Fig. 8.1
Cross-sectional imaging demonstrating solitary hepatic metastatic disease. a and b Preoperative PET-CT showing segment IVb/V mass. c Restaging following four cycles of neoadjuvant 5-fluorouracil, leucovorin, oxaliplatin, and bevacizumab, showing decreased size of metastatic lesion
Epidemiology
Colorectal cancer is the fourth most frequently diagnosed cancer and the second leading cause of cancer death in the United States. In 2016, approximately 39,000 new cases of rectal cancer will be diagnosed and 49,000 patients will die from colorectal cancer combined [1]. Between 50 and 60% of patients diagnosed with colorectal cancer will develop metastatic disease [2] with 80–90% of patients having unresectable hepatic metastatic burden [3]. Most patients will present with metachronous liver metastatic disease. However, like our patient, 15–34% of patients with colorectal cancer present with synchronous liver metastatic disease [4–8].
Preoperative Planning
The management of patients who present with colorectal cancer with synchronous metastatic disease is complex. Decisions regarding timing of surgery, chemotherapy or chemoradiotherapy, and surgical approach are influenced by tumor biology, primary tumor location, extent of hepatic metastatic disease, and patient comorbidities. All patients should undergo a comprehensive history and physical examination. Prior abdominal surgery or the presence of underlying comorbidities, such as chronic obstructive pulmonary disease or congestive heart failure, should be kept in mind when choosing patients for minimally invasive hepatectomy.
Standard workup of patients with hepatic malignancy includes a triphasic CT scan (noncontrast, arterial, and portal venous phase) or contrast-enhanced MRI exam of the abdomen and pelvis. These imaging studies should be recent (within 4–6 weeks of surgery), and allow for evaluation of tumor size and location, extent of hepatic disease, assessment of the background liver for cirrhosis and hepatic steatosis, and the size of the future liver remnant. Evaluation for extrahepatic disease can be accomplished with a CT scan of the chest or CT/PET imaging. Routine labs include complete blood count, coagulation panel, and hepatic function panel. The serum CEA is useful as part of the diagnostic workup and postoperative surveillance.
Similar to open hepatectomy, we favor a low central venous pressure (CVP) anesthesia approach when performing minimally invasive hepatic resections [11]. This avoids distention of the inferior vena cava, facilitating mobilization of the liver and dissection of the retrohepatic cava and major hepatic veins. Low CVP anesthesia decreases bleeding during parenchymal transection and simplifies control of inadvertent venous injury. The incidence of gas embolism is rare during laparoscopic liver resection [12].
General Clinical Pearls
The management of metastatic colorectal cancer should always involve a multidisciplinary team of physicians.
The decision for synchronous versus staged resection and neoadjuvant therapy versus up-front resection should be guided by tumor biology and patient factors.
Always perform intraoperative ultrasound
The operative approach (minimally invasive vs. open, laparoscopic vs. robotic-assisted) is probably the least important decision in the comprehensive management of patients with Stage IV colorectal cancer. Decisions should be guided by surgeon experience and institutional resources.
Management
All patients with metastatic colorectal cancer should be discussed by a multidisciplinary panel of physicians, including colorectal and hepato-pancreato-biliary (HPB) surgeons, medical oncologists, radiation oncologists, interventional radiologists, gastroenterologists, pathologists, and palliative care providers. For our patient, the consensus of the multidisciplinary tumor board was to initiate systemic chemotherapy with 5-fluorouracil, leucovorin, oxaliplatin, and bevacizumab, with restaging after four cycles (2 months). There is no survival benefit to more than 2–3 months (four to six cycles) of preoperative chemotherapy, but liver-related posthepatectomy complications increase [13].
There is no consensus regarding the optimal management of patients with synchronous colorectal cancer and liver metastases. One option is the staged approach, addressing the primary first, followed by chemotherapy and hepatectomy at a later date. Theoretically, this approach eliminates potential complications from the primary tumor, such as bleeding, perforation, or obstruction. However, these complications arise in fewer than 10% of patients presenting with Stage IV colorectal cancer who receive systemic treatment in the setting of an intact primary [14]. Addressing the primary first delays the treatment of liver metastastic disease, which is of the greatest risk to long-term survival. Conversely, the liver metastatic disease can be addressed first, followed by colectomy and adjuvant chemotherapy. Alternatively, the patient can receive preoperative chemotherapy, followed by simultaneous or staged resection. A recent meta-analysis found that no single approach was superior to the others in terms of postoperative morbidity, mortality, or 5-year overall survival [6]. Given the lack of prospective data to guide decision-making, the order of therapy should be dictated by the extent of surgery that would be required to clear both the primary and the liver metastases [15]. For patients with less disease burden, consideration should be given to up-front simultaneous resection of both the primary and the liver metastatic diseases (i.e., solitary liver metastasis with a right colon cancer). Patients who would require extensive surgery to clear either the primary (i.e., proctosigmoidectomy or abdominoperineal resection) and/or the liver metastases (i.e., formal lobectomy or extended hepactectomy) warrant preoperative chemotherapy in an attempt to downstage disease and the extent of surgery required. Depending on the response, simultaneous or staged approaches can then be undertaken.
