Detection method
Patient status
Remarks
Total no. of patients
n (%)
Concordance (%)
Reference
DTC+, DTC+
DTC+, DTC−
DTC−, DTC+
DTC−, DTC−
Pan-CK staining
M0 and M1
–
114
26 (22.8)
2 (1.7)
41 (35.9)
45 (39.4)
62.3
[166]
Pan-CK staining
M0 and M1
–
53
3 (5.6)
2 (3.7)
6 (11.3)
42 (79.2)
84.9
[69]
RT-PCR
M0 and M1
Detection of CK19
148
8 (5.4)
14 (9.5)
34 (22.9)
92 (62.2)
67.5
[167]
CK19 or mammaglobin
M0 and M1
Detection of mammaglobin
148
11 (7.4)
18 (12.2)
19 (12.8)
100 (67.6)
75.0
[167]
CK-staining
M0
–
341
8 (2.3)
26 (7.6)
40 (11.7)
267 (78.3)
80.6
[168]
Pan-CK staining
M0 and M1
–
39
12 (30.8)
3 (7.6)
12 (30.8)
12 (30.8)
61.5
[169]
RT-PCR, CK19
M0
Patients before neoadjuvant therapy
165
88 (53.3)
3 (1.8)
7 (4.2)
67 (40.6)
93.9
[170]
Patients after neoadjuvant therapy
84
32 (38.1)
12 (14.2)
11 (13.1)
29 (34.5)
72.6
[170]
CellSearch®, RT-PCR
M0
High-risk patients
27
16 (59.2)
4 (14.8)
3 (11.2)
4 (14.8)
74.1
[171]
Pan-CK staining, CellSearch®
M0
–
63
5 (7.9)
13 (20.6)
15 (23.8)
30 (47.6)
55.5
[172]
Average
72.8
[172]
Minimum
55.5
[172]
Maximum
93.9
[170]
Dissemination Sites: Lymph Nodes, Peripheral Blood, and Bone Marrow
Lymph Nodes (LN)
In the past, the detection of DTC is most important in pathological staging of lymph node (LN) specimens. In the last few years, the existence of DTC in bone marrow has also been shown to provide prognostic information. Promising detection strategies for DTC in peripheral blood (PB) are also being examined. Regarding LN in breast cancer, the risk of metastatic disease is classically estimated by factors such as tumor size, tumor grade, estrogen (ESR1) and progesterone (PGR) receptor status, ploidy, ERBB2 (HER2/neu), cytokines, MMPs, NF-KB overexpression, and the number of positive axillary lymph nodes (ALN). Several studies have shown that the presence of DTC in ALN is the most powerful prognostic factor, being associated with significantly poor disease-free (DFS) and overall survival [1]. During the past few years, the theory of sentinel lymph node (SLN) has emerged. SLN biopsy gears mapping of one or two LNs that primarily drain the tumor (the sentinel nodes) and therefore are most likely to harbor the metastatic disease. SLN examination is now widely performed in breast cancer, as it can provide prognostic value with minimal associated morbidity in contrast to complete ALN dissection.
The prescreening of SLN with highly sensitive detection methods for micrometastases thus represents a promising strategy. Considering that significant numbers of LN-negative patients develop metastatic disease, the dependability of current staging procedures to detect DTC in LN has been uncertain.
Peripheral Blood (PB)
Peripheral blood (PB) is historically one of the most potent diagnostic specimens. For example, circulating tumor markers have been evaluated in serum for years to give indicative values about metastatic or budding primary breast cancer. Serum markers may be good indications for tumor load, yet in most cases, they fail to provide information about minimal residual disease thus not up to mark. Technically speaking, PB appears as an ideal source for the monitoring of DTC. In fact, PB sampling is relatively trouble-free and can be done at frequent intervals (for instance, to permit an assessment of the patient’s recovery or potential to develop metastases). Several reports have demonstrated the presence of DTC in PB of patients with early-stage cancer without overt metastases [1, 24].
Bone Marrow (BM)
Contrary to PB sampling, blood marrow (BM) aspiration during surgery appears time-consuming and uncomfortable for the patient. However, among the distant organs, BM is a normal homing site for DTCs derived from breast cancer and other primary carcinomas, even in the absence of LN metastases or clinical signs of overt distant metastases [1]. Indeed, the screening rate of DTC in BM from nonmetastatic breast cancer patients has been demonstrated to be in the range from 0 % [44] to 100 % [45], and this corresponds to the variability of results obtained by the use of different techniques or marker genes. In a recent, large (more than 3,500 cases) study of stages I through III breast cancer patients, the incidence of DTC in BM detected by immunocytochemistry (ICC) ranged from 13 to 43 % [46]. The presence of DTC in BM may be supportive not only in predicting the development of bone metastases but also in predicting the development of metastases in other remote organs, such as the lung and liver. At present, however, it remains unsolved whether BM is a reservoir that allows for DTC to adapt and disseminate later into other organs or whether the presence of DTC in BM might reflect the general tendency of these cells to disseminate and survive in organs, rather than just in the BM. Until methods are developed to detect the presence of DTC in organs, such as the lung or liver, it will not be possible to distinguish between these two possibilities. The BM could serve as a reservoir in breast cancer and is supported by the presence of epithelial (cytokeratin-positive) cells in the PB of patients with overt remote metastases years after the removal of the primary tumor. This suggests that tumor cells could break from bone metastases to recirculate and disseminate to secondary tissues [1]. This “two-step” metastasis model could explain why the DTC in patients with overt metastases closely resemble each other genetically [47].
According to Ring et al. [48], in studies using antibody-based (cytometric) assays, cells with the characteristics of tumor cells have been shown in the PB of between 0 and 100 % of patients with operable (stages I through IIIa) breast cancer and in the PB of between 3 and 100 % of patients with metastatic disease. Several reports with nucleic acid-based techniques have shown cells with the characteristics of tumor cells in the PB of 0–88 % of patients with operable (stages I through IIIa) breast cancer and in 0–100 % of patients with metastatic disease. Along the same line, in a survey on a total of more than 3,500 stages I through III breast cancer patients, the incidence of DTCs in BM detected by ICC ranged from 13 to 43 % [46]. In fact, the detection rate of DTCs in BM from nonmetastatic breast cancer patients has been reported to be in the range from 0 % [44] to 100 % [45]. The variability of results obtained in DTC detection results from dramatic variations in methodology. Factors that may influence the results as heterogeneity of the studied populations may be:
1.
Stage. The number of positive patients and the absolute numbers of DTCs per patient rise as clinical stage rises [49].
2.
