthy subjects. Tankisi et al. observed that prolonged minimal F-wave latency, a measure of pure motor nerve function of the whole length of the motor axon, was the most common motor nerve abnormality in participants with peripheral neuropathies, and more common in the tibial (72%), than in the peroneal nerve (58%). This is in line with previous studies where F-wave latency has been found to be the most sensitive and reproducible NCS measure of motor neuropathy . Yet, until recently minimal F-wave latency has not been part of the standard electrodiagnostic battery of DSPN.
Few studies have examined motor nerve conduction within the central nervous system in patients with diabetes. Increased latencies and abnormal cortical excitability within central motor pathways have been described in clinical and preclinical animal studies . Overall, the clinical significance of these findings is elusive and unlikely to contribute significantly to signs and symptoms of clinical motor dysfunction.
Electromyography
Electromyography (EMG) enables the detection of neurogenic and myogenic factors for evaluation of neuromuscular deficits such as muscle weakness and atrophy. In patients with diabetes, early studies of EMG report a number of abnormalities, including fibrillation potentials, decreased recruitment pattern, larger motor unit potentials, and a higher number of polyphasic potentials . These findings emphasize the presence of a neurogenic motor dysfunction in patients with DN induced by axonal loss and incomplete compensatory reinnervation observed in NCS. For sensory nerves, skin biopsies can provide a direct estimate of the number of distal nerve segments. Clearly no such direct estimates of the number of motor axons are possible. Yet, neurophysiological techniques enable an indirect estimation of the degree of loss of motor axons. Early studies implicated that computer-assisted motor unit number counting and subtraction methods may help to quantify denervation and reinnervation of motor units . For many years, these techniques have been associated with a number of limitations, and as a consequence motor unit number estimation (MUNE) methods have only sparsely been used . Recently, emerging methods, like MScanFit MUNE, have proved to provide reliable estimates of motor unit numbers covering the range of 5–160 units with an overall error rate of only 6.9% . In recent clinical studies of DSPN, MScanFit