Minimally Invasive Hepatic Resection
Our patient completed 2 months of neoadjuvant chemotherapy and was restaged by contrast-enhanced CT scan. She had a partial clinical and radiographic response in both her primary rectal and rectosigmoid cancers (not shown) as well as in her solitary segment IVb/V liver metastasis (Fig. 8.1c). The decision was made to forgo neoadjuvant chemoradiotherapy for her distal rectal primary as the sphincter preservation was not an option; reducing the likelihood of pelvic recurrence was not a priority in the setting of end colostomy and the high risk of further distant disease recurrence. The patient underwent a robotic-assisted abdominoperineal resection with end colostomy and simultaneous cholecystectomy and segment IVb/V partial hepatectomy.
Our approach to robotic-assisted right and left anatomic lobectomy as well as left lateral sectionectomy has been previously described [16]. For robotic-assisted partial hepatectomy, patient positioning is generally supine, with arms tucked and in split-leg position. An orogastric tube and Foley catheter should be placed. In this specific case, the patient was in lithotomy with arms tucked because of the combined colorectal procedure. A cholecystectomy was performed and the gastrohepatic ligament was opened. A vessel loop was placed around the porta hepatis for control of vascular inflow (Fig. 8.2a). Intraoperative ultrasound (Fig. 8.2b) confirmed a solitary liver metastasis in segment IVb/V and no other lesions. Glisson’s capsule was scored with cautery (Fig. 8.2c) and intraoperative ultrasound was repeated, confirming the target lesion was centered within the planned wedge resection. The vessel loop was tightened to perform a Pringle maneuver. A traction suture was placed through the specimen (Fig. 8.2d) to assist with exposure, and parenchymal transection was performed with bipolar forceps using the crush–clamp technique (Fig. 8.2e). The Pringle was released, the cut surface was inspected for bleeding, and hemostasis was achieved. The patient had an uneventful postoperative course and was discharged on postoperative day five. She has completed eight additional cycles (4 months) of 5-fluorouracil, leucovorin, oxaliplatin, and bevacizumab and currently has no evidence of recurrent disease.
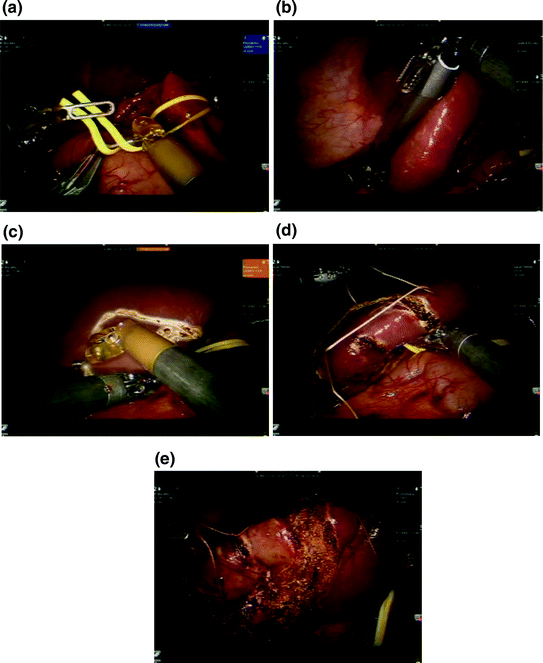
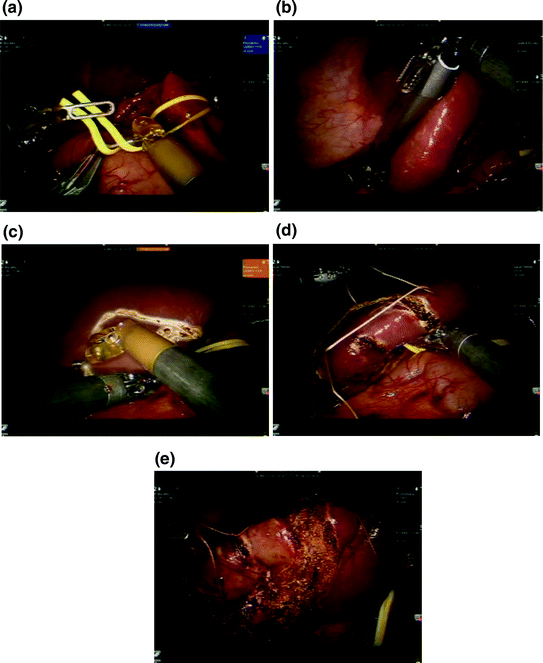
Fig. 8.2
Robotic-assisted partial hepatectomy. a The gastrohepatic ligament is opened and a vessel loop is placed around the hilar structures for control of vascular inflow. b Intraoperative ultrasound to confirm target lesion location and assess remaining liver. c The liver capsule is scored with cautery to declare the plane of transection and include the target lesion. d A traction suture is placed on the specimen to assist with exposure. Additional traction sutures may be placed on the remnant side as needed. e Parenchymal transection using bipolar forceps. A clamp crush technique is utilized. Larger pedicles can be ligated with endovascular staplers or electrothermal bipolar vessel sealing devices
Key Steps of Robotic-Assisted Partial Hepatectomy
Creation of pneumoperitoneum
Port placement
Mobilization of target hemiliver from ligamentous attachments as needed
Intraoperative ultrasound
Score the liver capsule and confirm target on ultrasound
Traction sutures on specimen
Inflow control—Pringle maneuver
Parenchymal transection and specimen retrieval
Assess for hemostasis after Pringle released
The application of minimally invasive techniques has transformed the surgical landscape and has demonstrated benefit in surgical subspecialties including colorectal surgery [17, 18], gynecology [19], urology [20], and thoracic surgery [21]. Multiple studies have demonstrated reduced postoperative pain, reduced morbidity, decreased length of stay, improved cosmesis, and improved overall cost-effectiveness without compromising oncologic outcomes [17, 18, 22–24]. Limitations of conventional laparoscopic techniques include reduced visualization, amplification of physiologic tremor, and suboptimal ergonomics. Range of motion is restricted to four degrees of freedom, compared to the seven degrees of freedom of the human wrist [25, 26]. These limitations become increasingly apparent as the complexity of the procedure increases, and have likely slowed the application of minimally invasive approaches to HPB procedures, the majority of which are performed at high-volume tertiary care centers.
