© Springer Science+Business Media New York 2015
Ashraf Khan, Ian O. Ellis, Andrew M. Hanby, Ediz F. Cosar, Emad A. Rakha and Dina Kandil (eds.)Precision Molecular Pathology of Breast CancerMolecular Pathology Library1010.1007/978-1-4939-2886-6_1212. Molecular-Based Diagnostic, Prognostic and Predictive Tests in Breast Cancer
(1)
Department of Histopathology, Division of Cancer and Stem Cells, School of Medicine, The University of Nottingham and Nottingham University Hospitals NHS Trust, Nottingham City Hospital, Nottingham, NG5 1PB, UK
Keywords
Breast cancerMolecular assaysDiagnosisPredictionGene expression profilingIntroduction
Generally speaking, a prognostic factor is any measurable parameter capable of providing information on patient clinical outcome, i.e. assessing the risk of disease recurrence at the time of primary diagnosis, independent of therapy. Prognostic factors are usually indicators of tumour growth, invasiveness and metastatic potential. A predictive factor is any measurable parameter capable of providing information on the likelihood of response to a particular therapeutic modality [1, 2]. A Prognostic/predictive factor could be either a single trait or signature of traits that can stratify patients into different population. Although prognostic and predictive factors could be separately classified, several factors in breast cancer provide both prognostic and predictive information [e.g. oestrogen receptor (ER) expression and human epidermal growth factor receptors 2 (HER2) overexpression]. Biological molecular prognostic and predictive variables are primary tumour molecular characteristics that reflect the underlying genetic abnormalities and their assessment, using different platforms, can be used to determine tumour behaviour and response to therapy.
ER, progesterone receptor (PR), HER2, tumour size, lymph node stage and histological grade are the existing practical prognostic and predictive parameters [3]. The last three prognostic parameters are combinatorially incorporated into the Nottingham Prognostic Index (NPI); [4], a well-recognised prognostic tool in breast cancer because of its simplicity and clinical utility [5]. However, there are increasing concerns that these parameters are not sufficient to assess prognosis and response to therapy in view of the diversity and heterogeneity of breast cancer behaviour. In addition, although positivity of hormonal receptors and overexpression of HER2 in breast cancer provide prognostic information and act as predictive parameters for the response of hormonal therapy and anti-HER2 targeted agents, respectively [6–8], there remains a need for further refinement of management decision. In recent years, personalised systemic therapy has become an increasingly required in patient management, especially in early stage breast cancer. Accordingly, there is a need to develop or update the existing prognostic and predictive classifiers.
Gene-expression profiling studies have attracted attention by demonstrating the presence of different molecular classes with clinical relevance and that breast cancer morphologic heterogeneity can be linked to specific molecular profiles. Most of these molecular profiling studies have identified at least four distinctive molecular/biological subgroups: two luminal subtypes; namely luminal A and luminal B, the HER2-enriched and the basal-like types [9, 10].
Despite the prognostic relevance of molecular taxonomies and the well-documented prognostic power of certain gene signatures, there remain technical and cost-effectiveness issues regarding their incorporation into routine practice. Previous studies have demonstrated that the behaviour of well-established clinical parameters varies in the different molecular classes. Therefore, performance of current clinical prognostic indices may not provide the same information within the different molecular classes. Adjustment of the performance of the clinical parameters and prognostic indices to the most recent advancement in molecular classification of breast cancer is considered a way forward for personalised patient management. An additional problem with this approach is the cost and feasibility of microarray and chip gene expression technology for routine management of breast cancer. Alternatively, immunohistochemistry (IHC) is considered a practical, cost-effective and reliable technique for molecular classification and the gold standard in the routine assessment of the essential predictive molecular biomarkers; ER, PR and HER2 [11, 12].
Hormonal Receptors: Oestrogen Receptor (ER) and Progesterone Receptor (PR)
The expression of ER and PR acts as a prognostic and a predictive parameters and assessment of their expression is an established standard procedure in breast pathology. Oestrogen receptor is one of the steroid receptor families and localised predominantly in the nuclear compartment [13, 14]. These receptors stimulated by steroid hormones as oestrogen which both are taken part in several processes such as suppression of apoptosis, cellular proliferation, angiogenesis and invasion [15].