proved more sensitive in detecting motor impairment than conventional NCS . Interestingly, MScanFit evaluating motor unit numbers and size of the median nerve detected a higher incidence of motor abnormalities (AUC 0.84) than motor NCS of the median, tibial, and peroneal nerve, including measures of minimum F-wave latency (AUC 0.79). This may be due to resprouting and collateral reinnervation of motor units resulting in conventional NCS not being able to detect motor changes before substantial motor dysfunction is present. This is supported by the study by Daube et al. who found that decreased CMAP amplitudes cannot be detected until about 50% of the motor units are lost . Fig. 9.2 illustrates ROC curves of MScanFit and NCS parameters and their ability to discriminate between healthy subjects and patients with DSPN. In Fig. 9.3 , loss of motor units is presented from a patient with DSPN and compared with a healthy subject. Comparisons of MScanFit with NCS in the lower extremity of patients with type 2 diabetes show similar findings as for the upper extremity . Kristensen et al. found a substantial decrease in the motor unit numbers in the anterior tibial muscle of patients with DSPN (AUC 0.90) as compared to CMAP amplitudes (AUC 0.77). Other studies applying similar MUNE methods in patients with type 1 and type 2 diabetes have shown similar results . These emerging MUNE studies are important to obtain a better understanding of the pathophysiology in DSPN.
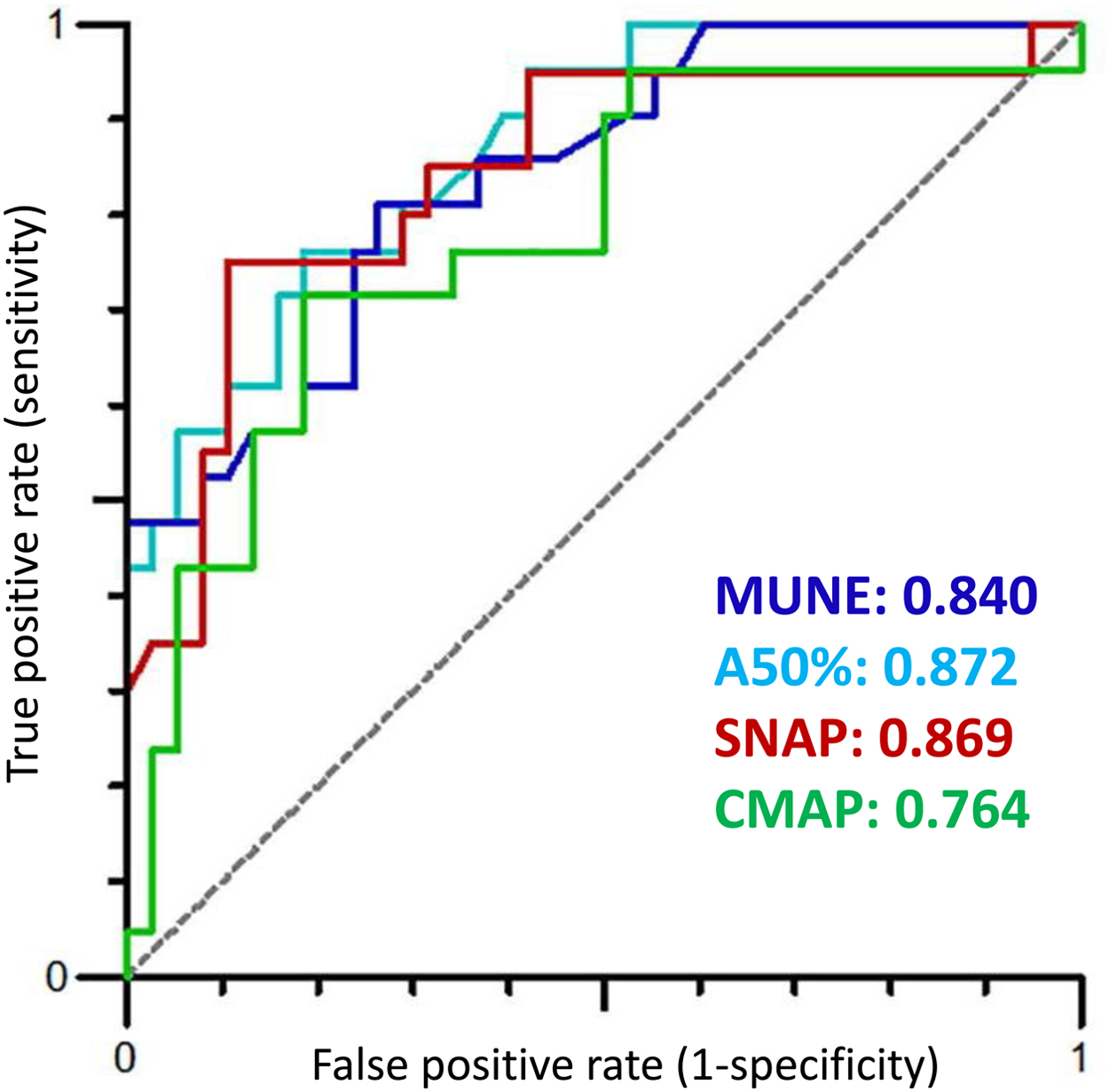
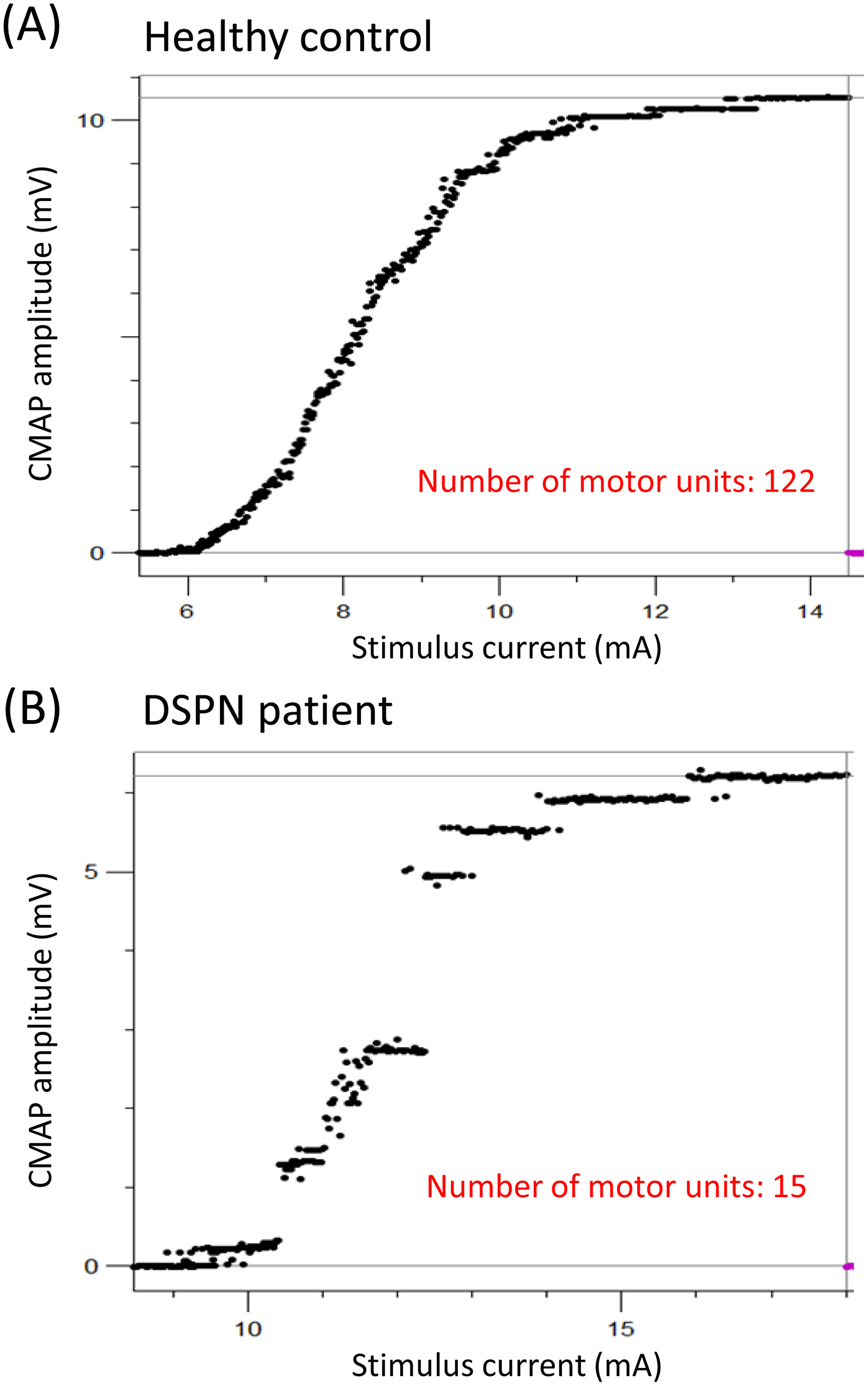
Based on the symptomatology a common assumption is that DSPN primarily targets sensory nerves . Findings of studies on MUNE methods clearly demonstrate that motor and sensory nerves are equally affected . Still, in the clinical setting EMG investigations are often considered redundant, except in cases with an unusual presentation. However, with the emerging evidence that MUNE methods provide motor measures with comparable sensitivity to sensory NCS parameters, future clinical studies could determine the utility of MScanFit MUNE as a clinical tool in DSPN.
Muscle strength and functional impairments
Muscle strength evaluation
Motor examination may include (1) functional tests, (2) manual muscle testing, and (3) dynamometric evaluation, including hand-held dynamometers and stationary dynamometers, providing quantification of isokinetic or isometric muscle strength.
