Fig. 6.1
Canonical and alternative miRNA biogenesis pathways. Most miRNA are transcribed by RNA Polymerase II to form long primary transcripts (pri-miRNA) which form complex structures that are then recognized and processed into precursor miRNA (pre-miRNA) by the microprocessor complex that includes the DGCR8 and RNAse III enzyme Drosha . These pre-miRNA hairpins are transported into the cytoplasm by exportin-5 and a Ran GTPase. In the cytoplasm, pre-miRNA are further processed into ~22 base pair duplexes by another RNAse III enzyme, Dicer , in association with GW182 and TAR RNA binding protein (TRBP). The mature miRNA then gets incorporated into the RISC complex, where it can then bind to the 3′-untranslated region (UTR ) of target mRNA to block translation or degrade the transcript, or both. Also illustrated is the biogenesis of miRNA through the non-canonical pathways, including an intronic splicing mechanism. In these cases, the pre-miRNA is generated directly bypassing the need for Drosha and DGCR8, afterwards the remaining steps of processing are similar to the canonical miRNA maturation pathway. Another alternative process occurs specifically with miR-451, this miRNA utilizes the beginning steps of the canonical pathway however, instead of Dicer the pre-miR is processed by Ago2 in the cytosol
In addition to this canonical pathway of miRNA biogenesis, several non-canonical pathways have been identified. A subset of miRNA called “mirtrons”, do not depend on Drosha for their initial processing (Fig. 6.1). These miRNAs are processed from introns of transcripts after splicing (Ruby et al. 2007). The lariat-debranching enzyme involved in splicing resolves the RNA branch site and results in a pre-miRNA hairpin that gets exported to the cytoplasm like other pre-miRNA to be further processed by Dicer (Berezikov et al. 2007). Yet another alternative miRNA biogenesis mechanism can be seen in miR-451, which is processed in a Dicer independent fashion. In the case of miR-451, pre-miR-451 gets loaded into argonaute-2 (Ago2) instead of Dicer, and is cleaved by the Ago2 catalytic center where it is then trimmed into a mature miRNA (Cheloufi et al. 2010).
Regardless of the mechanism of biogenesis, the mature miRNA are bound to the RISC to affect their biologic action within the cell. The RISC complex contains numerous proteins, but the primary effector of miRNA-mediated repression reside within a family of proteins known as the argonaute proteins (Gregory et al. 2005). The human genome contains eight argonaute-family proteins, but Ago2 is the only one with RNA cleavage activity and is thought to play the lead in miRNA-mediated silencing. Proteomics approaches have identified dozens of proteins that associate with Ago and RISC, and the combination of protein partners in this complex controls the regulation of RISC activity and the degree of miRNA-mediated repression (Hock et al. 2007). Association of the mature miRNA with RISC and the cognate mRNA at the 3′-UTR can result in repression of translation, or destabilization of the mRNA through decapping and degradation. The mechanistic details about these two methods of post-transcriptional repression remain an area of intense investigation (Wilczynska and Bushell 2015).
Mature miRNA can themselves be subjects to regulation in several ways. The mature miRNA transcript can be bound by other RNA transcripts called competing endogenous RNA (ceRNA) (Tay et al. 2014). Another class of RNA that can affect levels of mature miRNA are called circular RNA (circRNA), which are formed by a covalent link between the 5′ and 3′ ends of an exon by the spliceosome (Hentze and Preiss 2013). Both the ceRNA and circRNA can function as sponges and titrate mature RNA from the mRNA targets they may bind to. The function of these RNA families in the ovary has not yet been examined, but could provide useful information for understanding the biology of the endocrine functions of the ovaries.
