Fig. 4.1
Workflow of molecular diagnostics from thyyroid nodules FNAB
For DNA studies, a dedicated FNAB material can be smeared on a microscope glass slide and air-dried or can be collected in a dedicated tube. Reliable results can be obtained also by washing out the needle with 3–5 ml normal saline in a collection tube after having prepared the smear for cytology [4]. The samples can be stored at room temperature for up to 12 h, but should be preferentially centrifuged, suspended in a small volume of normal saline (0.5–1 ml) and kept frozen at -20 °C until processed for DNA isolation.
For RNA studies, material from one dedicate FNAB must be collected in solutions that preserve RNA integrity, suspended in RNAse-free buffers, stored frozen, and processed as quickly as possible for RNA isolation. Samples collected for RNA studies can also be used for DNA studies, dividing the samples before nucleic acids isolation takes place.
4.2 Assessment of Molecular Abnormality in Cytological Sample
4.2.1 MicroRNA
MicroRNAs (miRNAs) are small non-coding 19–25 nucleotides long highly conserved RNAs that potently modulate gene expression by inhibiting mRNA transcription or translation [5, 6], possibly acting as oncogenes or tumor suppressor genes [7]. miRNAs can be isolated from formalin-fixed paraffin embedded (FFPE) tissues, with good yield and quality levels [8, 9], and from FNAB material, achieving suboptimal yield and quality but comparable results [10]. Differential miRNA expression profiles have been characterized in thyroid cancers, benign thyroid lesions, and normal thyroid tissue [11], indicating that miRNA profiles may effectively become useful in differentiating benign from malignant lesions. Indeed, upregulation of several miRNAs, such as miR-146b, miR-187, miR-221, and miR-222, has been shown in papillary thyroid cancers (PTC) [12, 13] while others, such as miR-197 and miR-346, are over-expressed in follicular thyroid cancer (FTC) [14]. miRNA expression has been found to differentiate between benign and malignant lesions with variable accuracy in cases of indeterminate cytology [15, 16].
Most of miRNA studies have been performed on frozen or FFPE tissues that warrant higher yield and better miRNA quality as compared to FNAB samples. Therefore, the results should be regarded with caution and need to be validated in large, prospective, blinded, multicenter studies.
4.2.2 Gene Mutations
The identification of somatic point mutations in FNAB material represents a powerful method to rule in thyroid malignant nodules, being feasible in routine air-dried smears or in syringe wash out material from FNAB. BRAF and RAS somatic heterozygous mutations are indeed identified by several different methods starting from FNAB-derived DNA with good sensitivity and accuracy [1, 17–29].
BRAF gene, located on chromosome 7q, encodes a serine/threonine kinase belonging to the family of RAF proteins, taking part to the MAPK transduction pathway [30]. BRAF mutations, the most common mutations in PTC, are associated with constitutive activation of MAPK pathway and lead to follicular thyroid cell transformation. Their prevalence depends on the evaluated population, varying from 14 to 83 % of PTCs [31–34]. The BRAF V600E mutation at exon 15 is highly specific, since it almost always coincides with the presence of a PTC, representing a molecular marker for PTC and PTC-derived cancers. This mutation has been found to associate with recurrent disease, aggressive behavior and reduced iodide up-take [35], indicating a poorer prognosis [36, 37]. However, further investigation highlighted that BRAF V600E mutation is more frequent in older patients, may be found in metastatic lymph nodes but not in primary tumors [38], suggesting that BRAF mutations are not the main determinant for PTC morbidity and mortality. This aspect has been addressed in retrospective studies [39, 40], but prospective studies are still lacking.
RAS genes encode for a family of G-proteins participating in the MAPK and PI3K/AKT signaling pathways. The most common somatic mutations occur at codon 12 and 13 of the N-RAS and K-RAS isoforms and at codon 61 of the H-RAS isoform, being more frequently associated with FTC, follicular adenomas and, more rarely, with follicular variant of PTC [37]. The false-positive rate is high, since RAS mutations have been found in thyroid nodules that turned out to be benign lesions at final histology. Therefore, the diagnostic sensitivity of FNAB for thyroid cancer is often not improved by RAS mutation analysis [41]. However, RAS mutations may associate with malignant transformation of follicular adenomas into FTC, pushing mutated thyroid cells towards dedifferentiation and aggressive behavior [42].