Interval of time separating surgery from the obtaining of DTCs. Surgery may increase the number of breast cancer DTCs (from 0 to 8,000 cells/ml) in the PB, which persist for varying length of times in different patients [50].
3.
Metastasis location. The separation of populations into those with early and metastatic breast cancer is probably simplistic. Moreover, metastasis sites could be missed when DTCs are obtained, leading to a misclassification of the patient in the “early breast cancer” category.
Other factors such as sample handling and preparation, delay between collection and analysis, conditions of sample storage, and contamination with normal epithelial cells may influence the results. The introduction of skin cells into a PB sample at the time of venipuncture could lead to false-positive results. Many researchers advocate that the first few milliliters of sampled PB are discarded to avoid such contamination. It has also been suggested recently that false-positivity of SLN could result from iatrogenic displacement and transport of benign epithelial cells in patients with breast carcinoma [51]. Clearly, such epithelial cells do not represent metastasis.
Detection of DTCs/CTCs in Bone Marrow
Current models of breast cancer metastasis hold up the possibility of early dissemination of cells from primary tumors and the direct release of DTCs into the blood and BM, bypassing, in some cases, the lymphatic system. DTCs are rare with only 10–20 cells among millions of BM cells. In order to increase the opportunity to screen DTCs in this organ, procedures had to be developed for their enrichment prior to detection and further characterization. For this, different density gradient centrifugation methods such as Ficoll-based assays or the OncoQuick approach, as well as positive or negative immunomagnetic enrichment procedures and simple filtration methods separating tumor cells by their size, have been recognized [9]. Currently, there are two different methods to detect BM aspirates for DTCs/CTCs—namely, cytologic/cytometric (antibody-based) and molecular approaches and nucleic acid-based approaches. The current technologies for CTC detections are summarized in Table 22.2, and a list of commonly used markers in assays to detect disseminated tumor cells by antibody- or nucleic acid-based techniques is summarized in Table 22.3.
Table 22.2
Current technologies for CTC detection
Assay system | Enrichment | Detection | Comments |
---|---|---|---|
EPCAM–based assays | |||
CellSearch® system | Immunomagnetic beads EpCAM-Ab-coupled ferrofluid | Immunocytochemistry Positive for CK8, 18, 19 Negative for CD45 Nucleus positive for DAPI | Semiautomated system with FDA approval for metastatic breast, colon, and prostate cancer. CTC can be enumerated and visualized [173] |
CTC-chip | Microposts: EpCAM-Ab-coupled microposts | Immunocytochemistry Positive for CK8, 18, 19 Negative for CD45 Nucleus positive for DAPI | |
CTC-chip Ephesia | Column of nanobeads EpCAM-Ab-coupled ferrofluids | Immunocytochemistry Positive for CKs Negative for CD45 Nucleus positive for DAPI | Lack of validation studies in clinical settings [176] |
MagSweeper | Immunomagnetic beads EpCAM-Ab-coupled ferrofluids | Microscope visualization Morphology | Isolation of CepC with a high degree of purity. Analysis of large blood volume [177] |
Laser scanning cytometry Maintrac® | RBC lysis | Immunocytochemistry Positive for EpCAM Negative for CD45 | High incidence of positive events up to 3 logs higher CTC counts than those obtained with other techniques warrants further investigations of assay specificity [178] |
Ikoniscope® imaging system | Ficoll-isopaque or filtration with track-etched membranes | Immunocytochemistry Positive for EpCAM, CK7/8 PSA (prostate only) FISH: chromosomes 7 and 8 Nucleus positive for DAPI | Two epithelial-specific Abs and FISH to detect chromosomal abnormalities in CTCs [179] |
Ariol® system | RBC lysis, then immunomagnetic beads CK-Ab- + EpCAM-Ab-coupled ferrofluids | Immunocytochemistry Positive for CK8, 18, 19 Negative for CD45 Nucleus positive for DAPI | Detection of EpCAM+ and EpCAM- CTCs [180] |
AdnaTest | Immunomagnetic beads MUC1-, EpCAM-Ab-coupled microbeads | Molecular biology RT-PCR Positive for at least one of the following markers: MUC1, HER2, EpCAM | AdnaTest also does not quantify the tumor cell load, false-positive results due to unspecific amplification, no further analysis possible [181] |
Functional assays | |||
EPISPOT assay | Rosette plus Ficoll Depletion of CD45+ cells | Secretion of proteins CK19, MUC1, Cath-D (breast); CK19 (colon); PSA (prostate); TG (thyroid) | Detection of viable epithelial secreting cells; unbiased enrichment independent of CTC/DTC phenotype [9] |
Vita-AssayTM or Collagen Adhesion Matrix (CAM) technology | Invasion capacity Ingestion of fluorescent CAM fragments (CAM+) | Immunocytochemistry Positive for EpCAM, ESA, pan-CK 4, 5, 6, 8, 10, 13, and 18 Negative for CD45 | Detection of CTCs with the invasive phenotype in blood [182] |
Other | |||
ISET | Cell size | Immunocytochemistry, positive for CK nucleus: Mayer’s hematoxylin | Sensitivity threshold of one carcinoma cell per milliliter of blood; HER2 amplification determined by real-time PCR on DNA extracted from CK immunostained cells (CTCs) collected by laser microdissection from selected ISET-positive filters; the possibility of false-positive diagnosis stresses the need for using ancillary methods to improve this approach [183] |
FAST (fiber-optic array scanning technology) | No pre-enrichment | Immunofluorescence Positive for CK Nucleus positive for DAPI Morphology | Rare cells detected by laser scanning to almost 1,000 times faster than digital microscopy [184] |
DEP-FFF (dielectrophoretic field-flow-fractionation) | Phenotype–membrane capacitance | Immunocytochemistry Wright stain | No need for labeling or modification of CTCs; PBMC/CTC ratio is enriched more than 2,000-fold; CTCs isolated by DEP are viable and suitable for a wide spectrum of analyses [185] |
Versatile label-free biochip | Cell size deformability | Immunofluorescence Positive for CK Negative for CD45 Nucleus positive for DAPI Morphology | Label-free selection and CTCs are viable after blood processing [186] |
Table 22.3
Markers used as assays to detect disseminated tumor cells by antibody or nucleic acid-based techniques
Marker (gene) name | Gene locus | Standard name | Other frequently used names | Reference(s) related to DTC detection |
---|---|---|---|---|
ANKRD30A | 10p11.21 | Ankyrin repeat domain 30A | Breast cancer antigen NY-BR-1; B726P | |
B305D | 21q11.1–q11.2 | Antigen B305D | B305D; isoform A (B305D-A); B305D; isoform C (B305D-C) | |
CD44 | 11p13-pter | Antigen CD44 | Hermes antigen, PGP1 | [60] |
CDH1 | 16q22.1 | Cadherin-1 (epithelial) | E-cadherin, uvomorulin | [189] |
KRT19 | 17q21–q22 | Keratin 19 | Cytokeratin 19 (CK19) | |
KRT7 | 12q12–q14 | Keratin 7 | Cytokeratin 7 (CK7), sarcolectin (SCL) | [48] |
GABRP – | 5q32-q33 | γ-Aminobutyric acid type A receptor pi subunit | GABA receptor A, pi polypeptide (GABARAP); GABAA receptor, pi polypeptide (GABA A(pi)) |
Antibody-Based Techniques
Approaches by fluorescence microscopy (FM), ICC, and flow cytometry (FC) analysis aim to isolate and enumerate individual tumor cells. ICC is still a gold standard for DTC detection, and most of the available clinical data have been gathered by ICC screening, especially in BM [23]. An advantage of this approach is that it may permit further characterization of the cells at a molecular level, in terms of expression of key biological markers, such as ERBB2 (ERBB2 gene amplification estimated by FISH analysis) and morphological cell investigation. However, identification of intracellular targets, such as cytokeratins, by antibodies needs cell permeabilization. As a consequence, cell viability is lost, making the important discrimination of dead and viable DTC impossible. Since only viable cells might lead to metastasis, this valuable information cannot be evaluated [23].
