Fig. 1
Schematic overview of various genes targeted to provoke CIN in vivo
3 In Vivo Consequences of CIN
In the last two decades, a large number of mouse models for chromosome instability have been engineered. Hereunder, we summarize the findings from these models asking the following questions:
1.
Is CIN a bona fide instigator of cancer?
2.
Which genes collaborate with CIN in vivo converting aneuploid cells into aneuploid cancer cells?
3.
What are other consequences of CIN in vivo?
4 Can CIN Initiate Cancer?
CIN has detrimental consequences for cells grown in vitro (Kops et al. 2004; Williams et al. 2008; Torres et al. 2008), yet, two out of three human tumours are aneuploid (Weaver and Cleveland 2006; Duijf et al. 2013). This raises the question whether CIN is an initiating factor in cancer, a facilitator or merely a side effect of tumorigenesis. In the vast majority of all models (see Fig. 1), full inactivation of the targeted genes resulted in early embryonic lethality. Although the time of embryonic death varied between genotypes (Table 1), embryos were typically lost before embryonic day 10, which presumably was the result of aneuploidy in the inner cell mass of the developing embryos (Dobles et al. 2000; Weaver and Cleveland 2006; Foijer et al. 2008; Holland and Cleveland 2009; Schvartzman et al. 2010). To circumvent embryonic lethality, phenotypes of heterozygous mice were monitored, or in some cases, conditional alleles were engineered. Even though tumour phenotypes have been reported for many of these models (Table 1) tumour incidence is relatively low, with in most cases fewer than 50 % of the mice developing cancer. Moreover, tumours only arise late in the life of the mice, with latencies typically ranging from 12 to 24 months (Table 1). The most frequent pathologies observed include lymphoma, lung and liver tumours. Furthermore, not all models develop spontaneous tumours, for instance in case of the Bub family members [Bub1, Bub3, Rae1 (Kalitsis 2000; Babu et al. 2003; Wang et al. 2004; Baker et al. 2006a; Jeganathan et al. 2007; Baker et al. 2009)]. There is no clear correlation between the severity of the tumour phenotypes and the mechanism that drove CIN in the mice (i.e. SAC mutation, cohesion defects, centrosome abnormalities etc.). Expression levels of the CIN-provoking genes on the other hand appear to be a better predictor of tumour incidence: phenotypes were most severe in cases where CIN-driving proteins were overexpressed to high levels [e.g. Mad2, Cyclin B1, Cyclin B2, Hec1, Plk4 (Ko et al. 2005; Sotillo et al. 2007; Baker et al. 2008; Diaz-Rodríguez et al. 2008)] possibly because the relative effect on protein expression (several folds overexpression) was more dramatic than in heterozygous mice, where protein levels were typically reduced ~50 %. However, as tumour latency is high in these models as well, additional hits must be required for aneuploid cells to become malignant.
Table 1
List of various mouse models engineered to provoke CIN in vivo, with phenotypes and observed aneuploidy levels in vivo and in vitro where quantified
Group | Gene | −/− | ± | Cancer predisposed (chemical or genetic collaboration) | Aneuploidy in tissues | Aneuploidy in MEFs | Implicated in human cancer (Schvartzman et al. 2010) | References |
---|---|---|---|---|---|---|---|---|