4.2.3 Gene Translocations
Gene translocations can be investigated in FNAB material, after isolation of total RNA from the sample. Therefore, accurate and reliable analysis requires carefully collected and stored samples.
The RET gene, an acronym for REarranged during Transfection, is located on chromosome 10q11.2 and encodes for a tyrosine kinase receptor, which, in turn, is involved in the regulation of several cellular key functions [43]. Several chromosomal rearrangements involving the 3′ end of RET, corresponding to the C-terminal kinase domain, and the 5′ end of unrelated genes, leading to a constitutive activation of the kinase, have been described [44]. Among RET/PTC rearrangements, the most common are RET/PTC1 and RET/PTC3, the latter being more frequent in radiation-induced PTC [45]. The presence of a RET/PTC rearrangement does not invariably predict the presence of a cancer, since these rearrangements can be detected also in benign lesions, with a prevalence of 13.9–52.4 % in benign neoplasms, 10–20 % in PTC and ~20 % in follicular variant of PTC, while they have not been reported in FTC [46]. Therefore, the diagnostic value of RET/PTC rearrangement in FNAB material is limited, even though it has been reported that it might be helpful in the settings of FNAB with indeterminate cytology [24, 47]. A meta-analysis showed that RET/PTC rearrangement testing has a low sensitivity and an average accuracy [48], therefore, RET/PTC rearrangements detection does not improve the high diagnostic accuracy for malignancy of BRAF mutation testing. Indeed, the latter has a very high positive predictive value, even in indeterminate samples, which is not enhanced by simultaneous testing for both RAS mutations and RET/PTC rearrangements. On the other hand, in indeterminate lesions corresponding to atypia of undetermined significance the lack of alterations in all these three genetic markers corresponds to a very low cancer risk, thereby indicating a very high negative predictive value for the combination of the three molecular investigations [49].
Many differentiated functions of thyrocytes such as those mediated by thyroglobulin, sodium-iodide symporter and TSH receptor are profoundly influenced by the paired box gene 8 (PAX8), located at chromosome 2 [50, 51]. Peroxisome proliferator-activated receptor gamma (PPARγ), an orphan nuclear hormone receptor, is involved in the regulation of carbohydrate and lipid metabolism and is transcribed from a gene located on chromosome 3. The chromosomal translocation t(2;3)(q13;p25) results in the fusion of the two genes, called PAX8/PPARγ rearrangement, which encodes for a fusion protein that, in turn, activates the PI3K/AKT pathway and is involved in thyroid tumorigenesis [52]. However, the presence of this rearrangement is not diagnostic for cancer, being identified also in benign lesions (range 0–13 %), possibly characterizing evolutive lesions [53], on the contrary a higher frequency has been detected and associates with a more favorable prognosis in FTC (up to ~40 %) [54, 55]. The PAX8/PPARγ rearrangement has not been described in PTCs. Therefore, this test may be useful when assessing cytologically indeterminate lesions, in that, when the rearrangement is detected, an accurate evaluation of the nodule capsule and vessels by the pathologist is suggested [54]. Testing for PAX8/PPARγ rearrangements, therefore, is burdened by a low sensitivity, but seems to have a high positive predictive value [48].
4.2.4 Gene Amplifications
Among the potential molecular biomarkers that can be assayed on FNAB material for diagnostic purposes, also gene amplifications should be considered. An exemplification is represented by PI3KCA gene amplification. Indeed, in addition to activating mutations, PI3KCA gene amplification has also been implicated in cancer, for example, in ovarian and cervical cancers [56, 57]. The copy number gain is associated with increased cell growth and decreased apoptosis. The frequency of PI3KCA mutations and gene amplification was investigated in thyroid carcinomas and appeared to be higher in follicular and in anaplastic thyroid carcinoma, rather than in papillary thyroid carcinoma [58–61]. Significantly higher expression levels of PI3KCA gene were detected in cytological samples of the PTC group in comparison with the nontoxic multinodular goiter group (P < 0.05) [62]. Wu and colleagues described a strong association between PI3KCA copy number gain and high-risk clinic-pathological features in thyroid tumor, suggesting a potential role of that amplification in thyroid cancer progression [63]. Indeed, further investigations are needed to determine the definitive role of PI3KCA gene in thyroid cancer pathogenesis and progression and to clarify its role of molecular biomarker in nodule diagnostic procedures.