The oestrogen receptors, which are normally expressed in breast tissue, are two types ER-α [16] and ER-ß [17], ER-α, ER-α gene includes six functional domains and encodes a 67 KDa protein [11], is connected directly to the pathological processes which one of them is breast cancer [18].
Several studies assessed the prognostic value of ER negative status and its association with decrease in survival in node negative patients with breast cancer [19–22]. In addition, its expression in breast cancer has a high clinical importance as a predictive parameter and existence of oestrogen receptor confers favourable biology because the expression of ER by tumour cells was related to the benefit of hormonal therapy. Patient with ER-negative disease cannot benefit from endocrine therapy while the ER positive group does. For instance, the Early Breast Cancer Trialists’ Collaborative Group represented that treatment of patients with tamoxifen; an anti-oestrogen therapy which can block cellular proliferation in breast cancer had an effect on the 5-years breast cancer mortality and recurrence [6]. Moreover, in a meta-analysis, tamoxifen and other selective oestrogen receptor modulators have shown an overall improvement by 38 % and 42 in the 10 years and 5 years follow-up, respectively [23]. Furthermore, several studies supported the relation between hormonal receptor status and response to chemotherapy as ER negative group (21–33 %) had a better response to neoadjuvant chemotherapy than the hormonal receptors positive group (7–8 %) [24–26].
ER expressed in 60–80 % of breast cancer cases at the same time most of the oestrogen receptors cases express PRs as well [27, 28]. However, the discrepancy between PR and ER expression are sometimes present [28, 29]. PR is an ER-regulated gene; therefore the expression of this receptor is highly related to the existence of the ER. ER−/PR+ is an uncommon variety, constituting less than 1 % of all breast cancers [30]. Some benefits had been reported from tamoxifen therapy in this ER−/PR+ sub-group while no effect of PR receptor in oestrogen receptor positive cases [31].
Hormone receptor and HER2 also provide important diagnostic information in breast cancer. Hormone receptor positivity in primary or metastatic carcinoma is considered as strong diagnostic clue to breast origin. ER is expressed in both breast carcinomas and tumours of gynaecological origin. Although aberrant ER expression may be observed in other tissues such as lung carcinoma and colorectal carcinoma, the expression is usually weak and focal. For a diagnostic purpose, ER is often combined with other biomarkers based on initial assessment of the index tumour. Markers that can be used to determine origin of carcinoma with ER expression include CDX2 (colorectal), PAX8 (gynaecological tumours), TTF1 (lung and thyroid carcinoma), S100 and HMB45 (Melanoma). Interpretation of these markers is usually considered in combination together with morphology of the tumours and none of these markers alone can provide absolute specificity. Other diagnostic biomarkers for breast cancer include ER-related genes such as GATA3. As a diagnostic marker ER-alpha must be used; ER-beta is not specific to the breast and its expression can be seen in different organs. Other diagnostic breast biomarkers include GCDFP-15, mammaglobin and lactalbumin. Gross cystic disease fluid protein-15 (GCDFP-15) is a marker of apocrine differentiation that is considered to be specific to the breast, but it has low sensitivity and is also expressed in apocrine glands and submandibular salivary glands. Mammaglobin, a mammary-specific member of the uteroglobin family that is known to be overexpressed in human breast cancer; however, it is also expressed in some non-breast cancer sites such as endometroid carcinomas, endocervical adenocarcinoma in situ and sweat gland carcinomas.
Human Epidermal Growth Factor Receptor 2 (HER2)
HER2 is a member of the transmembrane receptors epidermal growth family (EGFR) of receptors; EGFR-/ErbB1, HER2/ErbB2, HER3/ErbB3 and HER4/ErbB4 [32]. Overexpression of HER2 occurs in 13–20 % of breast cancer cases, up to half of these cases are ER negative [33, 34]. Overexpression of HER2 is a well-established poor prognostic indicator of invasive breast cancer [27–29]. HER2 is the main predictive factor for anti-HER2 targeted therapy including Herceptin as well as other HER2 dual inhibitor tyrosine kinase such as Lapatinib which is dual inhibitor of HER2 and HER1 and HER dimerization inhibitor such as pertuzumab [35–37]. HER2 can also predict response to certain chemotherapeutic agents [38]. In addition to its prognostic value as a single marker with HER2 positive Herceptin naïve tumours show the worst outcome in breast cancer, HER2 when combined with hormone receptor with or without Ki67 can provide addition prognostic information similar to multiparameter prognostic gene signatures [39].