Spindle assembly checkpoint | AuroraB | EL | >60 %; 24 mo | Tumour suppression upon DMBA + TPA-induced (not sig.) | ND | ND | Fernández-Miranda et al. (2011) | |
Bub1 | EL (E6.5) | VNODD | DMBA-induced-57 %, p53+/−; 16.6 mo | ND | ND | |||
Bub1 hypomorph | n/a | 50 %; 12 mo | 78 %, p53+/−; 12 mo | ND | 15 % (seg. defects) | |||
Bub3 | EL (E6.5) | VNODD | DMBA-induced | 10 % (splenocytes) | 20 % | |||
Bub3; Rae1 | ND | VNODD | DMBA-induced | 40 % (splenocytes) | 40 % | |||
BubR1 | EL (E6.5) | VNODD | DMBA-induced | Polyploidy in megakaryocytes | 15 % | Yes | ||
BubR1 hypomorph | n/a | Premature ageing | DMBA- and azoxymethane-induced | 30 % (splenocytes) | 35 % | Yes (MVA) | Baker et al. (2004) | |
BubR1 overexpression | n/a | Delayed ageing | DMBA-induced, but decreased susceptibility than WT | 1 % (splenocytes) | 9 % (WT comparable) | Baker et al. (2013a) | ||
Ccnb1 (Cyclin B1) overexpression | n/a | >75 %; (lung, lymphoma, liver, lipoma) | ~80 %, APC+/min; 40 % (WT comparable; skin), DMBA-treatment | hi 20 %, lo 12 % (splenocytes) | 31 % (ctrl. 15 %) | Nam and van Deursen (2014) | ||
Cdc20 AAA mutant (does not bind to Mad2) | EL (E12.5) | 50 %; 24 mo | ND | 35 % (Cdc20AAA/+, splenocytes) | 28 % (Cdc20AAA/+ and 52 % of Cdc20AAA/AAA) | Li et al. (2009) | ||
Cdh1 | EL (E10.5) | 17 % females – (mammary); mild brain abnormalities and altered behaviour | Tumour suppression upon TPA/DMBA treatment | ND | Increased (not quantified) | García-Higuera et al. (2008) | ||
CENPE | EL (<E7.5) | 20 % (lung, spleen); 19–21 mo | Tumour suppression upon DMBA treatment or p19Arf loss | 40 % (splenocytes) | 20 % (up to 70 % at high passage) | |||
Mad1 | EL | 20 % (lung); 18–20 mo | Vinicristine-induced | ND | 10 % | Iwanaga et al. (2007) | ||
Mad2 | EL | 30 % (lung); 18 mo | ND | ND | 55 % | |||
Mad2 overexpression | n/a | 50 % (lymphomas, lung and liver); 20 mo | DMBA-induced | Aneuploid tumours (not quantified) | 50 % | Yes | Sotillo et al. (2007) | |
Mps1 (T-cell restricted) | VVNOD | ~50 % (lymphoma) 17 mo | 100 %, p53+/−; 5 mo | >90 % of cells aneuploid | ND | Foijer et al. (2014) | ||
Rae1 | EL (E6.5) | No spont. tumorigenesis | DMBA-induced | 10 % (splenocytes) | 20 % | |||
Ubch10 overexpression | n/a | Expression level dependent: 40–80 % (lymphoma, lung adenoma, lipoma and liver and skin) | Yes, but not significantly different compared to wild type | 4–19 % hi-lo, 5 mo (splenocytes); 52–64 % (lymphoma) | 28–33 % (WT 13 %) | Yes | van Ree et al. (2010) | |
Kinetochore | CENPA | EL (E6.5) | VNODD | ND | Chromosome missegregation in E6.5 CENPA−/− embryos | n/a | Howman et al. (2000) | |
CENPB | VNODD | VNODD | ND | ND | ND | |||
CENPC | EL (E3.5) | VNODD | ND | Aberrant mitosis and micronuclei in early embryos | n/a | Kalitsis et al. (1998) | ||
Hec1 overexpression | n/a | 13 % (lung), 26 % (liver); 67 wk, 60 wk | ND | ND | 25 % | Yes | Diaz-Rodríguez et al. (2008) | |
Cohesion | Espl1 (separase) | EL (E6.5) | Epsl1+/H; VNODD | 86 % (lymphomas), p53−/−; 4 mo—50 % (carcinoma), p53+/− | 57 % (splenocytes); 84 % (bone marrow) | ND | Yes | Mukherjee et al. (2011) |
Espl1 overexpression (mammary restricted) | n/a | 80 % (mammary), 11 mo | 100 % (mammary), p53+/−; 14 mo | >80 % (mammary tumours) | ND | Mukherjee et al. (2013) | ||