4.2.5 Gene Expression Variation
Unnecessary thyroid surgery could be avoided with a more accurate preoperative diagnosis of nodules based on the analysis of gene expression profile variations.
Real-time PCR (RT-PCR), as well as microarray studies have been employed to assess different gene expression profiles in benign and malignant lesions. For instance, after demonstrating the usefulness of six marker genes for the molecular distinction of benign and malignant thyroid tumors at the surgical specimen level, Karger and colleagues performed RT-PCR for ADM3, HGD1, LGALS3, PLAB, TFF3, and TG in cytological material in a prospective series of cancers with proven histological result, to investigate whether these markers could be applied for the differential preoperative diagnosis. HGD1 and TFF3 expression levels were significantly lower in FNAB of thyroid cancers compared with those of benign nodules and normal thyroid tissue. Conversely, ADM3 and LGALS3 expression levels were significantly higher in FNAB of thyroid cancers compared with benign lesions. Using two-marker gene sets, it was possible to distinguish between benign and malignant thyroid cancers with a negative predictive value (NPV) of up to 78 % and a positive predictive value (PPV) of up to 84 % [64].
Similarly, the mRNA expression level of other 9 genes (KIT, SYNGR2, C21orf4, Hs.296031, DDI2, CDH1, LSM7, TC1, NATH) was tested in cytological specimen. A computational model was developed (i.e., Neural Network Bayesian Classifier) and a multiple-variable analysis was performed to assess the correlation among the markers. KIT, CDH1, LSM7, C21orf4, DDI2 expression levels were significantly different between benign and malignant tumors (P < 0.05) and the neural network classifier, constituted by the markers used in the most predictive model along with BRAF mutational status, achieved a predictive power of 88.8 % [65].
4.2.6 Aberrant Gene Methylation
Among the potential molecular biomarkers that can be assayed on FNAB material for diagnostic purposes, also DNA methylation should be considered. Indeed, gene promoter methylation is one of the most common epigenetic alterations in human cancers, including thyroid carcinoma [66]. It is known that promoter hypermethylation usually occurs in cancer cells in order to inactivate tumor suppressor genes [67].
In a recent paper, Zhang and coworkers demonstrated the feasibility of DNA methylation assay in FNAB material and defined specificity and sensitivity of a panel of methylation markers for evaluating thyroid nodules. In detail, they performed quantitative methylation-specific PCR (Q-MSP) to determine differences between PTC and benign nodules in the methylation levels of five genes, including CALCA, DAPK1, TIMP3, RAR-beta, and RASSF1A. These genes were selected based on their documented relevance in thyroid cancer [68]. They found that in PTC methylation level of DAPK1 was significantly higher than that in benign samples (P < 0.0001). Conversely, in PTC methylation level of RASSF1A was significantly lower than that in benign samples (P < 0.003). However, their diagnostic specificity and accuracy were low. The authors also investigated the relationship between BRAF mutation and gene methylation levels. BRAF mutation was significantly associated with DAPK1 methylation (P = 0.0009), as well as with absence of RASSF1A methylation (P = 0.026). Moreover, combined analysis of BRAF mutation and methylation markers increased the diagnostic sensitivity, accuracy and also specificity of the molecular test [69].
DNA methylation markers alone, therefore, appear to have a limited usefulness in the diagnosis of thyroid cancer; however, by exploiting together with other molecular markers could improve the diagnostic accuracy of FNAB.