Functional tests are easily implemented without the need of testing equipment and are part of a standard neurological examination. In early studies, the ability to walk on heels and toes has often been applied as measures reflecting muscle strength of the ankle dorsal and plantar flexors . However, these tests are linked with limitations. First, inability to walk on heels or toes is only present in patients with severe DSPN . Second, since the test is dichotomous, with only two possible outcomes, grading of motor dysfunction is not possible, providing a poor measure for differentiation and evaluation of changes in longitudinal studies. Other functional motor tests include the 6-minute walk test and 5-time sit-to-stand test. These tests reflect the overall functional muscle capacity and endurance and provide reliable measures in identifying the risk of falling in patients with diabetes . Moreover, functional tests have proved valuable as prognostic indicators of mortality in the elderly population as well as for evaluation of skeletal muscle health, a factor increasingly considered important for the overall health of patients with diabetes . However, the exact relation between abnormal functional test findings and muscle strength remains unknown, and likely a number of other causes may affect functional motor capacity, including loss of proprioceptive sensation, poor vision, pain, overweight, arthritis, ageing, and limited joint mobility.
Manual muscle testing is part of a standard neurological examination and provides semiquantitative measures of muscle strength. As with functional tests, it is easily implementable. Still, manual muscle testing is highly examiner-dependent with low intra- and interrater reproducibility. Even in experienced hands, it is insensitive compared to quantitative assessments of muscle strength, especially in strong muscle groups with a short lever arm . Thus manual muscle testing cannot reliably detect even a 50% reduction of strength of the plantar flexors.
Dynamometric examination of isokinetic and isometric muscle strength has become increasingly popular in research settings for the evaluation of muscle strength in patients as well as healthy subjects . Dynamometry provides clear advantages compared to manual and functional testing when it comes to quantifying muscle strength. Isometric and isokinetic dynamometries enable quantitative information on the static and dynamic muscle strength of all major muscle groups of the upper and lower extremities. Furthermore, with strictly standardized testing procedures, low variation for repeated measures (<10%) and day-to-day testing can be obtained . Fig. 9.4 depicts the set-up for evaluation of knee flexors and extensors with the use of the isokinetic dynamometer Biodex-system 3 PRO-dynamometer (BioDex Medical Systems, Inc., NY, United States). Normative reference material of healthy participants exists applying the above BioDex set-up enabling correction for the influence of obvious explanatory variables of age, sex, height, and weight .
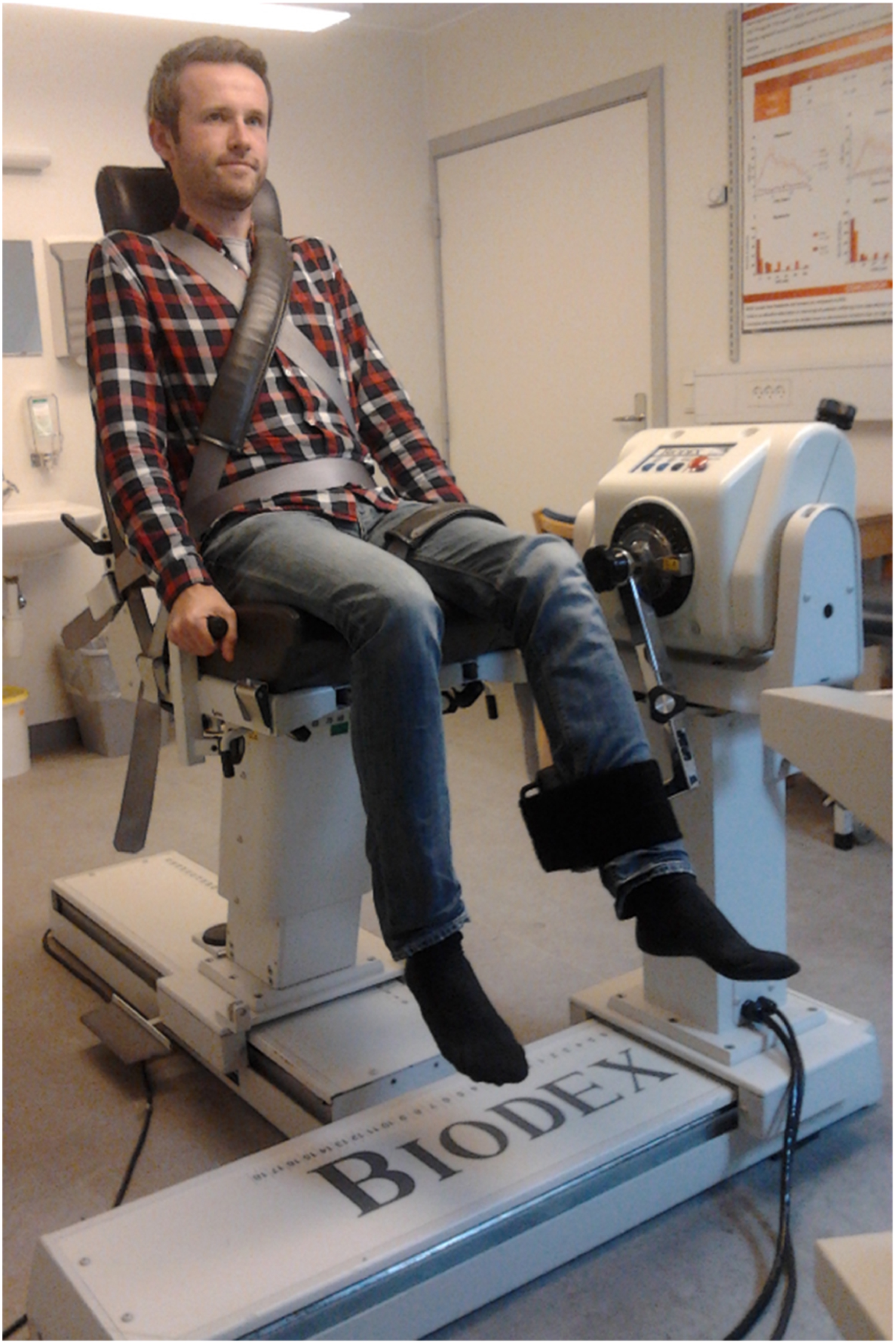
Determination of handgrip strength can easily be performed as it only requires a handgrip dynamometer. However, in most patients with diabetes this is not relevant since the upper extremities are infrequently weakened and if so, may be due to mononeuropathies such as entrapment of the median nerve rather than DSPN.