Stag1 (exon 3 and 4, encoding SA1-cohesin subunit) | EL (between E12.5 to E18.5) | 40–50 % (haematoma, lung, fibrosarcoma, liver, vascular, pancreas); 24 mo | Resistance to 3MC and DEN induced fibrosarcomas and liver tumours | 40 % (fetal liver) | >70 % | Remeseiro et al. (2012) | ||
Pttg (securin) | Reduced testis, spleen and thymus weight. | n/a | Tumour protective, pRb+/− | ND | 15 % (WT 1 %) | |||
Pttg (securin) overexpression | n/a | Enlarged pituitary; altered nuclear morphology | >80 % (pituitary), pRb+/−; 10 mo | ND | ND | |||
Chromosome passengers | APC/MIN | EL (<E8.5) | Intestinal tumours; 3 mo | ND | Aneuploidy and abnormal mitosis in crypt cells | Increased, not quantified | ||
Incenp | EL (3.5–8.5) | VNODD | ND | Abnormal nuclear morphology hyperdiploid content in E3.5 embryos | n/a | Uren et al. (2000) | ||
Survivin | EL (6.5) | VNODD | ND | Giant nuclei in early embryos | n/a | Uren et al. (2000) | ||
Centrosome | Aurora A overexpression (mammary restricted) | n/a | Increased p16 expression | 45 %, p53−/− (mammary gland); 4.5 mo | ND | 13.6 % | ||
Plk1 | EL (E10.5) | 27.5 % (lymphoma, lung); 12.5–17.5 mo | 100 % (lymphoma, lung), p53−/− | 12 % (splenocytes) | ND | Lu et al. (2008) | ||
Plk4 overexpression (CNS restricted) | n/a | Microcephaly, 100 % post-natal lethality; <1 wk | 100 % lethality, p53−/; 5 mo | 31.7 % centrosome amplification (neural stem cells); >60 % aneuploidy of chr. 18 in p53−/− | ND | Marthiens et al. (2013b) | ||
Usp44 | VNODD | Usp44+/− 20 %, Usp44−/− 50 %; 15 mo (lung, liver, lymphoma, sarcoma) | n/a | 8 %, 5 mo; 16 %, 15 %, 15 mo (splenocytes) | 18 % (WT 13 %) | Yes | Zhang et al. (2012) | |
Otherwise involved in mitosis | Ccnb2 (Cyclin B2) overexpression | n/a | >70 % (lung, lymphoma, liver, lipoma); 14 mo | >80 %, APC+/min; >80 % (lung), DMBA-treatment | 18 % (splenocytes) | 36 % (ctrl. 16 %) | Nam and van Deursen (2014) | |
Chfr | VNODD | Chfr−/− 50 %; 20 mo | DMBA-induced | ND | 25 % | Yu et al. (2005) | ||
Mcm4 Chaos3 | Chaos3/− EL (E14.5) | Mcm4Chaos3/+ (mammary); 12 mo | ND | ND | ND | Shima et al. (2007) | ||
Tpx2 | EL (E8.5) | 53 % (lymphoma, lung) | no | 18.3 %, 16 wk; 27 %, 90 wk (splenocytes) 48.9 %, 90 wk (lymphomas) | Aguirre-Portolés et al. (2012) |
5 Does CIN Predispose to Cancer?
Exposure to carcinogens is a powerful tool to assess tumour predisposition in vivo. Given the relative weak tumour phenotypes of CIN mice, various CIN models were exposed to carcinogens (Table 1) to assess whether CIN is a powerful collaborator in transforming cells. Indeed, carcinogens aggravated the tumour phenotypes of some of the CIN mice, more than their control counterparts. For instance, when Mad1 heterozygous mice were treated with Vincristine (a microtubule-depolymerizing agent), 40 % of the mice developed mostly lung tumours, while no tumours were detected in control-treated mice (Iwanaga et al. 2007). Likewise, carcinogens (NMBA or DMBA) accelerated tumorigenesis in Lzts1-deficient and Chfr-deficient mice (Yu et al. 2005; Vecchione et al. 2007). Furthermore, even in CIN mice without a tumour phenotype (e.g., Bub1+/−, Bub3+/−, Rae1+/− and Bub3+/− Rae1+/−), DMBA treatment had a stronger tumour promoting effect than on wild type mice (Baker et al. 2006b; Jeganathan et al. 2007). As carcinogens reduce tumour latency and increase tumour incidence in a CIN background, also these experiments indicate that additional mutations are required for a CIN cell to transform into a malignant cell.