4.2.7 Veracyte Afirma Gene Expression Classifier (GEC): Analytical and Clinical Value
The search for tools to predict benignity of cytological indeterminate thyroid nodules and to potentially avoid surgery on these nodules, has elicited the development of a gene expression classifier (GEC) test (Afirma®, Veracyte, Inc., South San Francisco, CA) that can be run on the FNAB sample. The Afirma GEC is an RNA-based assay designed to evaluate the gene expression profile of a given thyroid nodule by comparing it with the panel of 167 mRNA expression patterns (derived from 142 genes identified originally from the Affymetrix Human Exon Array) associated with benign nodules. It is characterized by a high sensitivity (90 %) in indeterminate cytology lesions to identify the signature of a benign thyroid nodule with 95 % NPV: that is similar to the risk of malignancy in a resected thyroid nodule with a preoperatively benign FNA cytopathology diagnosis [3, 70]. Specificities of the test were low, as expected, at 53 % for AUS/FLUS and 49 % for FN/SFN. NPV drops to 85 % in the case of a suspicious for malignancy cytology. Veracyte currently requires 2 sets of FNA samples: one for cytological evaluation and one for running the gene expression profiling (the Afirma GEC test). The patient’s FNA sample is subjected to standardized cytological evaluation by the company’s independent cytopathology examination at the thyroid cytopathology partners (TCP; Austin, TX). Cytological results are based on the Bethesda classification. FNA samples with Bethesda categories of benign, malignant or suspicious for malignancy do not undergo further evaluation with Afirma GEC and only the cytological diagnosis is forwarded to the ordering physician. For cytological samples with the diagnosis of AUS/FLUS/FN/SFN, clinicians should expect to receive a 2-part report from Veracyte: cytological evaluation and the GEC test result. The Afirma GEC sample undergoes RNA extraction and nucleic acid amplification. Processed Afirma GEC samples are hybridized to a custom Afirma Thyroid Microarray and analyzed with a classification algorithm using linear support vector machine logic [71]. The GEC test result is binary and reported as benign or suspicious if the samples were adequate. The genetic material is first put through 6 different cassettes before applying the final benign versus malignant classifier. The first 6 cassettes act as filters for rare subtypes of thyroid cancer and nonfollicular cell-derived tumors (malignant melanoma, renal cell carcinoma, medullary thyroid cancer, and parathyroid tissue), which prevent the material from being scored by the main thyroid classifier. The test has a false-negative rate of 5–15 % and, therefore, a new assessment is important if the nodule enlarges or clinical suspicions arise. Furthermore, a PPV for a suspicious test result is only 38 %. Two prospective multicenter studies evaluated the negative predictive value (NPV) for the Afirma GEC, which is the key diagnostic performance metric used to make a decision to monitor patients in lieu of referral for diagnostic thyroid surgery [3, 70]. Both studies utilized diagnosis of the surgical pathology specimen by a central panel of blinded academic endocrine histopathologists as the reference standard for clinical validation [71]. In one of these studies, the rate of malignancy was nearly equivalent in AUS/FLUS and FN/SFN (24 % vs. 25 %) [3]. This rate is not representative of the general risk of malignancy observed for AUS/FLUS lesions (5–15 %). Thus, the AUS/FLUS groups in the studies may not have been representative of the general population. This observation opens the issue of the need for more studies aimed at extending the evaluation of the analytical performance of Afirma GEC on much larger population layers in an international setting. Moreover, the high rate of false-positive results and not the negligible rate of false negatives narrows the indications of the test, cutting away the suspicious for malignancy cytology, burdened by a too high risk of malignancy making a false-negative rate of 5–15 % unacceptably high, and the nonsuspicious AUS/FLUS, where the risk of malignancy (5–15 %) is equivalent to the false-negative rate and the high false-positive rate may confound the evaluation and management of benign nodules [2].
4.3 Impact on Diagnosis
The availability of molecular markers has improved the diagnostic performance of FNAB in many settings. The major diagnostic contribution is expected in the group of cytological indeterminate nodules, where the available tests may be helpful in ruling in (mutation panels) or ruling out (GEC) thyroid cancer. This approach should ideally allow to avoid surgery in nodules that are benign, and to indicate therapeutic (and not diagnostic) surgery when the nodule is malignant. However, the currently available molecular tests have failed to offer both high sensitivity and high specificity. GEC demonstrated high sensitivity and high negative predictive value in samples having a low risk of malignancy (the “rule out” option). This approach would allow applying a conservative follow up and avoid diagnostic lobectomy for nodules, which are very likely to be benign follicular hyperplastic nodules, sparing resources and securing patients quality of life. On the other hand, this approach has a low specificity, and therefore is not optimal for identifying cancer. Conversely, mutation panels have a high specificity and positive predictive value (the “rule in” option) for cancer, allowing identifying malignant nodules to be addressed to therapeutic surgery. However, a negative test does not correspond to the absence of cancer, indicating the need for diagnostic surgery in the presence of an indeterminate cytology [72]. Ideally, both “rule in” and “rule out” testing should be performed when cytology is not informative, but the cost of this approach may override the clinical benefit for the patient.