Like IHC, FM and ICC are labor intensive and time-consuming, making these techniques too expensive for routine implementation. When compared with conventional, essentially qualitative FM and ICC, FC offers the advantage of a fully automated technique permitting quantitative measurements with high sensitivity, good resolution, speed, reproducibility, and statistical reliability. For breast tumors, the most used targets for antibody-based techniques are the cytokeratins. ERBB2, MUC1, and TACSTD1, the latter two being known under a variety of names, have also been used as antibody targets to isolate and/or identify DTC.
Two–color ELISPOT, an immunological assay based on enzyme-linked immunosorbent assay, has been recently used to detect DTC-secreting cathepsin D (CTSD) and mucin-1 (MUC1). However, antibody-based techniques have limitations. Many of the antibodies directed at epithelial and breast cancer cells are known to also stain hematopoietic cells, including cytokeratins (KRT19), TACSTD1, and MUC1. Nonspecific staining of plasma cells can also occur due to alkaline phosphatase reaction against the k and l light chains on the cell surface [52]. According to the antibody used, a false-positive detection rate of 1–3 % can be estimated [23]. Since tumor- and epithelial-specific cell marker antigens are expressed differentially in DTCs, the use of a panel of monoclonal antibodies may help to enrich DTCs and facilitate their finding [53].
Nucleic Acid-Based Techniques
PCR, either qualitative or quantitative, has been used to identify and characterize DTCs through the detection of genetic (allele-specific expression, microsatellite instability, loss of heterozygosity) and epigenetic alterations (methylation status) that are exclusively linked with cancer cells [54]. This includes the search for tumor-associated point mutations in oncogenes or tumor suppressors. This latter PCR approach, however, is complex by the substantial degree of genetic variability between tumors. For instance, TP53, the gene coding for p53, is mutated in about 25 % of breast tumors; however, more than 1,400 different mutations of this gene have been observed [55]. Of note, PCR has been used to screen free DNA within plasma. For instance, the analysis of DNA methylation status of specific genes (ESR1, APC, HSD17B4, HIC1, and RASSF1A) in serum of breast cancer patients has been shown to be of prognostic value [56]. The PCR-based measurement of RASSF1A methylation has been used for examining efficacy of adjuvant tamoxifen therapy [57]. However, this use of PCR is imperfect by poor specificity. This is due in part to the high stability of DNA in plasma when compared with mRNA [58]. As a result, it is unclear whether the free DNA that is amplified from plasma is from DTCs present in plasma or if the DNA is being shed from primary tumors, metastatic tumors, or from normal tissue [48]. To identify DNA gains and losses in single DTC, the technique of comparative genomic hybridization (CGH) is increasingly used [59].
Reverse transcription (RT)-PCR has been used to identify DTC through their expression of epithelial or breast cancer-associated mRNA transcripts. RT-PCR is generally more sensitive than antibody-based techniques but has also been hampered by false-positive results in samples from normal volunteers and from patients with hematological malignancies [48]. These false-positives stem from multiple sources, including issues with laboratory technique, primer selection, illegitimate expression of the target genes in normal cells, the existence of pseudogenes, or contamination (KRT19/CK19). When using assays based on RT-PCR for detection of DTCs, the balance between sensitivity and specificity must be considered. Generally, specificity decreases with the increase in sensitivity, and vice versa. One way to resolve this problem is to examine multiple tumor markers in samples. As mentioned below, multiplex RT-PCR assays have revealed a higher efficacy (in both sensitivity and specificity) in comparison with the assessment of single markers. To recover the reliability, especially the specificity of RT-PCR assays, quantitative RT-PCR (qRT-PCR) may be used. In addition, qualitative marker information, qRT-PCR uses cutoff values of marker transcript numbers, below which transcripts can be considered as tumor cell-derived. Moreover, when compared with conventional RT-PCR, qRT-PCR relies not only on primers but also on internal probes that specifically hybridize to the amplified sequences. In addition, due to the continuous measurement of the amplified signal, false-positive results, which could produce an abnormally shaped, nonlinear amplification curve, could be easily identified and removed [23]. Variations of the RT-PCR technique, such as nested RT-PCR and competitive nested RT-PCR, have also been used [60].
Fluorescence in situ hybridization (FISH) allows the detection of gene amplifications, for instance, ERBB2 amplification in breast cancer. FISH has been used to analyze genetic aberrations in DTC in BM. Considering the importance of ERBB2 as a novel target for successful antibody-based therapy, the use of FISH to identify ERBB2 amplification in DTC appears promising [61]. Among the cytologic methods that allow isolation and enumeration of individual cells, immunocytochemistry is the most widely used approach. Because of the absence of tumor-specific target antigens—most commonly antibodies against various epithelium-specific antigens such as cytoskeleton-associated cytokeratins—surface adhesion molecules or growth factor receptors are used for the screening of carcinoma cells [62]. The main advantage of cytologic methods is the opportunity to combine immunostaining with the morphology of the cells so that cell size and shape as well as the nucleusplasma relation might be predictable and illicit expression of the protein of interest in BM cells can be excluded.
