!DOCTYPE html PUBLIC “-//W3C//DTD XHTML 1.1//EN” “http://www.w3.org/TR/xhtml11/DTD/xhtml11.dtd”>
INTRODUCTION
Gynecologic cancer research has mirrored all cancer research programs in that it has focused largely on molecular defects in oncogenes, tumor-suppressor genes, and DNA repair mechanisms. Several research groups have also channeled their resources into various carcinogenic phenomena, such as apoptotic pathway defects, growth signaling, angiogenesis, tissue invasion, or metastasis. These efforts have led to a broad understanding of the chromosomal and molecular abnormalities that underlie malignancies of the female genital tract (vulva, vagina, cervix, uterus, ovaries, and fallopian tubes). It is clear that an improvement in outcome of these malignancies can only be achieved if: (1) early diagnosis is achieved, (2) there is accurate prediction of progression and response, and (3) new treatment options reflecting the molecular pathogenesis and progression are developed. This requires detailed disease-specific understanding of the diverse molecular changes in gynecologic malignancies that ultimately lead cells to develop the following hallmarks of cancer: abnormalities in self-sufficiency of growth signals, evasion of apoptosis, insensitivity to antigrowth signals, limitless replicative potential, sustained angiogenesis, and tissue invasion and metastases. Moreover, there is growing evidence for the concept of cancer immunosurveillance and immunoediting based on (1) protection against development of spontaneous and chemically induced tumors in animal systems and (2) the identification of targets for immune recognition of human cancer.1 This concept is supported by several studies in gynecologic cancers and has opened new avenues for the development of novel biomarkers and therapeutic targets. It is the purpose of this chapter to highlight and summarize some of the recent basic findings in gynecologic malignancies, with an emphasis on clinically applicable developments.
OVARIAN CANCER
Origins of Epithelial Ovarian Cancer
Until recently, epithelial ovarian cancer (EOC) was thought to arise primarily from the ovarian surface epithelium (OSE), with a subset likely originating in the adjacent fimbria.2,3 The OSE forms a monolayer surrounding the ovary, but is composed of relatively few cuboidal cells (107 cells per ovary, or 0.05% of the entire organ). Developmentally, it derives from the coelomic epithelium, which also gives rise to the peritoneal mesothelium and oviductal epithelium.4 The OSE appears generally stable, uniform, and quiescent, although it can undergo proliferation in vivo.5 Despite the small number of cells within the OSE and their apparent inactivity, the risk for EOC is nearly 2%, suggesting a high malignant potential. The basis for such a high potential is poorly understood. No physiologic role for the primate OSE has been established,6 and the lack of any obvious function could contribute to the asymptomatic nature of early-stage EOC.
In other organs, such as the colon, distinct premalignant lesions have been identified and found to accumulate genetic defects that ultimately result in malignancy. However, the search to identify similar epithelial precursors in the human ovary has proven only partially fruitful, in large part because normal ovaries are only rarely biopsied or examined. Histologic findings consistent with a preinvasive lesion for ovarian cancer have been described by a number of studies where ovaries were removed from women who eventually developed peritoneal carcinomas, ovaries from high-risk women who were undergoing prophylactic oophorectomy, and in areas of ovarian epithelium adjacent to early-stage ovarian cancers that demonstrate a transition from normal to malignant cells.7 Surprisingly, occult noninvasive and invasive carcinomas in the fallopian tubes, typically in the fimbria, have been discovered in women undergoing prophylactic salpingo-oophorectomies because of a family history or germ-line mutations of BRCA1 and BRCA2.8 This led to the hypothesis that these occult tubal carcinomas might shed malignant cells, which then implant and grow on the ovary, simulating primary ovarian cancer. Moreover, gene expression studies have demonstrated that the expression profiles of ovarian high-grade serous cancers (HGSCs) more closely resembled fallopian tube epithelium (FTE) than the OSE.9 Because the tubal carcinomas were associated with serous and not endometrioid, clear cell, or mucinous carcinomas, the noninvasive tubal carcinomas have been designated serous tubal intraepithelial carcinoma (STIC).