As the safety of HPB surgery has grown and outcomes have improved, minimally invasive approaches have been applied with increasing frequency to the field [27–37]. Laparoscopic liver surgery affords the same universal benefits of minimally invasive surgery elsewhere, including reduced postoperative pain and decreased length of hospital stay; and it has demonstrated safety in experienced hands [23]. However, the laparoscopic approach to the liver is challenging, due to complex vascular and biliary anatomy, risk of bleeding, fragile parenchyma, and difficult exposure secondary to size and deep, posterior retroperitoneal attachments. The minimally invasive approach is being utilized more frequently, but mainly for nonanatomic resections. Nguyen et al. reviewed more than 2,800 laparoscopic liver resections, and nonanatomic wedge resections and left lateral sectionectomy comprised nearly two-thirds of cases, while fewer than 10% of cases were formal right or left hepatic lobectomies [23].
In an effort to standardize and summarize the current position on laparoscopic liver surgery, an international conference was held in Louisville, Kentucky, USA in 2008 [38]. Consensus recommendations included: (1) the best indications for laparoscopic liver resection are in patients with solitary lesions, 5 cm or less, located in peripheral liver segments 2–6; (2) the laparoscopic approach to left lateral sectionectomy should be considered standard practice; and (3) although all types of liver resection can be performed laparoscopically, major liver resections (right or left hepatectomy) should be reserved for experienced surgeons already skilled at more complex laparoscopic hepatic resections. Lesions adjacent to major vessels or near the liver hilum were not considered appropriate for laparoscopic resection because of the potential risk of massive bleeding and need for biliary reconstruction. However, surgeons at high-volume centers may choose to operate beyond these criteria, provided that the surgeon is comfortable with minimally invasive methods to achieve hemostasis should significant bleeding be encountered. Despite the technical limitations of laparoscopy, malignant tumors are not a contraindication to minimally invasive resection, as demonstrated in many comparative studies; and laparoscopic resection does not appear to compromise the oncologic integrity of the procedure with regard to margin status and local recurrence rate when compared to the open approach [23, 33, 39, 40].
The inherent visual and ergonomic limitations of laparoscopy have played a major role in the development of robotic surgery, which allows surgeons to perform advanced laparoscopic procedures with greater ease. Currently, the da Vinci Surgical System (Intuitive Surgical, Inc, Sunnyvale, CA, USA) is the only commercially available robotic surgical system, approved by the United States Food and Drug Administration (FDA) for use in surgery. Advantages include articulating instruments that recreate the seven degrees of freedom of the human wrist, three-dimensional view of the operative field in high definition, and complex algorithms that minimize physiologic tremor. These features allow for precise dissection and intracorporeal suturing, thus expanding the scope and complexity of procedures that can be performed in a minimally invasive fashion. Disadvantages include high cost, loss of haptic feedback, inability to operate in multiple fields, and need for a skilled bedside assistant. The lack of haptic feedback is generally overcome by enhanced three-dimensional visualization, which allows the operating surgeon to “see” how much tension or force is being applied to tissues and suture within the operative field [41].