Diabetic myopathy
The importance of muscle impairments in diabetes to the overall health of patients is becoming increasingly evident . Deteriorating muscle health is linked with postural instability, dyslipidemia and dysregulated blood glucose levels with increased risk of diabetic complications, morbidity, and ultimately increased mortality . Loss of muscle strength in relation to atrophy, following DN, is well established. It is the general understanding that muscle biomechanical deficits in patients with diabetes are related to the presence and severity of DN. However, studies have documented impaired muscle function in patients with diabetes unrelated to DN also . The notion that muscle dysfunction in diabetes is affected by metabolic as well as neuropathic factors is gradually becoming more apparent. Still, the effects of diabetes and obesity on muscle function, irrespective of DN, are largely unknown.
Diabetic neuropathy and muscle impairment
Muscle strength
Preceding quantitative studies of muscle strength in patients with DSPN, muscle weakness, assessed by the presence of foot drop, was evident in a very small proportion of patients with diabetes (6% and 1% of patients with type 1 and 2 diabetes, respectively). Consequently, motor deficits were believed to occur only in relation to more severe DSPN . In a large-scale study applying manual muscle testing of elderly people (>70 years), Hiltunen et al. observed decreased muscle strength in participants with diabetes compared to those without diabetes at the upper extremity (thenar and interosseous muscles) as well as the lower extremity (tibialis anterior, tibialis posterior, and peroneus). In contrast to earlier studies, these findings suggested a more widespread muscle impairment in patients with diabetes .
Subsequent studies with quantitative muscle strength assessment support the observations by Hiltunen et al. Studies applying isokinetic dynamometry of extensor and flexor muscle groups have shown the loss of muscle strength in patients with type 1 as well as type 2 diabetes at both ankle and knee level . In all studies, muscle strength proximally as well as distally proved to be related to the presence and severity of DSPN. Notably, neither in patients with type 1 nor type 2 diabetes did muscle impairment independently relate to the degree of other chronic diabetic complications (retinopathy or nephropathy) . In Stouge et al., plantar flexors had the largest reduction in muscle strength (−38%), based on the standard deviation from the expected strength of healthy reference material, followed by the knee extensors (−27%) and dorsiflexors (−19%) . Muscle weakness of knee flexors in patients with DSPN did not significantly differ from healthy subjects after correction for multiple testing. In line with Stouge et al., Andersen proved that plantar flexors were weakened at least to the same degree as the dorsiflexors . These findings challenge the belief that the dorsal flexors are more prone to muscle damage following DSPN. Rather, foot drop may not be a consequence of more severe involvement of the dorsal flexors, but a consequence of dorsiflexors being a relatively smaller muscle group more easily resulting in clinically detectable deficits. Fig. 9.5 depicts the loss of muscle strength observed in flexors and extensors at knee and ankle level in patients with DSPN as compared to patients without DSPN and healthy subjects.
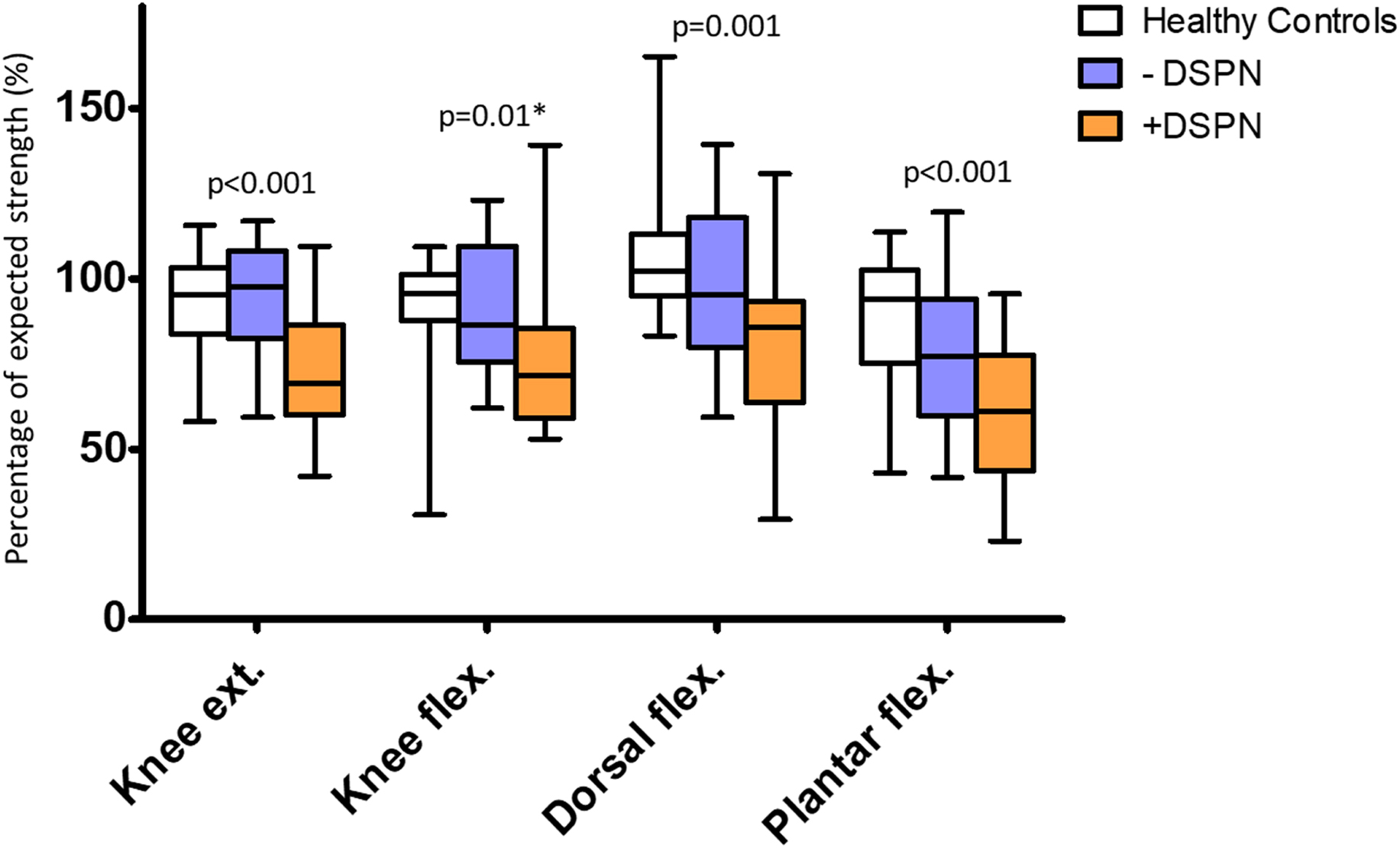
Interestingly, recent studies have suggested a larger involvement of proximal muscle groups than previously expected . The understanding of the pathology of DSPN with a clear distal–proximal gradient of sensory symptoms suggests a similar pattern should be detectable for motor symptoms. Indeed, previous studies of patients with severe DSPN have found that weakness of ankle muscle groups is more pronounced than at the knee level, supporting a distal–proximal gradient . In contrast, Almurdhi et al. observed a similar reduction in muscle strength between knee extensors (−32%) and plantar flexors (−34%) in a study group of patients with less severe DSPN . Proximal muscle deficits may partially be contributed to DSPN; however, metabolic factors of diabetes and insulin resistance per se may also contribute. In Stouge et al., multiple regression analyses proved that MRI detectable muscle changes at thigh level, including fat infiltration, to a higher degree were related to the loss of muscle strength and higher BMI than DSPN per se, supporting the assumption that additional factors, irrespective of DSPN, may be of importance for muscle impairment in diabetes . Thus a clear distal–proximal gradient of motor deficits may not be evident until later stages of DSPN.