Molecular testing has also been demonstrated to increase diagnostic sensitivity and accuracy for thyroid cancer in cytological benign nodules [4, 18], allowing anticipating the diagnosis of thyroid cancer through the detection of malignant lesions at a lower disease stage [49]. Most of the gathered experience is related to the BRAF mutation, whose detection by solid and validated techniques is strongly associated with PTC. Several reports indicate that diagnosis of thyroid cancer can be significantly improved by simultaneous testing for multiple mutations, as stated above, such as BRAF and RAS mutations as well as RET/PTC and PAX8/PPARγ rearrangements [47, 72, 73]. The identification of one of these genetic derangements may help in characterizing the risk of malignancy of a thyroid nodule, allowing a more accurate risk stratification for the patient and assisting the clinician in choosing patient management, especially in the settings of a nodule with indeterminate cytology. However, it must be underlined that more recent evidence indicates that BRAF analysis is superior to other mutation tests, such as RAS mutations and RET/PTC rearrangements, even when performed in combination [49], for the diagnosis of PTC. These discrepancies may be due to the different inclusion criteria employed in the sample selection (i.e., the investigated thyroid nodules) in the different studies, which are linked to a different pre-test probability of being a cancer.
The diagnostic impact of genetic derangements needs to be validated by prospective multicenter clinical studies, also assessing analytic validity and clinical utility as well as cost-effectiveness.
4.4 Impact on Surgical Choice
Molecular markers assayed on FNAB material might also harbor a prognostic significance and be useful to define the more appropriate surgical strategy according to the estimated aggressiveness of the lesion.
In particular, genetic markers – including mutations in RAS, PIK3CA, AKT1, PTEN, p53, CTNNB1, ALK, and TERT genes – show promise [74, 75]. However, the best-defined prognostic marker for thyroid carcinomas, namely PTC, is BRAF mutation [39, 40, 76–81].
The high NPV for recurrence of a BRAF negative test has suggested its use in clinical practice, especially in the preoperative planning where it can help to determine the extent of initial surgery [74].
In the absence of clinical and sonographic lymph node involvement, the accomplishment of a prophylactic central compartment lymph node dissection in all thyroid cancer surgeries is controversial [82]. Several studies have shown that prophylactic central compartment lymph node dissection reduces postoperative thyroglobulin levels and may decrease local regional recurrences. However, no study has demonstrated a reduction in disease-specific mortality [83–86]. Moreover, prophylactic central compartment lymph node dissection has been associated with a higher rate of postsurgical hypoparathyroidism and laryngeal nerve damage even when the procedure was performed by high volume surgeons [85]. In PTC, BRAF mutation has appeared as a significant predictor of central compartment lymph node metastasis on both univariate (OR 8.4, P = 0.01) and multivariate (OR 9.7, P = 0.02) analyses [87, 88]. Thus, preoperative BRAF mutation detection has been suggested to be one way to identify PTC patients who would best benefit from prophylactic central compartment lymph node dissection [75]. Several studies have also reported that BRAF mutation positive papillary thyroid microcarcinomas (PTMC) are characterized by higher rates of extrathyroidal tumor extension and cervical lymph node metastasis [89, 90]. Although the majority of PTMC are indolent, a subset of these tumors can behave aggressively leading to recurrence and mortality. Very recently, Niemeier and coworkers developed a molecular-pathological (MP) score for PTMC that included BRAF status and three histopathological features: superficial tumor location, intraglandular tumor spread/multifocality, and tumor fibrosis. This risk stratification model appeared a good predictor of extrathyroidal tumor spread and tumor aggressiveness measured as lymph node metastases or tumor recurrence [91]. In this context, a positive preoperative testing for BRAF mutation in a PTMC might induce a change in the surgical strategy moving from the American Thyroid Association-recommended lobectomy to a more radical total thyroidectomy [74]. However, there is still no prospective evidence that this approach will favorably change the outcome of these low-risk patients.