The detection of DTCs in BM is not yet a routine part of the tumor staging in the clinical practice, but rising data anticipate a future role of DTC screening for risk estimation and therapeutic monitoring of breast cancer patients [63]. However, the detection rates of DTCs in BM from nonmetastatic breast cancer patients vary significantly [45]. This might reflect the different sensitivity, but also specificity, of the numerous detection methods and marker genes/proteins used thus far. The newly defined consensus concept for the detection of DTCs in BM, signifying enrichment of mononuclear cells from BM by Ficoll density gradient centrifugation and immunocytochemical detection of cytokeratin expression as standard procedure, should help overcome these troubles and provide the basis for future multicentric clinical trials. The researchers recommend the pan-anti-cytokeratin antibodies A45-B/B3 or AE1/AE3 against a wide spectrum of cytokeratins as standard application, thereby ensuring detection of DTCs also in cells that have downregulated the expression of individual cytokeratins in the course of epithelial–mesenchymal transition [42]. Microscopic screening of large amounts of immunostained cytologic preparations is accomplished by automatic microscopes using sophisticated imaging approaches. Criteria to examine morphology and staining results have also been defined to avoid false-positive and false-negative results [42].
Although there are existing recommendations for standard operation procedures, there are still restrictions to the standardization of immunocytochemical methods with respect to reproducibility of the staining procedure itself as well as microscopic interpretations. Therefore, both intra- and interlaboratory evaluation of the methods is required to ensure reliability of the results [64].
Besides immunocytochemical methods, very sensitive nucleic acid-based techniques now allow the detection of DTCs at the single-cell level. The main advantage of these methods is the nearly unlimited availability of primers for almost every gene of interest. Although numerous genetic alterations have been described in breast cancer cells, heterogeneity is enormous, so that at present no universally applicable DNA marker exists for the primary screening of a wide range of DTCs [9]. Further efforts have been made to detect free circulating DNA or epigenetic alterations of circulating DNA such as methylation in BM and blood plasma, but the results are still preliminary [65]. Therefore, the measurement of epithelium-specific or more organ-specific mRNA species such as cytokeratin 19 or mammaglobin mRNA by RT-PCR has been proven to be a promising approach to detect DTCs in BM samples [66]. Because of the lack of tumor-specific markers, the main disadvantage of using surrogate tissue-specific markers is false-positive results due to illegitimate low-level transcription of epithelial or breast tissue-specific genes in normal cells [48]. Furthermore, heterogeneity in the expression of particular genes is not recognizable and the expression level of a gene of interest per cell cannot be estimated. At present, analyses are mainly performed by quantitative real-time RT-PCR, ensuring the discrimination between different levels of expression. Moreover, multimarker real-time RT-PCRs have the potential to improve the method even in the case of downregulation of the expression of a single gene [45]. However, storage and sample preparation have to be performed under conditions avoiding RNA degradation, one of the major problems of RT-PCR approaches [66].
The application of multimarker assays might also compensate for low mRNA amounts due to the low number of tumor cells. There are numerous excellent reviews listing the marker genes currently used in RT-PCR approaches to detect DTCs in BM or CTCs in blood from breast cancer patients [48]. The methods explained above are not able to discriminate between viable and apoptotic DTCs. A new technique, designated EPISPOT (epithelial immunospot), offers the advantage of detecting viable tumor cells by their ability to secrete individual proteins. In a newly published study, it was demonstrated that BM samples from metastatic and nonmetastatic breast cancer patients contain viable tumor cells which secret Muc-1 and/or cytokeratin 19 in about 90 and 50 % of cases, respectively, whereas in controls from healthy women, cells secreting these proteins could not be detected [9].
Clinical Relevance of DTCs in Bone Marrow (BM)
A large number of studies have documented DTCs in BM from patients with most types of epithelial cancers [1]. Within the last 15 years, several studies have confirmed that detection of DTCs in BM of breast cancer patients is accompanied by a substantially worse prognosis [63]. In a pooled analysis evaluating the results from 9 different European centers, including a total of 4,703 patients, Braun et al. have reported that approximately 30 % of women with primary breast cancer have DTCs in BM, and in a multivariate analysis, the 10-year follow-up of these patients revealed a significantly decreased overall survival, when compared to patients without DTCs [67]. The presence of DTCs in BM was significantly associated with higher tumor stage, worse differentiation, lymph node metastasis, and negativity in hormone receptor expression. Prognostic relevance was shown for all subgroups, even among those patients with small tumors and without lymph node metastasis. While using different antibodies and detection methods, almost all investigators participating in this pooled analysis used anti-cytokeratin antibodies to screen for DTCs in the BM [67].
Bone Marrow of DTCs Replaceable by Blood CTCs?
Aspiration of bone marrow (BM) is invasive, time-consuming, and in many cases painful or at least uncomfortable for patients, precluding repeated samplings necessary for therapy-monitoring studies. Moreover, BM aspiration is more difficult to standardize with regard to the required volume and quality. Consequently, recent efforts have concentrated on the detection of CTCs in peripheral blood (PB) of cancer patients [48], but the clinical usage of CTCs has not yet been implemented for routine clinical practice. Furthermore, there are only a limited number of studies comparing BM and PB examinations performed at the same time points, and the clinical significance of CTCs in PB is less clear than that for DTCs in BM. In all studies published thus far, there was a higher frequency of BM-positive than blood-positive samples from the same patients [68], probably due to the fact that BM might provide conditions for homing and survival of DTCs, thus contributing to their accumulation in this compartment. Although both Pierga et al. [68] and Muller et al. reported about a significant number of patients with concordant results concerning BM and blood analysis [69], in the Pierga study, only the presence of DTCs in BM and not that of CTCs in blood had prognostic relevance for disease-free survival in nonmetastatic breast cancer patients [68]. Interestingly, the presence of both DTCs in BM and CTCs in blood in a subgroup of patients resulted in an especially poor prognosis [70]. While all studies referred above applied immunocytochemical methods, real-time RT-PCR detection of DTCs in BM also had superior prognostic significance in comparison with CTCs in patients with breast cancer. A study analyzed cytokeratin 19 and mammaglobin mRNA levels by quantitative RT-PCR [71]. Currently, the results obtained by comparative studies do not hold a replacement of BM by blood analysis, but CTC detection might have supplementary value.
There are an increasing number of studies demonstrating clinical relevance of CTCs in blood detected by real-time RT-PCR, identifying either only cytokeratin 19 mRNA or multiple markers [62]. Recently, analyzing cytokeratin 19 mRNA by real-time RT-PCR [72], they detected CTCs in 22 % of blood samples from 167 node-negative breast cancer patients as significantly associated with overall and disease-free survival. A correlation of the presence of CTCs in blood to the lymph node status was found in 2007 [73], when CTCs were detected with the help of a multimarker real-time RT-PCR in 39 of 90 (43 %) stage I through III breast cancer patients, but not in normal healthy volunteers.