3 Consequences of Global miRNA Depletion in Ovarian Cells
Targeted gene deletion studies in mice suggest a critical role for miRNA regulation throughout the body, including the ovary . Initial studies showed that deletion of the Dicer eliminated almost all miRNA from the developing embryo and caused embryonic lethality at E6.5 due to a loss of pluripotent stem cells and impaired angiogenesis (Bernstein et al. 2003; Yang et al. 2005). Similarly, loss of Ago2 or Drosha was also embryonic lethal by E6.5–E7.5 (Kaneda et al. 2009; Yuan et al. 2014). To overcome these embryonic lethal phenotypes, Dicer conditional knockout (cKO) mouse models were developed and the essential roles of Dicer were demonstrated in a number of critically important tissues and cell types (Luense et al. 2009). In the reproductive organs, Dicer has been deleted using the oocyte-specific zona pellucida-3 (Zp3) promoter linked to Cre recombinase (Murchison et al. 2007), another oocyte-specific knockout using the DEAD (Asp-Glu-Ala-Asp) box polypeptide-4 (DDX4 or vasa) Cre and the anti-Mullerian hormone receptor-2 (Amhr2) Cre (Hong et al. 2008; Lei et al. 2010; Nagaraja et al. 2008; Gonzalez and Behringer 2009; Yuan et al. 2014), which is expressed in ovarian granulosa cells and derivatives of the Mullerian duct (oviduct, uterus, and cervix) and pituitary.
The targeted deletion of Dicer in the oocyte using Zp3-Cre resulted in infertility due to disorganized spindles, chromosome alignment defects, and oocyte arrest at metaphase-I of meiosis (Murchison et al. 2007; Tang et al. 2007). The Zp3-cKO of Ago2 resulted in similar chromosome abnormalities although these oocytes are able to mature to metaphase-II (Kaneda et al. 2009). Deleting Dicer at an even earlier stage of oocyte development (E15) caused female infertility due to failure of follicle growth (Yuan et al. 2014). Thus, Dicer, and Ago2 are necessary for normal oocyte meiosis, early follicle development and female fertility. This was originally interpreted to indicate that miRNA had minimal roles in oocyte development or in the crosstalk of the oocyte with the cumulus cells of growing follicles but that miRNA were critical in the oocyte at later stages for the completion of meiotic maturation prior to ovulation. However, more recent studies have discovered that Dicer and Ago2 are also involved in the biogenesis of endogenous small interfering RNA (siRNA) as well as miRNA (Flemr et al. 2013; Yuan et al. 2014). Two studies have helped to differentiate between the siRNA and miRNA pathways in the oocyte (Suh et al. 2010; Yuan et al. 2014). DGCR8 and Drosha are essential for miRNA but not siRNA biogenesis pathways. Therefore, DGCR8 was conditionally deleted from oocytes using the Zp3-Cre and the DDX4-cre, similar to the studies with Dicer and Ago2 (Suh et al. 2010; Yuan et al. 2014). Interestingly, deletion of DGCR8 or Drosha resulted in normal follicular development and oocytes that matured, fertilized and developed to healthy live born offspring indicating a requirement for siRNA but not miRNA in mouse oocyte maturation (Suh et al. 2010; Yuan et al. 2014). Another recent study specific to rats and mice discovered that the Dicer protein in the oocytes (DicerO) of these species is different from the Dicer expressed in their somatic cells (Flemr et al. 2013). Expression of the DicerO transcript is driven by an alternative promoter that originates from a retrotransposon. As a result, DicerO has a higher affinity for double stranded RNA that have perfect complementarity, thus DicerO generates siRNA instead of miRNA (Flemr et al. 2013). These findings explain the severe phenotypes seen in the oocyte-specific knock-outs of Dicer and Ago2, but that are not found in the cKO of DGCR8 and Drosha. Because this oocyte-specific isoform of Dicer is not present in humans or other mammals, the implications only affect studies performed in rodents. It is clear that the function of small RNAs is necessary for correct development and function of the oocyte, however, much remains to be discovered in this young field. The mouse may not be an adequate model for the study of miRNA in the oocyte, but it may be suitable for studies in somatic cells because these cells have the full length Dicer that is required for miRNA processing.