The first major series reporting the use of robotics in general surgery was by Guilianotti et al. in 2003 [42]. The report included 207 procedures such as fundoplication, cholecystectomy, esophagectomy, colectomy, pancreatectomy, and hepatectomy. Morbidity and mortality rates were acceptable (8.3 and 1.5%, respectively), and the conversion rate was 2.1%. This report established that robotic surgery was both safe and feasible. Over the past decade, robotic-assisted surgery has been applied broadly in urological, gynecological, HPB, and cardiac surgery [36, 43–45]. The platform has controls and ergonomics that closely mimic the movements of open surgery, has improved three-dimensional visualization, and appears to shorten the learning curve for complex cases compared to conventional laparoscopy [42, 46, 47]. The multitude of technically challenging HPB procedures provides an ever-expanding and ideal application of this technology [34, 36, 48, 49].
Alternative Approaches and Controversies
The robotic platform is an advanced laparoscopic tool—depending on surgeon experience and skill level, all robotic-assisted procedures can be performed with pure laparoscopy.
Minimally invasive hepatectomy should only be performed by experienced HPB surgeons.
Ablation techniques (radiofrequency or microwave) can be used in conjunction with partial hepatectomy for smaller lesions not amenable to partial hepatectomy. Local recurrence rates may be higher but overall survival does not appear to be different.
Outcomes
Minimally invasive hepatectomy is being performed with increasing frequency. In the largest review of laparoscopic liver resections to date, Nguyen et al. analyzed case series totaling more than 2,800 cases [23]. The overall findings suggest that minimally invasive liver resection is both safe and feasible. Nearly 75% of the cases were performed purely laparoscopic. Nearly 50% of resections were for malignancy, and the majority of cases (65%) were minor resections, including wedge hepatectomy or left lateral sectionectomy. Formal lobectomy was performed in 15% of cases, and fewer than 1% of the cases were extended lobectomies. Perioperative death occurred in 0.3% of the patients. The overall complication rate was 11%, with the most common complications being bile leak (1.5%) and transient hepatic dysfunction (1%).
Several series have compared laparoscopic to open major liver resection [50–53]. Laparoscopic resections appear to be associated with less blood loss, fewer postoperative complications, and shorter hospital length of stay, with the trade-off being slightly longer operative times. Short- and long-term oncologic outcomes of laparoscopic liver resections are comparable to disease-matched open outcomes [54, 55].
There are eight series in the literature that compare robotic-assisted liver resection to laparoscopic liver resection [34, 49, 56–61]. Data are summarized in Table 8.1. The largest experience belongs to the group of Tsung et al. at the University of Pittsburgh [34]. Each of the 57 patients who underwent robotic hepatectomy were retrospectively matched in a 1:2 ratio to patients that underwent laparoscopic liver resection, with emphasis on background liver disease, extent of resection, diagnosis, American Society of Anesthesiologists (ASA) score, age, body mass index (BMI), and gender. There was no difference in blood loss, transfusion requirements, conversion rate, complication rate, or length of stay. Robotic-assisted procedures took significantly longer (253 vs. 199 min). There appeared to be a learning curve effect when comparing the initial 13 robotic procedures to the subsequent 44, as there were significant reductions in blood loss (300 vs. 100 ml), operative times (381 vs. 232 min), and length of stay (5 vs. 4 days). However, the comparison of robotic-assisted to surgery to conventional laparoscopy may not be worthwhile, because the robotic platform should be looked at as an advanced tool to overcome the inherent limitations of conventional laparoscopy, thus expanding the repertoire of complex minimally invasive procedures that can be applied to the liver, pancreas, and biliary tract.
Table 8.1
Perioperative outcomes of robotic-assisted hepatectomy versus laparoscopic hepatectomy
Tsung et al. (2014) | Tranchart et al. (2014) | Troisi et al. (2013) | Wu et al. (2014) | Yu et al. (2014) | Spampinato et al. (2014) | Packiam et al. (2012) | Berber et al. (2010) | |||||||||||||||||
---|---|---|---|---|---|---|---|---|---|---|---|---|---|---|---|---|---|---|---|---|---|---|---|---|
LA | RA | p | LA | RA | p | LA | RA | p | LA | RA | p | LA | RA | p | LA | RA | p | LA | RA | p | LA | RA | p | |
Major resection (n) | 42 | 21 | NS | 0 | 0 | NS | 37 | 0 | <0.05 | 10 | 20 | NR | 11 | 3 | NR | 25 | 25 | NS | 0 | 0 | NR | 0 | 0 | NR |
Minor resection (n)
![]() Stay updated, free articles. Join our Telegram channel![]() Full access? Get Clinical Tree![]() ![]() ![]() |