The new paradigm for the pathogenesis of ovarian serous cancer based on a dualistic model and the recognition that the majority of these tumors originate outside the ovary facilitates the development of novel approaches to prevention, screening, and treatment. The low-grade serous tumors (type I) are generally indolent, present in stage I (tumor confined to the ovary) and develop from well-established precursors, and are characterized by specific mutations, including KRAS, BRAF and ERBB2, but rarely TP53. They are relatively genetically stable. In contrast, the HGSCs (type II) are aggressive, present in advanced stage, and develop from STICs. They have a very high frequency of TP53 mutations, but rarely harbor the mutations detected in the low-grade serous tumors. Although low-grade serous and HGSCs develop along different molecular pathways, both types may develop from FTE and secondarily involve the ovary.10
Molecular Pathways to Ovarian Cancer
Inherited Syndromes of Ovarian Cancers
A family history of the disease is the most significant known risk factor for EOC. There is a threefold increased risk of developing the disease for an individual with a first-degree relative affected with ovarian cancer.11 Inherited EOC most often occurs as part of families with both ovarian and breast cancer cases or in families with only multiple ovarian cancer cases. Inherited ovarian cancer is also part of Lynch syndrome or hereditary nonpolyposis colorectal cancer (HNPCC), found in families with multiple cases of colon cancer. It is estimated that 5% to 10% of EOC cases are due to these familial syndromes.12
A linkage analysis of familial breast and ovarian cancers provided some of the first insights into the molecular basis of ovarian cancer, ultimately leading to the identification of two genes, BRCA1 and BRCA2, each associated with a significant increased incidence of ovarian cancer. The frequency of BRCA1 and BRCA2 mutations in the general population is estimated to be 1 in 800 and 1 in 500, respectively,11 whereas population-based ovarian cancer studies revealed that the frequency of BRCA1 mutations was 3% to 10% and for BRCA2, 0.6% to 6%.12
The BRCA1 gene was cloned in 199413 and was found to have 24 exons spanning 80 kb of genomic DNA, and had a 7.8 kb transcript coding for an 1,863 amino acid protein. The BRCA2 gene mapped to chromosome 13q12-13, had 27 exons (26 coding), spanning 70 kb of genomic DNA, and had an 11.4-kb transcript and coded a 3,418 amino acid protein.14 Hundreds of mutations in BRCA1 have now been identified, most commonly, loss of function nonsense or frameshift mutations. Two specific mutations, 185delAG and 5382insC, are found in 1% and 0.1%, respectively, of Ashkenazi Jewish women. Some missense changes have been found to be pathogenic, but the majority are polymorphisms or unclassified variants (UV), also known as variants of uncertain significance (VUS). A high frequency of large genomic rearrangements (LGR) have been identified in both BRCA1 and the BRCA2 gene.15
The position of mutations within large BRCA1 and BRCA2 genes determines the risk of ovarian cancer. The BRCA1 gene mutation within nucleotides 2402 and 4190 carries the high risk of ovarian cancer (and a lower risk of breast cancer). Both genes show an autosomal-dominant transmission of highly penetrant germ-line mutations and behave as tumor suppressor genes. Both proteins function in the double-strand DNA break repair pathway but may have additional functions; BRCA1 functions in both checkpoint activation and DNA repair, and BRCA2 is a mediator of homologous recombination.
Functionally, BRCA1 regulates p53, an oncogene frequently implicated in ovarian cancer. Thus, the loss of BRCA1 allows DNA damage to accumulate via a loss of its activation of p53. However, mutations in BRCA1 also likely contribute to ovarian cancer by mechanisms other than its interactions with p53. Any understanding of the role of BRCA1 in ovarian cancer is further complicated by reports of women with high-risk mutations in BRCA1 who fail to develop ovarian cancer. These observations speak clearly to the role of genetic modifiers in determining whether BRCA1 or BRCA2 mutations ultimately lead to malignancy. For example, the CAG repeat polymorphism in the androgen receptor has been shown to modify the subsequent risk of ovarian cancer in women with known mutations in BRCA1.