6 Which Genes Collaborate with CIN in Cancer?
To test which genetic alterations collaborate with CIN in tumorigenesis, various CIN models were crossed into cancer-predisposed backgrounds. For instance, when CIN was combined with p53 heterozygosity, [Bub1, Espl1, Mps1 (Baker et al. 2009; Mukherjee et al. 2011; Baker et al. 2013b)] tumour incidence dramatically increased while tumour latencies decreased. In all reported cases, tumours had lost the remaining p53 wild type allele, indicating that full p53 loss and CIN synergize in tumorigenesis (Baker et al. 2009; Foijer et al. 2014). However, as CIN further increased tumour incidence of p53null mice, CIN must have facilitated cancer formation through additional genomic alterations as well. Furthermore, CIN provoked by Bub1 hypomorphic alleles or Cyclin B1 overexpression accelerates tumours in a Apcmin background (Baker et al. 2008, 2009). However, in other tumour predisposed backgrounds (e.g., pRb or Pten heterozygousity) CIN has no effect on tumour incidence (Baker et al. 2009).
7 CIN as a Tumour Suppressor
In some cases CIN can also act in a tumour suppressive manner. For instance, CIN driven by SA1 heterozygosity delays 3-methyl-colanthrene (3-MC)-induced fibrosarcoma and diethyl-nitrosamine (DEN)-induced liver tumours (Remeseiro et al. 2012). Similarly, even though Cdh1+/− mice and CenpE+/− mice are more susceptible to spontaneous tumours, they are more resistant to carcinogenic insults than their wild type counterparts (Weaver et al. 2007; García-Higuera et al. 2008). Furthermore, CIN can also delay tumorigenesis in some genetically predisposed models, for instance by delaying p19Arf or Pten loss-driven tumours (Weaver et al. 2007; Baker et al. 2009). Why then is CIN tumour promoting in one setting, but tumour suppressive in another? The answer might lie in the levels of CIN. CIN is quite toxic and provokes an ‘aneuploidy stress’ response in untransformed cells (Kops et al. 2004; Torres et al. 2007; Williams et al. 2008; Foijer et al. 2013). However, aneuploid cancer cells also exhibit this stress response (Dürrbaum et al. 2014; Foijer et al. 2014), suggesting that aneuploid cancer cells still suffer from the disadvantageous effects of CIN. Therefore, the levels of CIN occurring in premalignant cells could be a determining factor for the outcome. The fact that p19Arf loss provokes aneuploidy itself fits with this hypothesis, as CenpE heterozygosity would exacerbate CIN to a level that is toxic for cancer cells (Silk et al. 2013). However, further experiments are required to determine at what level CIN is beneficial for cancer cells and at what level the balance is tipped.
8 What Other Phenotypes Are Provoked by CIN?
There is increasing evidence that aneuploidy also occurs in untransformed tissues, with liver being the most well-known example. Up to half of both human and murine hepatocytes are aneuploid (Duncan et al. 2012a, b), but it is unclear why hepatocytes evolved to become aneuploid. One suggestion is that particular karyotypes are selected for during hepatotoxic insults, making the hepatocytes more resistant to injury (Duncan et al. 2012b). Other studies quantified over 30 % of normal human neuroblasts to be aneuploid (Rehen et al. 2001, 2005), which has been suggested to contribute to the plasticity of neurons (Kingsbury et al. 2005). However, when provoked in a random fashion, CIN appears to mostly have disadvantageous effects on brain functioning, as mice heterozygous for Cdh1 exhibit defects in neuromuscular coordination and learning (García-Higuera et al. 2008). The interfollicular epidermal cells in mouse skin on the other hand appear to cope surprisingly well with CIN as they tolerate full abrogation of the SAC provoked by Mad2 loss, which results in dramatic aneuploidy (Foijer et al. 2013). The hair follicle stem cells that reside in the same compartment do not cope at all and disappear, resulting in mice with functional skin, but without hair (Foijer et al. 2013). Together these data clearly indicate that CIN is tolerated by some cell lineages, but not others, underscoring the importance of in vivo modelling.