HER2 is also used to diagnose Paget’s disease of the breast in which it is expressed in more than 90 % of Paget’s cells; therefore, it can be used to differentiate Paget’s disease from melanocytic lesion and Bowen’s disease of the nipple. In addition HER2 amplification in breast tumours can be used to indicate malignancy as benign tumours do not show HER2 amplification. Some benign tumours show weak to moderate HER2 membrane staining.
Ki67 Expression
Cell proliferation is considered as one of the well-recognised prognostic factors in breast cancer [40]. Nuclear positivity of Ki67 protein is an indication of cellular proliferation and that the cells are in the proliferative pool/entered cell cycle but not necessarily in mitosis [41, 42]. Ki67 expression has long been reported as a prognostic marker in breast cancer [43–46]. Breast cancer positive for Ki67 is associated with worse prognosis than those tumours negative for Ki67. In retrospective analysis, we and others reported better response to adjuvant therapy in tumours with higher expression levels of Ki67 than those tumour having low levels of Ki67 [46, 47]. However, these results need validation on prospective basis. Importantly, Ki67 expression has been reported as an independent predictive factor for neoadjuvant chemotherapy in breast cancer patients [48, 49] as well as the neoadjuvant endocrine therapy in postmenopausal patients [50]. Patients with high post-treatment Ki67 expression levels were reported to have higher risk for disease relapse and death more than those with low or intermediate Ki67 expression [51]. In 2013, the St. Gallen International Breast Cancer Conference reinforced the addition of Ki67 into the definition of intrinsic subtype of breast cancer [52]. The most significant prognostic value of Ki67 in breast cancer is seen in ER+ luminal class and in grade 2 tumours. Ki67 has limited prognostic value in ER− and triple negative tumours. Consistent with its prognostic value, some centres have introduced Ki67 in the routine diagnostic practice.
Gene Expression Profiling
The introduction of modern technologies having the ability of simultaneous analysis of thousands of genes in a single assay combined with rigorous analytical approaches gave an opportunity for classifying breast cancer into distinct groups depend on gene expression profiles. This approach developed our understanding of breast cancer and opened new avenues for novel prognostic/predictive signatures in human breast cancer.
Breast Cancer Molecular Classes
Gene-expression profiling studies initially have led to invasive breast cancer classification into four distinctive molecular groups as discussed in Chap. 10. These molecularly classified subgroups have distinct clinical outcomes, with luminal A having the most favourable outcome, followed by luminal B, basal-like, and lastly the HER2-enriched [10, 53]. In addition to the prognostic value of this molecular classification, there is some evidences indicating that luminal A sub-group is particularly sensitive to endocrine therapy and it has a more positive natural history than HER2 and basal-like subgroups in spite of the sensitivity of HER2 subtype tumours to the chemotherapy [54]. Molecular classification of breast cancer was thought to provide predictive information superior to that provided by ER and HER2 alone. This concept was based on the fact that the classification of tumours within each class is based on several hundreds of genes reflecting the molecular portraits of the tumour. Global gene expression profiles are expected to provide more accurate information on the biological feature of the tumour and reflect the activity of important pathways and interaction of key driving molecules, and subsequently determine the behaviour of the tumour and response to specific therapy better than that provided by a single molecule. In addition, molecular classes were expected to provide information for resistance of some tumours to specific therapy such as HER2 positive tumours not responding to HER2 targeted therapy may be classified as luminal or basal tumours using gene expression profiling. Similar ER positive tumours that are assigned to basal or HER2 positive classes may explain resistance to endocrine therapy. However, the predictive value of molecular classes over ER and HER2 status remain limited and currently not used in clinical practice to predict response to targeted therapy. Molecular classification of breast does not have diagnostic value.