High penetrance BRCA1 and BRCA2 gene mutation accounts for about 40% of ovarian cancer risk.16 The remaining risk is due to very rare high risk genes, very few moderate risk genes, and/or multiple low risk genes. The Ovarian Cancer Association Consortium (OCAC) was established in 2005 to investigate the risk of SNP in ovarian cancer. So far, an additional six loci associated with a risk of ovarian cancer have been reported: 2q31, 3q25, 8q24, 9p22.2, 17q21, and 19p13.1.11
Genomic Instability, Fanconi Anemia/BRCA DNA Repair Pathway, and Rare Mutations
BRCA1 indirectly and BRCA2 directly are associated with maintaining the genome stability (i.e., a cell’s ability to tolerate DNA damage). BRCA2 is a member of BRCA/Fanconi anemia (FA) DNA repair pathway. Studies on the pathogenesis of FA, a rare inherited DNA repair disorder, have helped define the molecular basis of defective DNA damage responses linked to cancer risk. FA is a genetic disorder characterized by skeletal anomalies, progressive bone marrow failure, cancer susceptibility, and cellular hypersensitivity to DNA cross-linking agents. To date, 15 FA genes have been cloned: FANCA, -B, -C, -D1, -D2, -E, -F, -G, -J, -L, -M, -N, and -I, -O, and -P. Of these, FANCA, FANCB, FANCC, FANCE, FANCF, FANCG, FANCL, and FANCM form a nuclear core complex. Although the functional scope of this complex has not been fully defined, it is clear that it must be completely intact to facilitate monoubiquitination of the downstream FANCD2 and FANCI proteins, a change that allows FANCD2 and FANCI to colocalize with BRCA1, BRCA2 (and presumably FANCJ and FANCN), and RAD51 in damage-induced nuclear foci.17
Four lines of evidence link the FA pathway with ovarian carcinogenesis. First, BRCA2 has been identified as the FA gene, FANCD1. As a result, heterozygotes for BRCA2 mutations have a high risk of tissue-specific epithelial cancers, whereas homozygotes develop FA. Second, an increased prevalence of ovarian malignancies has been observed in FANCD2 nullizygous mice. Functionally silencing FANCF in ovarian cancer through promoter hypermethylation has also been described. Lastly, low levels of the FANCD2 protein are found in ovarian surface epithelia from women at risk for ovarian cancer. Taken together, these data suggest that the FA pathway is important in defining a predisposition to ovarian (and breast) cancer, and that aberrations of FA genes may account for some familial ovarian cancer cases not accounted for by BRCA1 and BRCA2 mutations.
In sporadic ovarian cancers, the epigenetic silencing of the FA pathway through methylation of the FA gene promoter region is one of the frequent mechanisms of inactivation. One study found that 4 of 19 primary ovarian carcinomas had FANCF methylation, although a larger study of 106 ovarian tumors did not identify the loss of FANCF expression. Loss of the BRCA2 mRNA and protein has been reported in 13% of ovarian carcinomas and, in contrast to other FA genes, methylation is not a cause of the protein loss. Epigenetic silencing of BRCA1 through methylation was found in 23% of advanced ovarian carcinomas.