Significant progress in this field arose from the development of an automated enrichment and immunocytochemical detection system for CTCs (CellSearch™) [74]. This system consists of an automated instrument for the enrichment of epithelial cells by ferrofluids coated with anti-EpCAM antibodies followed by immunostaining of captured cells with fluorescently labeled anti-cytokeratin and anti-CD45 antibodies (AutoPrep), and a semiautomated microscope for scanning and reading results (CellSpotter Analyzer). Using this system, Cristofanilli et al. [74] demonstrated in a prospective study that CTC detection provided important prognostic information for patients with metastatic breast cancer. Additionally, Hayes et al. demonstrated that CTCs at each follow-up time point during therapy of these metastatic breast cancer patients predict progression-free and overall survival. The CellSearch system has been cleared by the FDA for regular clinical use in metastatic breast cancer patients. Validation data from three independent laboratories and high interinstrument accordance confirmed the reliability of this system for CTC measurements in PB from metastatic breast cancer patients.
Also, it was shown that samples can be shipped at room temperature and CTC counts are stable for at least 72 h, which facilitates testing at central laboratories or remote sites requiring transportation [75]. There are also several reports about the detection of CTCs in patients with primary breast cancer, however, mostly with lower frequencies and varying results concerning both the number of positive patients and the number of CTCs in individual patients [76].
Molecular Characterization of DTCs in Bone Marrow and CTCs in Blood
The characterization of DTCs/CTCs is aimed to (1) provide proof for their malignant origin and (2) identify further diagnostically and therapeutically related features of these cells, which might permit a more targeted and individualized antimetastatic therapy. This characterization is hindered by the fact that DTCs/CTCs can exhibit features distinct from the primary tumors, but on the other hand, this could help to identify cancer patients for additional targeted therapies. By multiple fluorescence in situ hybridization analysis, it was shown that the vast majority of CTCs in blood from breast cancer patients are aneusomic and derived from the primary tumor [42]. By single-cell comparative genomic hybridization, further study indicated that DTCs might be gnomically unstable and heterogeneous [77]. Moreover, research also suggests that DTCs from BM of breast cancer patients disseminate in a less progressed genomic state and might acquire genomic alterations typical for metastatic cells later [78].
In order to escape from the dormant state into the dynamic phase of metastasis arrangement, dormancy has to be disturbed by both genetic and epigenetic changes in the DTCs/CTCs as well as in the surrounding microenvironment or premetastatic niche [79]. Transcriptional analyses of EpCAM-enriched BM and blood cells resulted in gene expression profiles that may be used to differentiate normal donors from cancer patients [80]. Further studies have to reveal whether individual genes, the expression of which is changed in these cell populations, might become markers to recognize recurrence in breast cancer patients early [80].
Interestingly, TWIST1, a transcription factor that in the past has been recognized to play an important role in metastasis by accelerating epithelial–mesenchymal transition [81], was part of the gene expression signature identified in EpCAM-enriched cells from BM of breast cancer patients after chemotherapy [80]. TWIST1 expression, which was not observed in EpCAM-enriched cells of BM from healthy volunteers, linked with the occurrence of remote metastasis and local progression, even in pretreatment BM samples [80].
DTCs/CTCs seem to be heterogeneous with regard to the expression of growth factor receptors, adhesion molecules, proteases, and their inducers and receptors, major histocompatibility complex antigens, or signaling kinases [47]. Of particular attention is the epidermal growth factor receptor HER2, the expression of which in primary tumors forms the basis of Herceptin treatment decisions for breast cancer patients.
As shown by Braun et al., HER2 overexpression on DTCs in BM was predictive for a poor clinical outcome of stage I through III breast cancer patients [82]. While a study of 27 breast cancer patients showed that the HER2 status remained relatively stable between primary tumors and BM micrometastases in most cases [83], there is also increasing proof for discrepancies between the HER2 status in primary tumors and DTCs in BM [84]. They noticed HER2-positive DTCs in 12 of 20 BM samples from patients with HER2-negative primary tumors. Although HER2 expression was heterogeneous in DTCs from individual patients, HER2-positive DTCs might recognize additional patients who can benefit from Herceptin therapy. The HER2 status of CTCs from PB might also be different from that of the corresponding primary tumors as reported [85]. These authors presented a significant number of patients whose primary tumors were HER2 negative, whereas CTCs were HER2 positive before surgery. Moreover, in this study the recognition of HER2-positive CTCs correlated significantly with disease-free and overall survival [85]. It remains to be explored whether high levels of HER2-positive CTCs reflect the activity of the tumor and have predictive value for an improved response of the patients to Herceptin treatment [85]. Although Meng et al. reported a high agreement (97 %) of the HER2 status between primary tumors and CTCs in 31 cases, during tumor progression, HER2-positive CTCs could be detected in 9 of 24 breast cancer patients in spite of HER2-negative primary tumors. These CTCs might have acquired HER2 gene amplifications. Four of these patients received Herceptin therapy and three of them responded to this therapy [61].
In the study shown by Apostolaki et al., adjuvant chemotherapy eliminated HER2 mRNA-positive CTCs in 16 of 45 patients. The detection of HER2 mRNA-positive CTCs after chemotherapy was linked with a reduced disease-free survival. Moreover, in 8 of 161 patients with HER2-negative primary tumors, HER2 mRNA-positive CTCs could be noticed [86]. Therefore, the detection of HER2 mRNA-positive CTCs after adjuvant chemotherapy in the PB of stage I and II breast cancer patients might provide information about the usefulness of chemotherapy and the prognosis of the patients and identify patients in need of additional Herceptin therapy [86].
During the past few years, the number of single markers that have been assessed for DTC detection, mainly by nucleic acid-based techniques, has noticeably increased. For a detailed description of these studies, the reader is encouraged to consult the current reports published by Gilbey et al. [60] and Ring et al. [48]. In this chapter, the same name will be used for the gene and the corresponding protein. For instance, regardless of the fact that the terms NY-BR-1 and B726P are bumped into in the literature, the name of the corresponding gene, ANKRD30A, will also preferentially be used to cite the protein. SCGB2A2 will be used instead of mammaglobin, ESR1 rather than estrogen receptor-a (ERa), etc.