In a 7–8 years follow-up study, patients with type 1 and 2 diabetes and DSPN, enrolled in the initial studies by Andersen et al. , were observed to have accelerated the loss of muscle strength as compared to patients without DPSN and healthy controls. This highly suggests motor dysfunction in diabetes to be a progressive disorder . In a subsequent study, a more than 50% reduction in the muscle volume of the lower leg was observed during a follow-up period of 9–12 years in patients with type 1 diabetes . Thus, in later stages of DSPN, progressive loss of muscle strength seems primarily to be due to accelerated muscle atrophy rather than decreased muscle quality. Recent MRI studies of patients with type 2 diabetes and DSPN support this notion with clear findings of fat infiltration secondary to atrophy of the lower extremity .
Muscle strength at the upper extremity is affected to a much lesser degree . This is not surprising considering the distal–proximal gradient of DSPN. Nevertheless, in patients with type 1 diabetes, muscle strength of wrist flexors has been found to be associated with the severity of DSPN .
Muscle power
In addition to the loss of muscle strength, patient may elicit reduced muscle power related to the degree of DSPN. Muscle power is defined as the product of muscle strength and muscle contractile velocity (work per unit time), and since daily living involves dynamic muscle action, loss of muscle power could have a greater impact on functional muscle limitation than muscle weakness per se . Hilton et al. observed a greater reduction in power (−73% to −86%) in plantar flexors and dorsiflexor in obese patients with type 2 diabetes as compared to the loss of muscle strength alone (−41% to −79%) . Allen et al. found lower strength of dorsiflexors (−35%) as well as slower contractile properties for both voluntary (−48%) and evoked contractions (−40%) (percutaneous electrical stimulation of the fibular nerve) in patients with type 2 diabetes and DSPN . Patients with DSPN depicted similar ability as healthy subjects to activate their muscles (>95%). However, slower contractile properties were observed with decreased twitch average rates of rise (−40%, Nm/ms), prolonged contraction duration due to a combination of longer time to peak tension and half-relaxation time (+22%, ms), as well as the decreased maximal rate of voluntary torque development (−48%, Nm/s). Contractile impairment and slowing seem to explain the greater power reduction observed in patients with diabetes than what is explained from loss of muscle strength alone. Fig. 9.6 clearly depicts the impairment of muscle power properties observed in patients with DSPN in addition to the loss of muscle strength. Similarly, Sacchetti et al. evaluated isometric and isokinetic contractions at different angular velocities at both the upper and lower extremity in a mixed group of patients with type 1 or type 2 diabetes . Patients were divided into two groups based on the NCS of the peroneal nerve. Isometric strength proved similar between groups of diabetes and healthy sedentary subjects, whereas dynamic muscle strength was lower in patients with diabetes and proved more pronounced at higher angular velocities. Moreover, isokinetic muscle strength was lower in patients with motor nerve dysfunction than patients without nerve dysfunction, correspondingly increasing with angular velocities. These findings suggest muscular deficits in patients with diabetes is best visualized by dynamic muscle work. The slowing of skeletal muscle contractile properties may be affected by both myogenic and neurogenic factors. A preferential loss of type 2 muscle fibers has been suggested as an explanation with collateral reinnervation of type 1 motor neurons. This is supported by findings of inverse correlations between reduced MUNE measures and prolonged twitch half-relaxation time of dorsiflexors . Other potential explanations include changes in the excitation–contraction coupling and Ca 2+ , or alterations in muscle morphology and structure with fascicular disruption reducing the numbers of sarcomeres and hereby contractile velocity . Still, the assessment of muscle power in patients with diabetes is sparse, and the association to DSPN is poorly understood and mostly limited to studies on dorsal and plantar flexors.