9 Linking Ageing and CIN In Vivo
Ageing is the time-dependent functional decline in the fitness of cells, organs and organisms. A common hallmark of ageing is genomic instability, as exemplified by genetic alterations in old blood cells (Forsberg et al. 2012; López-Otín et al. 2013). Some of the CIN mouse models also suggest a role for aneuploidy in ageing. For instance, BubR1 hypomorphic mice are not only prone to severe aneuploidization, but also display progeroid pathologies. Similar to BubR1, combined Bub3/Rae1 haploinsufficiency also results in a premature ageing phenotype, albeit less severe than the BubR1 hypomorphic mice (Baker et al. 2006b), MEFs isolated from BubR1 hypomorphic mice express various ageing-associated markers such as p53, p21, p19Arf and p16Ink4a. Interestingly, when p16Ink4a positive cells are killed in vivo using a p16Ink4a-promotor regulated suicide construct, ageing pathologies induced by a reduction of BubR1 protein levels are dramatically delayed (Baker et al. 2011). The pathologies observed in BubR1 hypomorphic mice mimic those of patients with multi-variegated aneuploidy (MVA), a disease that frequently coincides with mutations in BUB1B, the gene encoding BUBR1 (Hanks et al. 2004, 2006; Matsuura et al. 2006). Furthermore, BubR1 expression levels decline with age providing further evidence for a role of BubR1 in ageing (Baker et al. 2011) in mice. Even more striking, when BubR1 is overexpressed, a dose-dependent delay in the onset of ageing is observed, as well as protection against developing chemically-induced tumours (Baker et al. 2013a). As discussed above, in most tested cases overexpression of CIN-controlling proteins increases CIN and predisposes for cancer (Sotillo et al. 2007; Diaz-Rodríguez et al. 2008; Fernández-Miranda et al. 2011). Apparently, BubR1 is the exception that forms the rule, but future work should reveal whether BubR1 has a unique role in the SAC or whether it has additional roles that can explain the beneficial effects of an overdose of BubR1.
10 What Have We Learnt from Modelling CIN in the Mouse so Far?