An ideal marker should be universally, but exclusively, expressed on all breast cancer cells. It should be easily noticeable, with little variance, and bear clinical relevance. Since no single, precise marker that meets these criteria has been recognized, attempts are now made to develop assays with multiple tumor markers, of which some are preferably highly specific to breast tissue or breast tumors. The aim is to avoid both false-positive (detection of non-tumor cells, due to the fact that the majority of potential markers have some baseline expression in normal tissues) and false-negative (non-detection of tumor cells, due to the use of high-threshold levels for positivity) cases.
Multimarker assays have been used by various researchers [48, 60, 87] and have shown a higher efficacy (sensitivity and specificity) in comparison with the assessment of single markers. Markers with low breast (cancer) specificity cytokeratins (KRTs) regarding epithelial tumors, the cytoskeleton components KRTs have become the markers of choice for DTC recognition. They belong to a large multigene family of more than 30 identified members. They are expressed at various levels and compositions in all epithelial tumors, but hardly ever in other tissues. For antibody-based studies, most use a combination of several monoclonal antibodies that distinguish various cytokeratin antigens or a broad-spectrum anti-cytokeratin monoclonal antibody that recognizes a single epitope that is frequent to most cytokeratins [1, 48]. For nucleic acid-based studies, cytokeratin 19 (KRT19) and, to a lesser extent, cytokeratin 20 (KRT20) have been commonly used as markers. KRT19 presents an illustration of the possible sources of false-positivity in DTC detection.
Due to its high sensitivity, KRT19 is the widely used marker for finding DTCs in breast cancer patients [48, 60]. Depending on the assays, KRT19 has been discovered to be both a specific and a nonspecific marker. In fact, KRT19 is an outstanding candidate to demonstrate the potential sources of false-positivity in RT-PCR studies: illegitimate transcription, hematological disorders, the presence of pseudogenes, and sample contamination. Illegitimate transcription explains the expression in normal tissues of small amounts of mRNA by genes that have no actual physiological role in these cells. It can be estimated that every promoter could be activated by ubiquitous transcription factors, which leads to an estimated expression level of one tumor marker gene transcript in 500–1,000 non-tumor cells [23]. For hematological disorders, KRT19 expression can be induced in PB by cytokines and growth factors, which circulate at higher concentrations in inflammatory conditions and neutropenia [48]. As a consequence, false-positive results are more expected under these circumstances. The presence of pseudogenes, two KRT19 pseudogenes, KRT19a and KRT19b [88], have been identified, which have significant sequence homology to KRT19 mRNA. Consequently, attempts to identify the expression of the authentic KRT19 may result in the detection of either or both of these pseudogenes. To avoid pseudogene amplification, it is suggested to carefully design the primers used for RT-PCR analysis. Regarding contamination, it has been suggested that PB sampling for subsequent analysis could introduce contaminating epithelial cells expressing the KRT19 mRNA into the blood sample. Possible contamination could be reduced or prevented by discarding the first sample of blood taken.
In conclusion, KRT19 emerges to be a very sensitive tumor marker, whose use, however, is often held back by low specificity. It is useful in detecting disseminated epithelial cells but is not a true breast cancer marker.
KRT20
KRT20 is found in breast cancer cells [89]. However, its expression is less linked to breast tissue and more related to gastric and intestinal epithelium, urothelium, and Merkel cells [23]. Additionally, KRT20 expression has been established in granulocytes [90]. Due to its lower specificity when compared with KRT19, the use of KRT20 is not suggested in breast cancer patients. KRT8 and KRT18 have been hardly ever used for DTC detection. In fact, the expression patterns of these epithelial cytokines are very similar to that of KRT19 and they are not expected to provide more specificity than KRT19. Of note, KRT8, KRT18, and KRT19 are expressed in the breast epithelium but at higher levels in the luminal than in the basal component. In view of recent observations that breast tumors may be classified into subtypes, or classes, including luminal epithelial-like and basal epithelial-like, one can believe that these cytokeratins will be less easily distinguished in DTCs originating from basal-like tumors.
CEACAM5
Commonly known as CEA, it functions in several biological roles, including cell–cell adhesion. It is one of the most commonly expressed markers in breast, as well as in various other, cancer cells [48, 60]. Therefore, it suffers from low specificity, as also seen with KRT19, and can likewise be induced in peripheral blood (PB) by cytokines and growth factors [48].
TACSTD1
This epithelial cell–cell adhesion protein is known under a range of names, of which GA733-2 and EpCAM are the most commonly used. Ubiquitously expressed on the surface of epithelial cells, it has been normally used as a target for positive IMS to enrich DTC for RT-PCR analysis [23]. Monoclonal antibodies against this antigen have been widely developed for diagnostic, but also therapeutic, approaches. Although highly sensitive for epithelial malignancies, including breast cancer, its use is, however, hindered by the fact that it is expressed in low amounts in PB cells [91].
MUC1
Mucin-1 is an extensive, polymorphic, and heavily glycosylated mucin. The role of mucins is mainly one of the hydrating and lubricating epithelial linings, but these proteins have also been concerned in modulating both growth factor signaling and cell adhesion. Further, it has been suggested that MUC1 expression at the surface of tumor cells could decrease cell adhesion and favor dissemination [92]. Conversely, MUC1 could play a role in the initial attachment of breast tumor cells to tissue at remote sites, facilitating establishment of metastatic sites [93]. Extensively expressed in normal epithelial tissues, MUC1 is remarkably present on the apical surfaces of breast, bronchial, pancreatic, uterine, salivary, intestinal, and other glandular tissue cells. Like TACSTD1, MUC1 has been commonly used as a target for positive IMS to enrich DTC for RT-PCR analysis [23]. Many studies have reported the expression of MUC1 in a significant proportion of healthy blood donors. Indeed, MUC1 expression has been considerably found in PB cells [23]. Although it has low specificity, the assessment of MUC1 expression in DTC is supported by the increasing interest for MUC1-based immunotherapy [94]. Although MUC1 is expressed in a majority of breast tumors, its overexpression has been associated with a lower grade and a higher ER-positive phenotype [95].
EGFR
A series of RT-PCR-based mono- or multimarker studies have assessed the relevance of this growth factor receptor for DTC detection [96, 97]. EGFR emerges as more specific but less sensitive than KRT19. Unluckily, it has also been found infrequently in the PB of healthy donors [23]. Furthermore, Weigelt et al. [97] have shown that the median expression of EGFR was higher in normal ALN than in DTC-positive ALN! Notably, EGFRvIII, a cancer-specific EGFR variant, has been now used to detect DTC in breast cancer patients. The mutant was seen in the peripheral blood in 30 % of 33 low-risk, early-stage patients, 56 % of 18 patients chosen for neoadjuvant chemotherapy, 63.6 % of 11 patients with disseminated disease, and, remarkably, 0 of 40 control women [98].