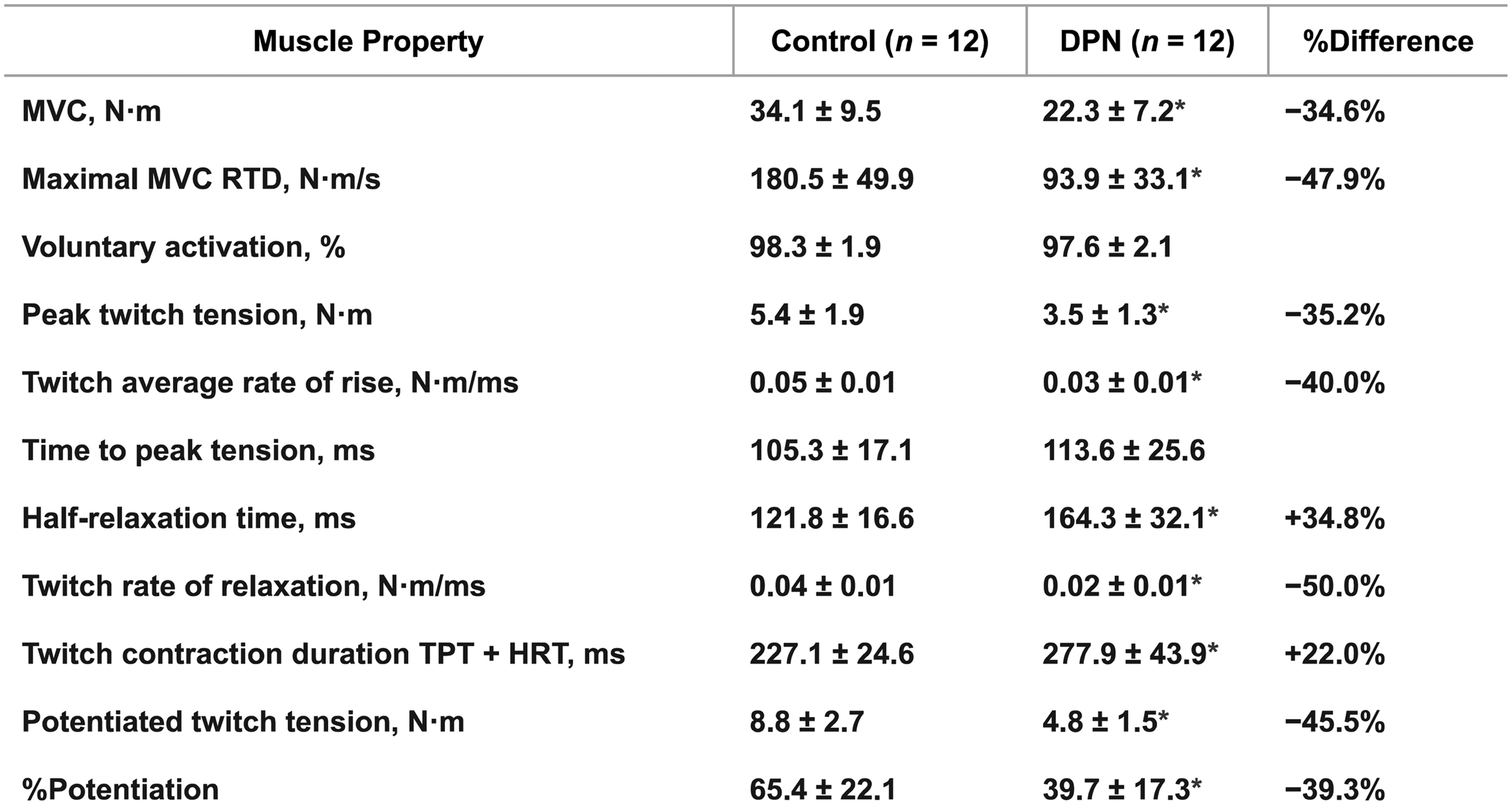
Muscle endurance
The understanding of muscle endurance in patients with diabetes is limited due to few studies and contradictory results. A recent study observed decreased muscle endurance of dorsiflexors in a small population (10 participants) of patients with type 2 diabetes and DSPN . Patients with DSPN fatigued 21% more quickly than healthy control subjects performing a sustained isometric contraction, despite equal levels of voluntary muscle activation. Maximal twitch torque immediately following fatigue decreased to a similar degree in patients with DSPN and healthy controls (−20%). In DSPN patients, this was further followed by a 12% reduction in CMAP. Both in healthy participants and patients with DSPN muscle strength recovered by 2 minutes postfatigue. In contrast to the findings of Allen et al., a previous study did not find that weakness of the extensors and flexors at the ankle and knee was accompanied by lower muscular endurance . In fact, following 30 maximal reciprocal isokinetic movements, the endurance ratio was higher for patients with type 1 diabetes. It has been suggested that metabolic changes and alterations in muscle fiber type composition (more type 1 fibers) could explain this finding, favoring less fatigability. In contrast to Andersen et al., participants in Allen et al. elicited a larger degree of DSPN, and measures of voluntary muscle activation were included.
Muscular endurance in patients with diabetes is still not well understood. The contradictory findings in the above studies may be explained by the different protocols and study population assessed. Furthermore, fatigue may primarily be a neurogenic property with neuromuscular transmission failure and therefore may not be present unless a relatively severe degree of DSPN is observed. Future studies should examine fatigue in patients with diabetes applying both dynamic and isometric conditions. Other explanatory factors have also been suggested, including muscle oxygen kinetics and microvascular dysfunction .