Prognostic Gene Signatures
Oncotype DX [21-Gene Genomic Health Recurrence Score (GHI-RS)]
A multistep approach was followed to develop a commercially available robust reverse transcriptase-polymerase chain reaction (RT-PCR) assay of 21 prospectively selected genes for invasive breast cancer. The initial step used a real-time RT-PCR to assess the gene expression from formalin-fixed paraffin-embedded (FFPE) tissue sections. The initial 250 genes were selected from genomic databases, along with review of literature and DNA array-based experiments (using fresh-frozen tissues). To test the correlation between recurrence of breast cancer and gene expressions of these 250 candidates, data from 447 patients from three independent clinical studies of breast cancer was analysed. Using the results of these studies, a panel of 21 genes (16 cancer-related genes and 5 other reference genes) was selected. Depending on the levels of expression of these genes, an algorithm was designed to calculate a recurrence score (RS) for each sample. The cancer-related genes include genes of the ER group (ER, PR, SCUBE2 and BCL2), HER2 group (HER2 and GRB7), cell proliferation group (Ki67, CCNB1, Survivin, STK15 and MYBL2), invasion group (MMP11 and CTSL2) and GSTM1, BAG1 and CD68. The reference genes include ACTB (b-actin), GAPDH, RPLPO, GUS and TFRC. The expression of these 21 genes was presented as a recurrence score (RS); a continuous variable ranging between 0 and 100, where higher scores reflect a greater possibility of recurrence. The RS was divided into three categories: low risk (<18); intermediate risk (≥18, but <31) and high risk (≥31) [55]. The Oncotype DX breast cancer assay is designed to predict the 10-year risks of breast cancer recurrence in women with ER positive newly diagnosed node negative early stage (I–II) breast cancer, treated with tamoxifen. Oncotype DX is more than just a risk assessment tool: it can provide further information, including to what extent the woman would benefit from chemotherapy as well as tamoxifen therapy [56].
MammaPrint
MammaPrint is a diagnostic classifier developed by Van’t Veer and colleagues, who identified a 70-gene signature significantly associated with prognosis in breast cancer patients using inkjet-synthesised oligonucleotide microarrays. The initial study was applied on 98 lymph node negative breast cancer patients younger than 55 years, with primary breast tumour size less than 5 cm [57]. The subsequent study of a cohort of 295 was validated for both lymph node negative and positive breast cancer cases [58]. MammaPrint can predict the probability of distant metastases within 5 years, and it can divide breast cancer patients into two groups with significantly different distant metastasis-free survival. In a good profile group, patients are more likely to remain free of distant metastases, while those classified as a poor profile group have a high risk of developing distant metastases [57, 59]. MammaPrint was the first FDA approved, gene expression-based prognostic test for stage I–II lymph node negative breast cancer [60].
Furthermore, an exploratory study resulted in the prediction that gene expression signatures (including the 70-gene signature) developed on frozen tissue showed a high level of concordance between fresh-frozen and FFPE matched pairs, [61] as validated by a subsequent study [62]. Recently in 2015, the MammaPrint as a breast cancer test using FFPE was FDA approved [63]. Importantly, the “Microarray in Node negative Disease may Avoid ChemoTherapy (MINDACT)” phase III randomised trial, prospectively tests the of MammaPrint assay, in parallel with Adjuvant! Online tool, on 6600 breast cancer patients form 9 countries [64]. The results of pilot study of MINDACT concludes that proportion of discordantly classified patients, the potential reduction in chemotherapy using the genomic signature, and compliance to treatment assignment are in accordance with the trial hypotheses are in accordance with the trial hypotheses [65].
Genomic Grade Index (GGI)
Sotiriou et al. used five datasets of gene expression with total number 661 breast cancer patients. They examined the association between the histologic grade and gene expression profiles of breast cancers for the reason of improving histologic grading by measuring the expression of 97 genes. Therefore, instead of the classic grades 1, 2 and 3, GGI divides histologic grade into low and high risk. The proposed gene expression grade index was able to reclassify the intermediate grade of ER positive cases into a high and low gene expression grade index [53]. Moreover, the GGI ability to predict response to neoadjuvant chemotherapy in ER positive and ER negative evaluated patients [66].
Breast Cancer Index (BCI)
It is a RT-PCR test of the ratio of two genes expression, HOXB13 and IL17BR, joined with Molecular Grade Index (MGI) to test the risk of recurrence for ER+ and LN negative early invasive breast cancer patients. Initially, a genome-wide microarray analysis of frozen tumour specimens of 60 ER positive breast cancer patients treated with tamoxifen alone was performed [67]. Then, a two-gene expression ratio was identified which is highly predictive of clinical outcome. This expression ratio adapted to use analysis based on PCR of standard FFPE, which was established using an independent set of 20 FFPE tissues samples [68]. The BCI risk score ranges from 0 to 10 and divides patients into three categories, When the score is <5 (low risk group); between 5 and 6.3 (intermediate risk group); ≥6.4 (high risk group) [69]. Subsequent studies have demonstrated that both genes (HOXB13 and IL17BR) have a prognostic as well as a predictive value [70–72].