ERBB2
Involved in signal transduction, ERBB2 participates in breast tumor biology. Yet, it is not breast specific [99], and weak ERBB2 expression has been found in the PB of healthy women in several studies [23]. However, it is overexpressed in 20–35 % of breast cancer patients, mostly as a result of gene amplification, and this forecasts for reduced survival. Furthermore, in patients with breast cancer, ERBB2 overexpression by DTC in the BM predicts poor clinical outcome [82]. This, as well as the increasing use of ERBB2 as target for immunotherapy (trastuzumab) [94], supports its assessment in DTC, at both the mRNA (RT-PCR) and the DNA (FISH) levels.
Markers with High Breast (Cancer) Specificity
Using molecular biology methods or combinations of techniques, various groups have recognized markers specifically expressed in breast and/or breast cancer tissue or cells, when compared with normal PB, BM, or other human tissues. For instance, genes profusely expressed in breast cancer tissue but absent in normal PB and BM have been identified by serial investigation of gene expression (SAGE).
By order of decreasing SAGE tag frequency, these genes are SBEM, LACRT, TFF3, COL1A1, MGP, KRT8, MUC1, KRT7, CLECSF1, IL6ST, APOC1, SCGB2A2, TFF1, TM4SF1, C6, and KRT19 [100]. A series of genes coding for secreted proteins overexpressed in breast cancer tissue when compared with corresponding normal tissue and/or other (colon, gastric, kidney, liver, lung, ovary, pancreas, prostate) normal tissues were recognized by a combination of annotation/protein sequence analysis, transcript profiling, immunohistochemistry, and immunoassay: HAPLN1, GFRA, SCGB1D2, CXCL10, CXCL11, COL11A1, E2F3, TRMT1, CHST2, SERHL2, ZNF324, SCGB2A2, COX6C, and SCGB2A1 [101]. Gene expression profiling was used to construct a site of origin classifier in order to decide the origin of cancer of unknown primary. From an analysis of 229 primary and metastatic tumors representing 14 tumor types (breast – 34 samples, colorectal, gastric, melanoma, mesothelioma, ovarian, pancreas, prostate, renal, testicular, squamous cell carcinoma, uterine, and lung), a “finest” list of 79 site-specific markers was defined. Genes linked to breast specificity were ACADSB, CCNG2, ESR1, EFHD1, GATA3, SLC39A6, MYB, SCYL3, PIK3R3, PIP, PRLR, RABEP1, TRPS1, and VAV3. Two of them, GATA3 and PIP, were recognized as appearing to be strongly and relatively consistently expressed across the range of breast tumors.
Smirnov et al. [102] achieved PB containing R100 DTC from one metastatic colorectal, one metastatic prostate, and one metastatic breast cancer patient. In a primary step, global gene expression study was performed on these samples, and a list of cancer-specific DTC genes was achieved. Among genes distinguishing between tumor (colorectal, prostate, and breast) and control patients were KRT18, KRT19, TACSTD1, TACSTD2, AGR2, TFF1, and TFF3, all genes known to be linked to the epithelial cell phenotype. Fifty-three genes distinguishing between breast tumor and controls were recognized, including ESR1 and ERBB2.
In a second step, PB samples immunomagnetically enriched for DTC from 74 metastatic patients (30 colorectal, 31 prostate, 13 metastatic breast cancer patients, and 50 normal donors) were used to confirm the DTC-specific expression of selected genes by real-time RT-PCR. The genes most restricted to breast cancer patients, when compared with normal donors and colorectal cancer and prostate cancer patients, were SCGB2A1, SCGB2A2, and PIP. Two additional genes, S100A14 and S100A16, were restricted to breast and colon cancers. Of note, two genes, KRT19 and AGR2, were expressed in the majority of metastatic samples (colorectal and prostate and breast) and not in the control individuals. This validates the interest of KRT19 as an epithelial tumor cell marker.
Yet AGR2 expression has been less frequently examined. Smirnov et al. [102] isolated RNA from a highly metastatic SCGB2A2-overexpressing ALN (only one sample). It was diluted into a pool of normal LN RNA at various ratios. Gene expression (microarray) analysis was performed, and candidate breast cancer-associated genes were then selected based on three criteria: (a) absence of expression in a pool of four normal LN, (b) a high fluorescence signal on microarray, and (c) a fluorescence signal also present in the 1:50 dilution. The 34 genes recognized by criteria (a), (b), and (c) were specified by relative intensity of the signal in the metastatic ALN. The 14 genes were SCGB2A2, TFF1, TFF3, KRT19, SCGB1D2, S100P, FOS, SERPINA3, ESR1, TACSTD2, JUN, PGDS, KRT8, and AFP. Notably, other genes used for molecular finding of micrometastatic disease, such as PIP, SPDEF, TACSTD1, CEACAM5, and SCGB2A1, were not present among the top 15, even though their signal was observed in metastatic ALN. Real-time RT-PCR analysis of pathology-negative ALN (nZ72) demonstrated that of PIP, SCGB2A2, SPDEF, TACSTD1, and TFF1, SCGB2A2 and TFF1 had the highest evident sensitivity for the detection of micrometastatic breast cancer [103].
In a microarray approach, Backus et al. investigated RNA from samples covering normal, benign, and cancerous tissues from breast, colon, lung, ovarian, prostate, and peripheral blood leukocytes from healthy donors. By a combination of this microarray testing and database/literature searching, a series of candidate breast tissue-specific markers and candidate breast cancer status markers were recognized [104]. These potential markers were then submitted to an additional multiuse selection process: some markers were excluded for one of the following reasons: (1) their expression level in white blood cells was too high, (2) their expression in breast cancer was too low, and (3) their expression in lung, colon, and ovarian cancers was too high. The authors finally achieved 14 markers, of which 7, ANKRD30A, GABRP, KRT19, OR4K11P, PIP, SCGB2A2, and SPDEF, were further chosen (the others were CEACAM6, ERBB2, MUC1, S100A7, S100A14, SBEM, and TNNT1). The utility of these markers for identifying clinically utilizable metastases in LN was assessed through RT-PCR analysis of SLN from 254 breast cancer patients. The investigators recognized an optimal two-gene expression (KRT19 and SCGB2A2) marker set for the detection of the actionable metastasis in breast SLN [104].
A series of markers with high breast (cancer) specificity reported so far are now in details.