Respiratory muscle function
In diabetes, respiratory muscles may be impaired in patients with DSPN . Applying nerve stimulation of the phrenic nerve, reductions of inspiratory and expiratory pressures were related to the severity of DSPN assessed by a neurological disability score. Whereas patients without DSPN, based on clinical examinations, were found to have normal pressures. Emerging evidence suggests that diabetes is associated with decreased pulmonary function . Axonal loss within the phrenic nerves may contribute to the more adverse outcome following admission to the intensive care unit in patients with diabetes. Still, only a very limited number of studies exist evaluating the effects of DSPN on respiratory function, and a possible relation remains largely unknown.
Muscle impairments in diabetes independent of neuropathy
The population-based health, aging, and body composition study by Park et al. evaluated 2618 participants aged 70–79 years , of which 485 had type 2 diabetes. Quantitative muscle strength was evaluated by applying isokinetic dynamometry of the knee extensors and isometric dynamometry of grip strength. In addition, dual-energy X-ray absorptiometry was included for the evaluation of lean body mass of the lower and upper extremity. Men with type 2 diabetes exhibited lower muscle strength, and in both genders, patients with diabetes had lower muscle strength relative to the amount of muscle mass, entitled muscle quality (strength per unit muscle mass). In line with the emerging belief of a general muscle dysfunction in patients with diabetes, irrespective of DSPN, the authors reported that reduced muscle quality was related to the duration of diabetes and poor glycemic control (HbA 1c >8%). Unfortunately, assessment of peripheral nerve dysfunction was not included, and therefore the relation to DSPN is unknown. Even though the difference in muscle quality was statistically significant, the magnitudes were small (7%–8% reduction of leg and arm muscle quality in both men and women with diabetes), and the variation between subjects was considerable. A similar study evaluated 874 Asian participants, of whom 49% were known to have diabetes . The authors observed a threefold increase in the prevalence of sarcopenia, defined as a skeletal muscle index (total skeletal muscle mass/total body weight) at least 2 SDs lower than a reference population, of patients with diabetes as compared to participants without diabetes. These observations were evident after correction for age, gender, smoking, alcohol, physical activity, medications, and BMI. In addition, patients with diabetes had lower muscle quality (i.e., strength per unit of muscle mass) although they did not have higher BMI, which was in contrast to Park et al. This difference may be due to ethnic differences.
A 3-year follow-up reevaluated 70% of the participants from the initial studies of Park et al. . During follow-up, a considerable reduction (−15%) in strength of knee extensors was observed in patients with diabetes compared to participants without diabetes. Muscle mass declined only by −4%, resulting in a considerable reduction in muscle quality. The rapid decline of leg muscle strength and quality was significant even after correction for the influence of ethnicity, gender, physical activity, drinking, smoking, and other chronic disorders. As in the initial study, no examination of peripheral nerve dysfunction was performed. Since the increased loss of muscle strength was only observed in the lower extremity and not in the upper extremity, DSPN may have contributed to the accelerated loss of muscle strength. These large-scale studies suggest that diabetes, irrespective of DSPN, is related to reduced muscle quality and a greater degree of sarcopenia than what would be expected from age-related sarcopenia alone.
Studies assessing the association between diabetes and muscle impairment have largely focused on functional muscle deficits assessing gait and walking speed, with limited knowledge of the implications and presence of alterations in quantitative muscle strength . Volpato et al. found that impaired quantitative strength measures in the lower limb, including lower muscle strength and quality, were important contributing factors to functional muscle deficits assessed by gait speed . The study included 835 randomly selected participants at the age of 65 or older, of whom 11% had type 2 diabetes. Muscle strength of eight muscle groups, including knee extensors, plantar flexors, and dorsiflexors, was assessed using a handheld dynamometer. Furthermore, peripheral quantitative computerized tomography was applied for muscle volume and fat infiltration calculation based on a cross-sectional area of the calf. The study included NCS of the superficial peroneal nerve. In a multivariable analysis, differences in muscle strength, muscle quality, and walking speed between participants with and without diabetes remained significant even after adjusting for peripheral arterial disease and motor deficits assessed by the peroneal nerve. This underscores that diabetes per se has a detrimental effect on muscle function.
Hyperglycemia and lower insulin sensitivity may independently or combinedly contribute to muscle impairment in diabetes patients. Nomura et al. found that muscle strength of knee extensors was inversely related to insulin resistance in type 2 diabetes involving both patients with and without DSPN . Yet, although none of the participants had severe DSPN, these findings may still be suspected to be influenced by DSPN. In a cross-sectional study on 1391 participants, Sayer et al. reported that muscle strength of the wrist, assessed by grip strength, was lower not only in patients with known diabetes but in participants with impaired glucose tolerance as well. These findings extended across the normal range of glucose concentration for men and remained significant even following adjustment for physical activity . In support of Sayer et al., a study examining a population of nondiabetic otherwise well-functioning older adults found that decreased strength per kilogram of muscle mass of knee extensors was inversely related to the degree of insulin resistance . These findings not only suggest that insulin insensitivity is an independent risk factor of muscle impairment in diabetes, but also further imply that muscle deficits may occur prior to the diagnosis of diabetes. Few studies have examined muscle function in relation to hyperglycemia, and further studies are needed to conclude if hyperglycemia, independently of insulin resistance, leads to lower muscle quality.
A range of other patient characteristics, inevitable in patients with diabetes, for example, obesity, low physical activity, and higher age, may also independently and synergistically impair muscle function in patients with diabetes. Age-related sarcopenia is related to loss of lean muscle mass with atrophy of the individual muscle fibers as well as decreased muscle quality . Age- and diabetes-related muscle dysfunction shares several common mechanisms, with diabetes accelerating the decline in muscle function seen with ageing . Similarly, synergistic effects of obesity and age-related sarcopenia have been observed in the elderly population . In addition, in the study by Volpato et al., although findings of reduced muscle quality remained significant, an attenuation of the observed lower muscle strength and higher fat infiltration in patients with diabetes was observed after adjustment for BMI. A possibly independent effect of obesity on muscle strength and function could be expected but is as of yet largely unknown.