PAM50 (Prediction Analysis of Microarray 50)
This a gene expression assay using 50 genes (PAM50), representing a reduced gene set assayed by quantitative real-time reverse transcription-PCR (qRT-PCR). It accurately identifies the four intrinsic biological/molecular subtypes of breast cancer and generates risk-of-relapse (ROR) scores. Moreover, its prognostic value in both untreated and tamoxifen-treated patient populations has been confirmed [73, 74]. A combined prognostic marker that includes the proliferation genes of PAM50 and tumour size identifies a subpopulation with an excellent outcome if treated with hormonal therapy alone and produces a score of estimating a probability of disease recurrence [75]. The PAM50 test was further adapted to be performed to develop a simplified workflow that could be easily and efficiently to measure gene expression in a local pathology lab (Prosigna™ Breast Cancer Gene Signature Assay, NanoString Technologies, Seattle) in frozen or FFPE tissues [76–78].
Next-Generation Sequencing (NGS)
Nucleic acid sequencing is a method for determining the exact order of nucleotides present in a given DNA or RNA molecule. Over the past decade, the use of nucleic acid sequencing has increased exponentially following attempt to complete human genome sequence and increasing demand for cheaper and faster sequencing methods. This demand has driven the development of next-generation sequencing (NGS) which perform massively parallel sequencing, during which millions of fragments of DNA from a single sample are sequenced in unison. NGS allows fast sequencing of the entire genome and it provides very sensitive quantifying applications including gene expression analysis more than traditional microarray-based methods. NGS can allow high-throughput sensitive analysis of the genome transcriptome and epigenome which is expected to provide important prognostic and predictive information. In addition it can provide information about the molecular nature of the tumour and detect previous unknown mutation that can useful in diagnosis and prediction of response to specific therapy. In breast, 45 regions of sequence alterations are demarcated by Curtis et al. to have a role in the development of the cancer [79]. The whole-genome sequencing data provide an overall view of individual tumour and understanding of the full catalogue of somatic genetic changes will be the way for personalised treatment of breast cancer patients [80].
Circulated Tumour Cells
Circulating tumour cells (CTC) are cells that originate from the primary tumour and circulate through blood stream and greatly contribute to the metastatic spread of cancer [81]. Currently, detection and molecular characterisation of CTCs in breast cancer patients is an active area of translational breast cancer research. Different detection systems have been developed for characterisation and enumeration of CTC with acceptable sensitivity and specificity. For instance, the protein-based CellSearch® system (FDA-USA cleared), the functional EPISPOT assay (for EPithelial ImmunoSPOT) [82], Ephesia-chip [83] and others [84, 85]. In addition, mRNA-based assays targeting specific mRNAs (e.g. CK19 mRNA), and the highly sensitive RT-qPCR assay (e.g. Adna-Test) [86], are the widely used alternatives to identify CTCs [87].
Different studies have reported CTC as a reliable prognostic factor in patients with early stage and metastatic breast cancer, irrespective of the CTC detection method and time point of blood sample withdrawal [88, 89]. Some studies also showed that CTC load in peripheral blood is associated with shorter survival in patients with early breast cancer [90, 91]. Moreover, CTC can be used as an effective independent prognostic factor before and after neoadjuvant chemotherapy [92]. CTC detection is able to monitor efficacy of adjuvant therapies [93], and assess therapeutic responses of advanced disease earlier than traditional imaging methods [94]. In addition to the analysis of peripheral blood CTC, some studies have assessed the prognostic value of breast cancer cells in bone marrow aspirates and demonstrated a correlation with outcome [95].