Interestingly, tumors with inactivated BRCA2 are responsive to cisplatin. However, due to their low accuracy of DNA repair, these cells accumulate secondary genetic modifications that can lead to a reversal of the BRCA2 mutation, allowing these cells to acquire resistance to cross-linking agents.18
Recently, mutations have been identified in four genes: RAD51C, RAD51D, BRIP1 and PALB2 in the FA-BRCA pathway. Mutations in the RAD51C and RAD51D genes were found in approximately 1% of breast/ovarian cancer families. The relative risk (RR) of ovarian cancer in RAD51D carriers was 6.3 (95% confidence interval [CI], 2.9 to 13.9). Mutations in PALB2 (FANCN) were found in 3.4% of breast cancer families, and 55% of these families had family members with ovarian cancer. An Icelandic mutation in BRIP1 (FANCJ) with a frequency of 0.41% increased the risk ovarian cancer, OR 8.1 (95% CI, 4.7 to 14.0). A Spanish BRIP1 mutation had a frequency of 1.3% in ovarian cancer cases and the risk of ovarian cancer was OR 25 (95% CI, 1.8 to 340).11
In 2010, Walsh et al.19 screened 21 tumor suppressor genes in mutations in 360 unselected ovarian, fallopian tube, or peritoneal cancer cases and found mutations in six additional genes (BARD1, CHEK2, MRE11A, NBN, RAD50, and TP53).19 These findings suggest that a careful and comprehensive mutational screen of all women may identify that the inherited risk of ovarian cancer is higher than originally thought and that an early identification of the risk may ultimately lead to prevention in 24% of ovarian cancer cases.
Role of Poly (ADP-Ribose)-Polymerase (PARP) and PARP Inhibitors in Gynecologic Cancers
Abnormalities in DNA repair have been identified in many human epithelial cancers. Gynecologic cancers are not an exception, with ovarian cancer being the most extensively studied. Serous ovarian cancer has been characterized as having a major defect in homologous recombination resulting from mutations within Fanconi pathway, as noted previously. DNA repair is a complex process designed to repair specific abnormalities within the DNA. Single-stranded DNA breaks are repaired by a protein complex containing poly (ADP-ribose)-polymerase (PARP). In contrast, double-stranded breaks that are lethal for the cell require a different complex, which contains the BRCA proteins.
Because PARP proteins are intimately involved in the repair of single-stranded DNA breaks, the inhibition of PARP function impedes single-stranded DNA break repair, leading to the formation of double-stranded breaks. Research performed in multiple laboratories suggested that the inhibition of PARP would be a lethal event in cells that have inactivation of BRCA1 or BRCA2. This concept, called synthetic lethality (first proposed for cancer therapeutics in 1997), was tested early in the development of PARP inhibitors.20 Preclinical data strongly supported this concept, with BRCA-mutated cell lines showing a 100- to 1,000-fold sensitivity to PARP inhibitors compared with wild-type control cell lines. This laboratory proof of principle led to a rapid transition into the clinic.
The clinical development of PARP inhibitors began in earnest in the mid-2000s where they were tested in early phase trials. The first phase I trial focused on women with germ-line BRCA mutation of and yielded encouraging results. The expansion cohort of this study treated woman with ovarian cancer and recorded a 46% response rate in platinum-sensitive patients and a 33% response rate in platinum-resistant patients. Of note, the drug was well tolerated and its oral delivery made it quite convenient. This landmark trial led to a follow-up study that examined doses of 100 mg to 400 mg and involved ovarian cancer mutation carriers. The response rate was dose dependent (13% to 33%) and again demonstrated significant activity in the platinum-resistant disease. PARP inhibitors have also been tested in the maintenance setting after the effective treatment of platinum-sensitive recurrent disease. A dramatic improvement in progression-free survival was demonstrated with a hazard ratio (HR) of 0.15 for mutation carriers.21
Despite these results, the development of PARP inhibitors has been slow. Difficulties in formulation, precise dose determination, and the identification of pharmacodynamic endpoints have impeded determining the effective role of these agents. Further, a randomized phase II trial directly comparing a PARP inhibitor to doxorubicin in germ-line mutation patients with recurrent ovarian cancer showed no difference between the arms. Despite these setbacks, continued enthusiasm for these agents has led three companies to recently design phase III trials to establish their role in the treatment of ovarian cancer.