In summary, studies of large cohorts have documented that diabetes and impaired glucose tolerance have a negative impact on skeletal muscle function, irrespective of DSPN, with observations of reduced muscle strength, lower muscle quality, and accelerated sarcopenia.
Muscle strength, balance, gait, and falls
The risk of falling relies heavily on postural control. Muscle weakness, reduced power, and muscle endurance may all independently and synergistically contribute to functional impairment with gait instability, altered balance, and ultimately increased risk of falling. Moreover, falls and impaired balance are major contributors to overall morbidity and mortality in the elderly population . Indeed, multiple studies have shown that patients with diabetes are at increased risk of falling , and this risk is expected to further increase with the presence of DSPN . Several studies have linked DSPN with postural instability and poor balance . Moreover, gait instability has been observed with altered walking pace, a wider stance, and larger movements in the anterior/posterior plane as well as laterally . Impaired neuromuscular control, following the loss of motor units and incomplete reinnervation with subsequent increased motor unit size, has been suggested to explain functional muscle impairment . Motor unit alterations following DSPN could affect the final fine-tuning and grading of motor-outputs. Moreover, proposed slowing of muscle contractile properties and impaired proprioception following DSPN challenges the reaction response to sudden change of movements or positions in daily living . Fear of falling is suspected to have a major impact on daily life in patients with diabetes, expected to lead to restriction of daily physical activity, with decreased mobility, isolation, and reduced quality of life ; however, studies are lacking evaluating the impact of fear of falling on daily living and quality of life.
Postural stability is a complex skill, which cannot be explained by alterations in muscular function alone . Further discussion of additional factors, for example, somatosensory deficits, visual and vestibular function, and altered joint mobility, is considered outside the scope of this chapter.
Muscular composition and structure
Introduction—technical advances and muscle composition
Technical advances in imaging modalities have enabled improved visualization of skeletal muscle tissue and thereby provide objective and reliable measures for skeletal muscle compositional and structural assessment. Both ultrasonomyography, X-ray computed tomography (CT) and MRI, have been applied for imaging of skeletal muscles. MRI is the preferred option, and by far the image modality most extensively covered in the literature, providing detailed high-resolution images of muscle tissue with clear separation of muscle tissue from noncontractile tissue, for example, fat and connective tissue . Emerging quantitative MRI parameters are increasingly considered valuable in diagnosis as well as monitoring of disease progression or treatment response of neuropathic and muscular changes following neuromuscular diseases . Concerns regarding the extension of MRI to the clinical setting are (1) costs, (2) time required, and (3) for practical and safety reasons, the limited ability to evaluate very obese subjects and patients with pacemakers, claustrophobia, and metal implants. Methodology of newer MRI studies of skeletal muscles includes time-optimization with faster MRI spin-sequences and semiautomated MRI data-analysis programs . Furthermore, such protocols have proven clinically feasible even in obese patients with type 2 diabetes . CT has higher spatial resolution compared with MRI, but lower contrast resolution of soft tissue involves ionizing radiation exposure. CT is, therefore, seldomly used for the evaluation of skeletal muscles. Advantages of ultrasonomyography compared to MRI and CT are low-cost equipment and the possibility for bed-side evaluation, whereas drawbacks of ultrasonography include lower resolution and high observer dependency with lower interrater reliability of skeletal muscles.
Clinical assessment of muscle atrophy
Clinically perceptible changes following atrophy are predominantly related to the feet, including a reduction in the bulk of the extensor digitorum brevis muscle . Other signs include prominent extensor tendons and metatarsal heads, and foot deformities with pes cavus and clawing of the toes. Such changes may lead to callus formation, which are easily detectable and often developing at high-pressure sites. As atrophy progresses more proximally, the lower legs will be involved showing reduced bulk of the calf muscles and a more prominent anterior border of the tibial bone. Atrophy of small hand muscles is an infrequent finding, and if found in isolation, can often be attributed to hypoglycemia-induced neuropathy . Clinical assessment of muscle atrophy is very insensitive with distinct changes only in severe cases of DSPN. Obviously, clinical assessment does not allow for the evaluation of compositional and structural muscle changes.
MRI for evaluation of skeletal muscles in patients with diabetes has, and still is, primarily applied in the experimental setting. The clinical application of MRI in diabetes is limited to evaluation and diagnosis of the presence of Charcot of the feet . Less common, MRI can be useful in diabetic patients with complaints of acute muscle pain or if there is soft tissue swelling at the clinical examination. In particular, MRI can be used to visualize vascular properties of diabetic myopathy, such as muscle ischemia and infarction with necrosis of muscle tissue, which can be observed in patients with long-standing diabetes .
Foot muscle atrophy—an early sign of motor deficits in DSPN
Consistent with the distal–proximal gradient of DSPN, abnormalities of the feet with the loss of foot muscle bulk are early signs of atrophy following motor neuropathy in DSPN. Several MRI studies have indeed reported the loss of small foot muscles with more than a 50% reduction of muscle volume in patients with DSPN . In fact, atrophy of foot muscles is even observed in patients without clinical signs of DSPN, underlining the presence of motor changes in DSPN well in advance of clinical findings and complaints . Similar findings have been observed applying ultrasonography with reduced muscle volume in both neuropathic and nonneuropathic patients with diabetes . Diagnostic criteria of DSPN differ in the different studies, with Greenman et al. only assessing clinical signs of DSPN, in contrast to others also including NCS . Still, these findings suggest that atrophy of foot muscles occurs in earlier phases of DSPN. Normal ageing is associated with stagnation of the sensorimotor function with age-related atrophy of foot muscles . Still, DSPN clearly accelerates the age-related muscle abnormalities. Fig. 9.7 depicts the severe atrophy and fat infiltration observed in relation to DSPN in foot muscles as compared to an age-matched healthy control.