As most tumours are aneuploid to some extent, CIN makes an attractive target for therapy. For this, understanding how CIN is signalled is crucial. A large number of mouse models have been engineered over the last 15 years specifically for this purpose, with a wide variety of phenotypes summarized in Table 1. Even though many of the targeted genes will have other roles than safeguarding faithful chromosome segregation, some common conclusions can be drawn from the cumulative data. The first important conclusion is that CIN alone is not sufficient for efficient tumourigenesis and that CIN alone mostly has disadvantageous effects on cell proliferation. This has important implications for therapy targeting aneuploid cancer, as discussed below. A second conclusion is that CIN facilitates tumourigenesis efficiently in some tumour-predisposed backgrounds, chemical or genetic. However, when CIN is aggravated and becomes too severe, it can actually suppress tumour formation in the mouse, which can also be exploited in cancer therapy. A third and perhaps the most important conclusion is that several unaddressed questions remain before we can develop therapeutic strategies targeting aneuploid cell progeny, some of which are discussed below. Although all models discussed here were designed to study the consequences of CIN in vivo, the majority mimic a situation that is not typically found in human cancers, as loss of genes that regulate chromosome segregations are rarely lost in human cancer (Schvartzman et al. 2010; Foijer 2012). Even though those models mimic chromosome missegregation and its consequences, overexpression of CIN-modulating genes is more common [e.g., Mad2 overexpression, which is seen in many tumours (Hernando et al. 2004; Sotillo et al. 2007)]. Possibly, mimicking the CIN-provoking mutations that are found in human cancers would result in a physiologically more relevant CIN level, thus adding to our understanding of CIN and its role in tumorigenesis. A lot can be learned about affected pathways from in vitro studies as well. For instance, Donnelly and colleagues have shown that increased HSF1 activity can play a facilitating role in coping with aneuploidy-induced proteotoxic stress by regulating the gene expression of various heat shock proteins (Donnelly et al. 2014)
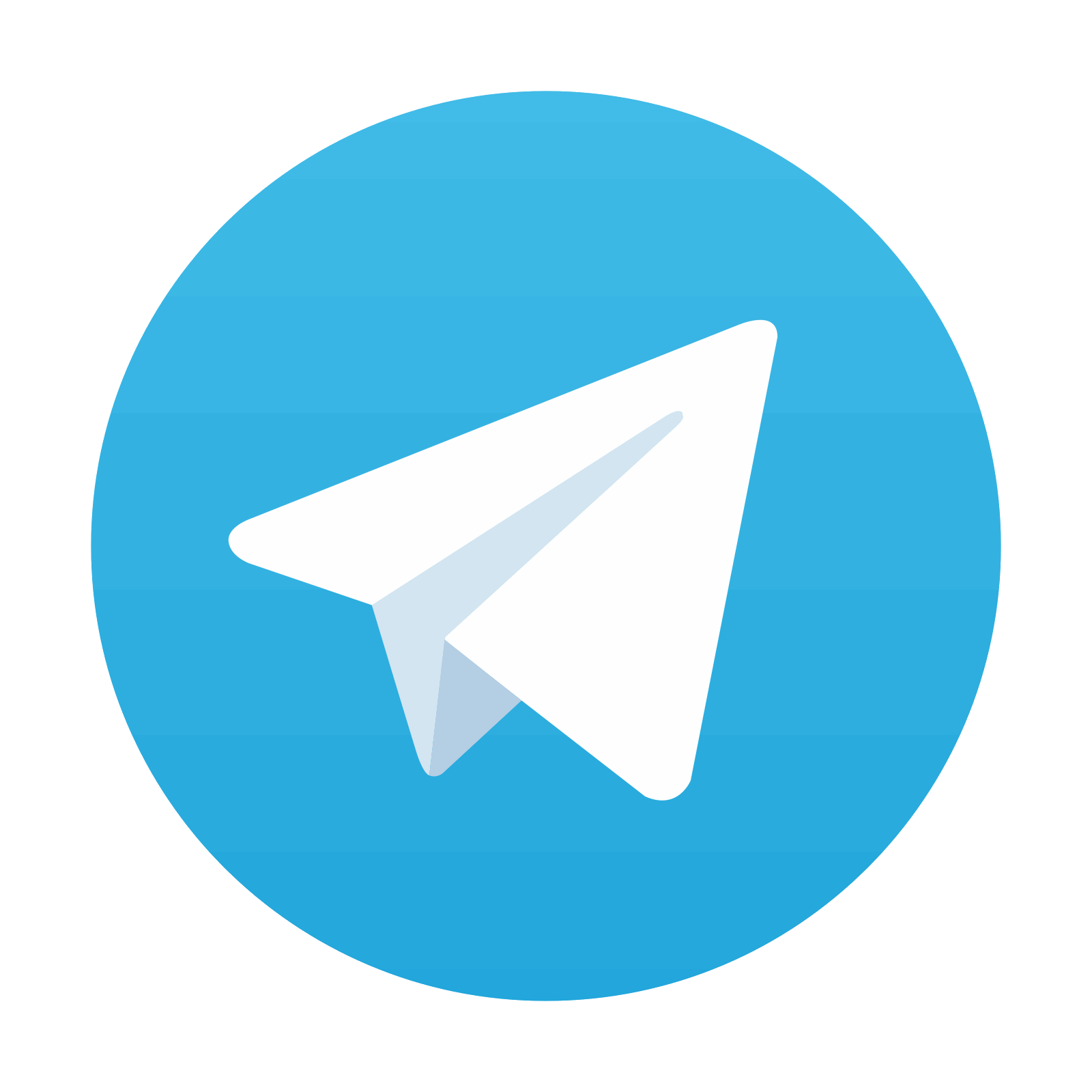
Stay updated, free articles. Join our Telegram channel

Full access? Get Clinical Tree
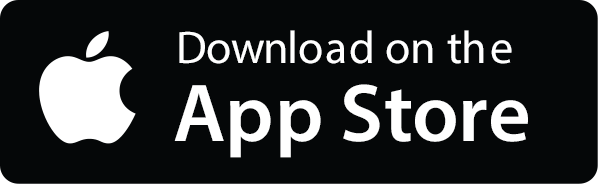
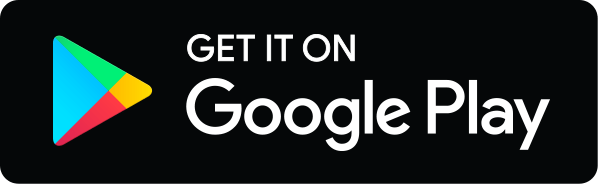