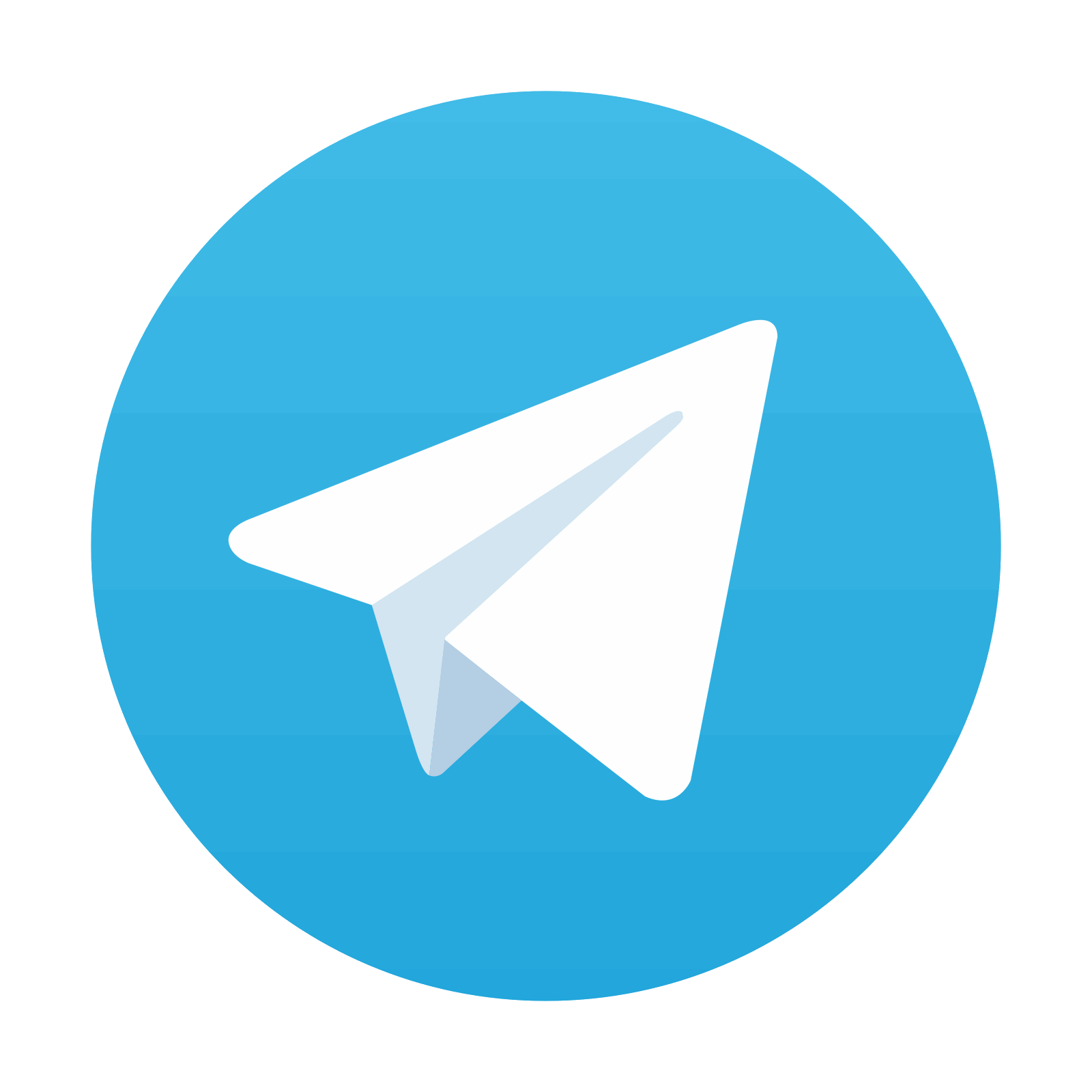
Stay updated, free articles. Join our Telegram channel

Full access? Get Clinical Tree
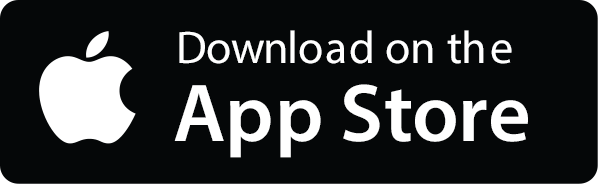
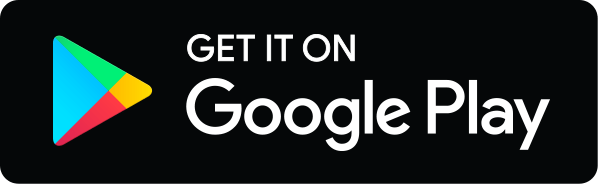