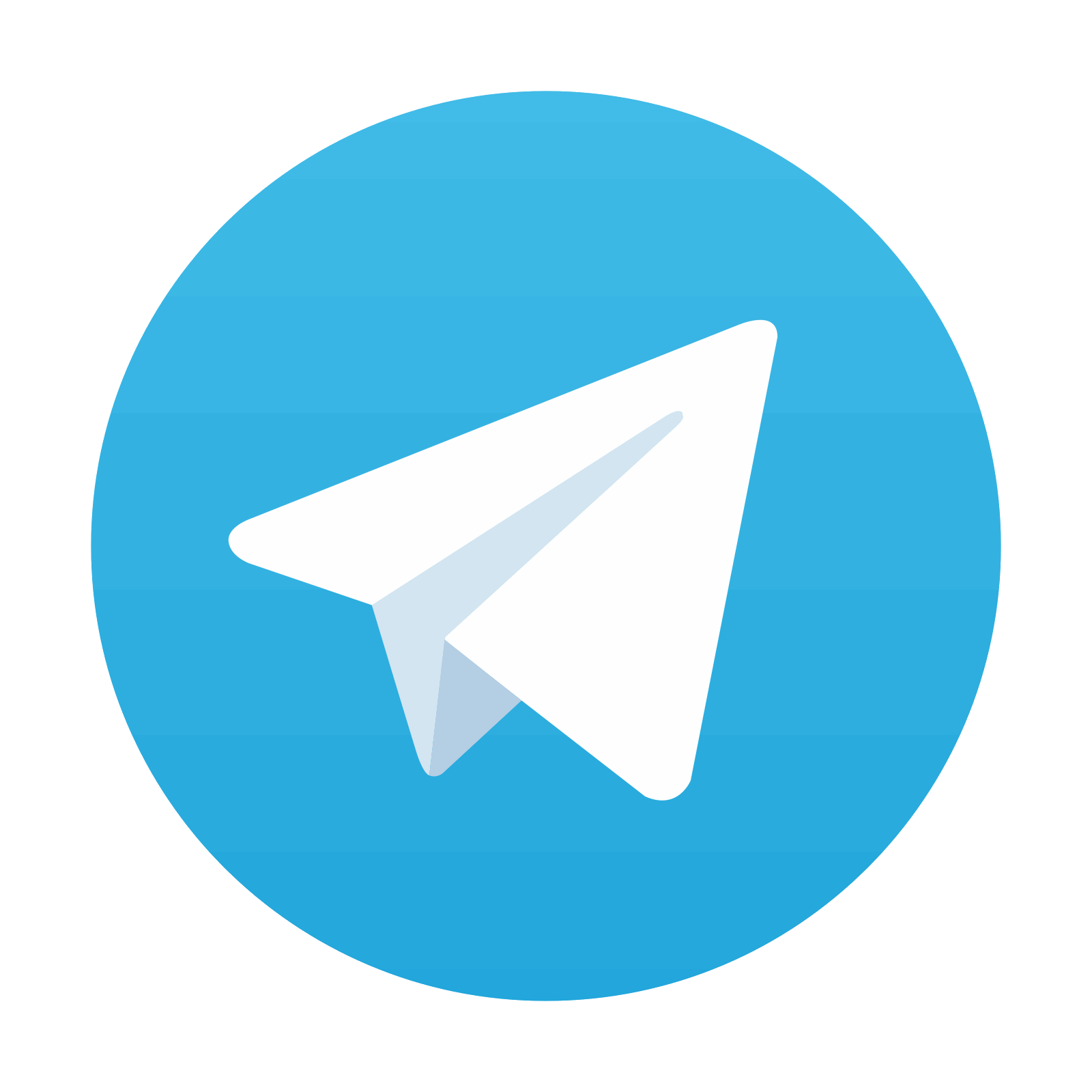
Stay updated, free articles. Join our Telegram channel

Full access? Get Clinical Tree
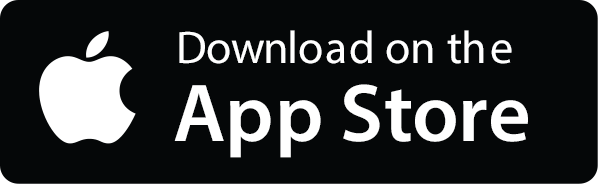
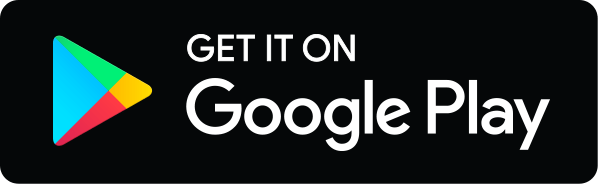