SCGB2A2
No breast cancer marker has been shown to be never expressed in healthy volunteers, but some markers are hardly ever found in controls. SCGB2A2 [105], widely known as mammaglobin, is one of these markers. It is a member of the secretoglobin superfamily [106], a group of small, secretory, rarely glycosylated, dimeric proteins generally expressed in mucosal tissues that could be involved in signaling, immune response, chemotaxis [107], and, probably, as a carrier for steroid hormones in humans. SCGB2A2 has become a quasi standard in breast DTC detection by RT-PCR-based methods, being the most extensively studied marker after KRT19. It has been used to identify DTC in LN, PB, BM, and even in malignant effusions. SCGB2A2 expression has been noticed, rarely and in low levels, in various normal tissues. This could restrict its prospective use as an immunotherapeutic target [108], due to concerns about autoimmune toxicity.
Zafrakas et al. have found an abundant SCGB2A2 expression in malignant and normal tissues of the breast and in the female genital tract, namely, the cervix, uterus, and ovary, while lower expression levels were hardly ever found in other tumors and normal tissues [109]. These remarks might extend the diagnostic potential of SCGB2A2 to the detection of DTC from gynecologic malignancies. While SCGB2A2 is significantly more breast cancer specific than KRT19, it is less “universal” among these tumors. Indeed, SCGB2A2 expression level is highly changeable in breast tumors, with some of them showing no expression at all. SCGB2A2 expression, estimated at mRNA or protein level, has been reported in 61–93 % of primary and/or metastatic breast cancer biopsies [110–112]. By examining SCGB2A2 gene expression levels in 11 BCC lines, BT-474, Evsa-T, Hs578T, IBEP-1, IBEP-2, IBEP-3 [113], KPL-1, MCF-7, MDA-MB-231, MDA-MB-453, and T-47D, by microarray and RT-PCR, researchers have shown elevated SCGB2A2 mRNA level only in Evsa-T BCC, while mild expression was seen in BT-474 BCC [114]. Notably, most of these BCC lines are of metastatic origin [113].
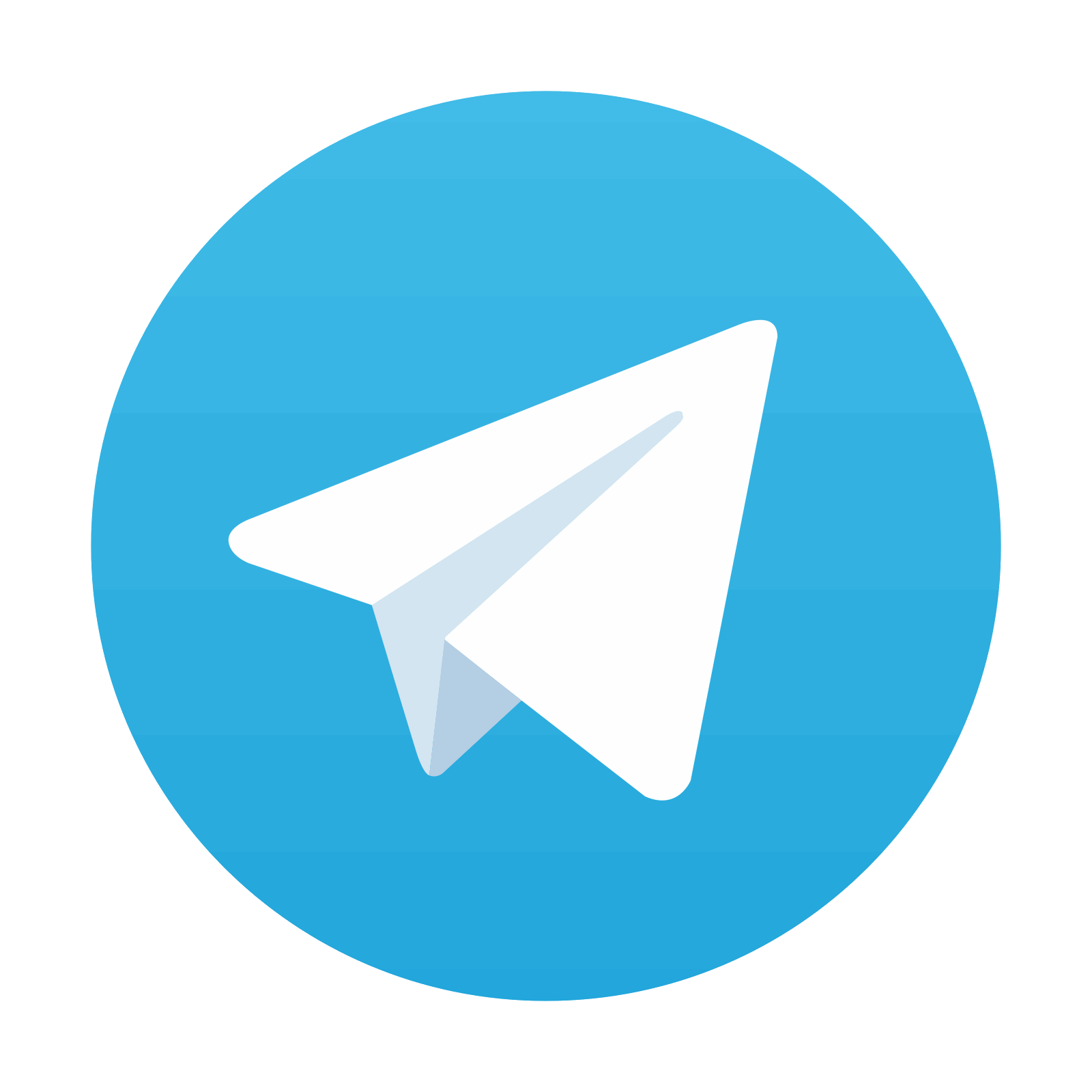
Stay updated, free articles. Join our Telegram channel

Full access? Get Clinical Tree
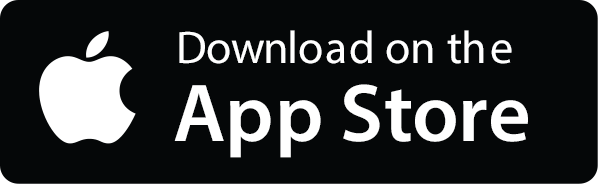
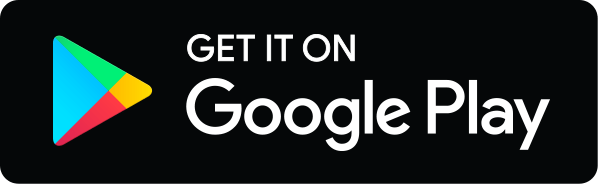