It is important to note that most of these trials have tested the effectiveness of PARP inhibitors in mutation carriers. Recent work form large genomic studies support that a much larger percentage of serous ovarian cancers have homologous recombination deficiencies. Estimates have been as high as 50% of all sporadic ovarian cancers. The precise genomic or protein biomarkers, which predict for responsiveness, is the focus of intense research.
There are important unanswered questions and challenges involving the effective use of PARP inhibitors. The precise optimal dose, duration, and sequence are yet to be determined. Should PARP inhibitors be used up-front with chemotherapy or later in maintenance or relapse disease? At present, there are little long-term safety data on PARP inhibition and, given their mechanisms of action, there is concern about secondary malignancies such as leukemia. This may be dependent on the mode of administration with chronic use versus intermittent being more problematic. Further, the mechanism(s) of PARP inhibitor resistance remains essentially unknown. Recent work has demonstrated an in-frame mutation in the BRCA gene that restored the protein function in a small number of resistant tumors. Other mechanisms are surely present and need to be determined. Finally, other potential clinical settings for PARP inhibitors, such as radiation sensitizers in combination with chemotherapy, for cervical or endometrial cancers will need to be evaluated.
Ovarian Cancer Microenvironment, Metastases, and Angiogenesis
The ovarian cancer microenvironment consists of any biologic component that interacts with tumor cells, and it includes stromal components, extracellular matrix (ECM) molecules, and cytokines.22 Together, these components create a microenvironment that interacts with the tumor and influences tumor behavior. Two hallmarks of cancer, angiogenesis and metastatic potential, are dependent on the tumor microenvironment. Targeting the interactions between the tumor and its environment has the potential to revolutionize the treatment for ovarian cancer patients.
Inflammatory Cytokines
Cytokine-mediator molecules have a profound effect on tumors. Tumor necrosis factor alpha (TNF-α) is a cytokine that is expressed on ovarian cancer cells and macrophages and is implicated in inflammation. It works through affecting other cytokines and angiogenesis.23 Animal studies showed that suppressing TNF-α resulted in lower plasma levels of interleukin (IL)-6, reduced IL-17 levels in the ascites, and reduced tumor burden.24 In a phase I clinical trial with the TNF-α antibody, infliximab, patients with ovarian cancer showed decreased IL-17 ascitic levels.24 In addition to TNF-α, high levels of IL-6, IL-8, and macrophage inflammatory protein are also found in ascites. In a multivariate analysis, IL-6 has shown to be a predictor of poor prognosis. A monoclonal antibody siltuximab, which abrogates the IL-6 signaling pathway, had been tested in a phase II clinical trial in platinum-resistant ovarian cancer with one third of the patients showing a clinically meaningful response.25
Particular attention has recently focused on the role of lysophosphatidic acid (LPA) in promoting the metastasis of ovarian cancers. LPA is constitutively produced by mesothelial cells lining the peritoneal cavity; its levels are increased in the ascites of women with both early- and late-stage ovarian cancers.26
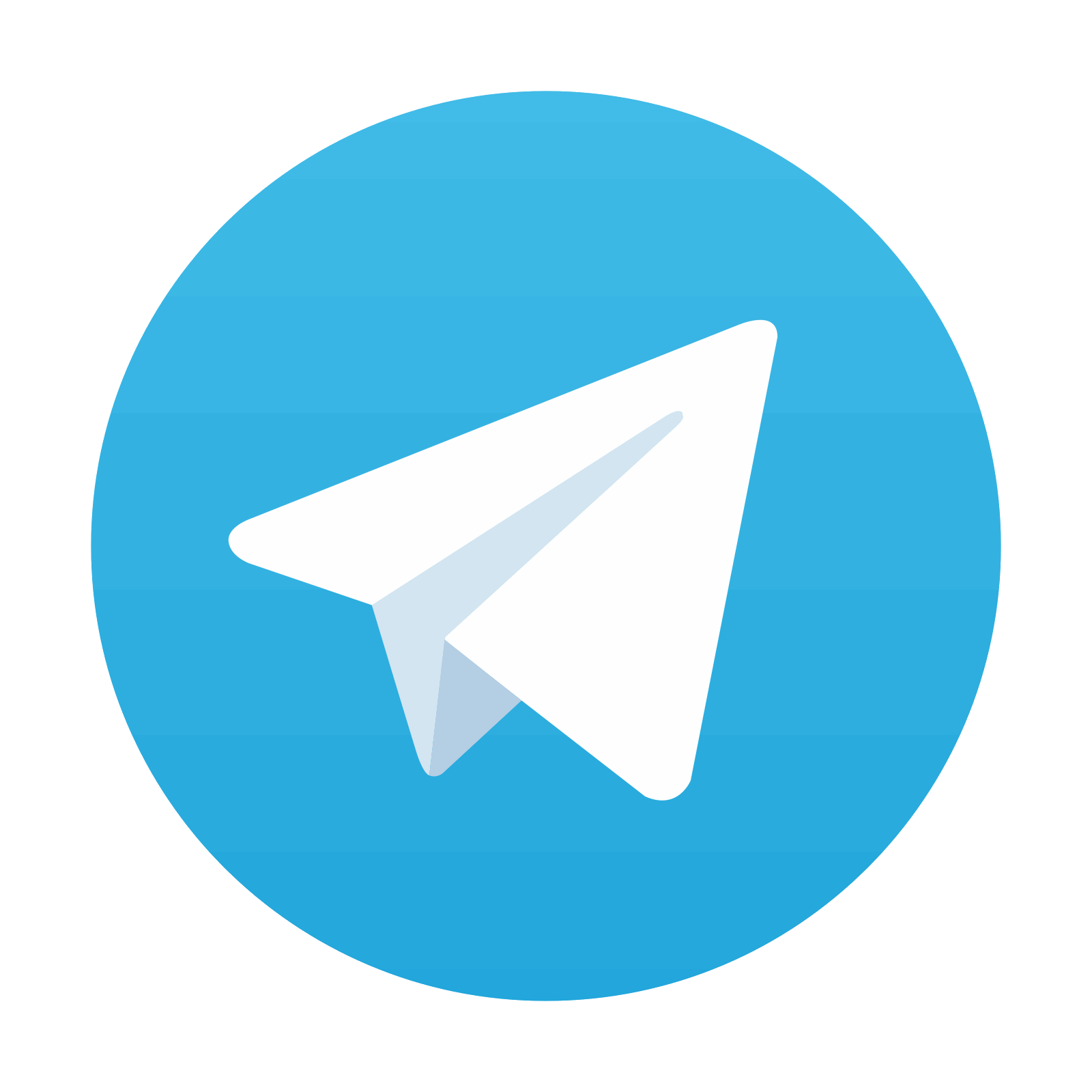
Stay updated, free articles. Join our Telegram channel

Full access? Get Clinical Tree
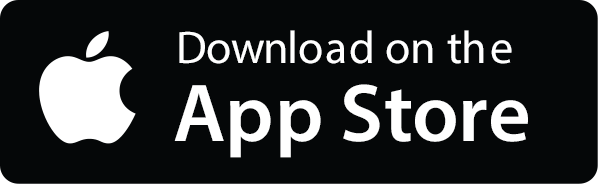
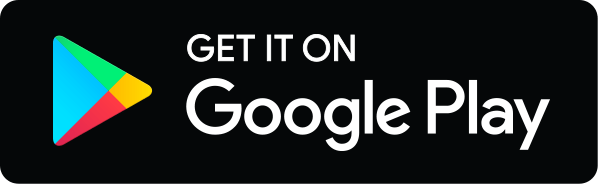