Targeted knockout of Dicer has also been conducted using the Amhr2-Cre which deletes Dicer from the mesenchyme of the developing Mullerian ducts and post-natally in ovarian granulosa cells and mesenchyme-derived cells of the female reproductive tract (Gonzalez and Behringer 2009; Hong et al. 2008; Nagaraja et al. 2008; Pastorelli et al. 2009). Female mice with Amhr2-cKO of Dicer suffer from complete infertility due to abnormalities in all of these tissues. Progesterone (P4) and estrogen levels tested normal in these mice (Hong et al. 2008). However uteri were short and immature. Oviduct development was severely affected, especially at the isthmus, which showed a lack of smooth muscle leading to bulbous distended sac-like structures and a general disorganization of the oviductal epithelial layer (Nagaraja et al. 2008; Hong et al. 2008; Pastorelli et al. 2009). While the primary cause of infertility in the Amhr2-cKO Dicer mice was due to the trapping of oocytes and embryos within the enlarged sacs of the oviduct, these mice also exhibited reduced ovary weight and lower ovulation rates demonstrating a need for Dicer in ovary and follicular development (Hong et al. 2008). In addition, the cKO of Dicer in follicular cells led to luteal insufficiency and failure to maintain pregnancy (Otsuka et al. 2008). Studies such as these alter miRNA in a global manner, and consequently disrupt the more than 2500 miRNAs that have been described (see http://microRNA .sanger.ac.uk). Further research is necessary in order to understand the effect of specific miRNA and their targets on endocrine regulation within the ovary.
4 Ovarian miRNA Expression Profiling
Another mechanism used by researchers to identify whether miRNA might be involved in ovarian function has been through the profiling of miRNA expression in ovarian tissues. Initial studies focused on miRNA gene expression in whole ovarian tissues comparing different ages of animal (i.e., fetal/newborn to adults) (Ahn et al. 2010; Ro et al. 2007; Mishima et al. 2008; Tripurani et al. 2010; Huang et al. 2011) and different physiological states (follicular versus luteal phase) (Hossain et al. 2009). Mouse and cow have been the models most commonly used to identify miRNA expression patterns in the ovaries of adult and fetal animals, but studies on, pigs, goats, and sheep have also been performed (Li et al. 2011; Ling et al. 2014; McBride et al. 2012). While these studies identified ovarian specific miRNA, the advent of more robust techniques such as small RNA sequencing and deeper analysis of more tissues, has left very few miRNA for consideration as ovarian specific. Another major drawback of these studies is that the ovary is comprised of a very heterogeneous population of different cell types and that even within a cell type, the function and therefore gene expression can vary wildly dependent on the stage of the estrous or menstrual cycle at which the cells are evaluated.
Several studies have used more defined populations of ovarian somatic cells or have compared the changes in miRNA expression in extracellular vesicles (i.e., exosomes and microvesicles) isolated from follicular fluid collected at discrete periods of follicle development. Briefly, comparisons of miRNA expression of granulosa cells of subordinate and dominant follicles during the early luteal phase of the bovine estrous cycle using RNAseq identified significant differential miRNA expression (Salilew-Wondim et al. 2014). In granulosa cells isolated from mice following a superovulation protocol, a small number of miRNA in granulosa cells were found to change temporally with respect to administration of an ovulatory dose of the LH mimic, human chorionic gonadotropin (hCG) (Fiedler et al. 2008). Within the highly steroidogenic corpus luteum, small RNAseq was used to identify 15 miRNA that were differentially expressed in pregnant versus non-pregnant cows and these miRNA were predicted to target genes involved in apoptosis and immune signaling pathways (Maalouf et al. 2014). Lastly, several studies have characterized the miRNA associated with extracellular vesicles derived from follicular fluid (Diez-Fraile et al. 2014; Roth et al. 2014; Sohel et al. 2013; Sang et al. 2013; da Silveira et al. 2012). In mares and women, age was shown to influence miRNA expression in extracellular vesicles (da Silveira et al. 2012; Diez-Fraile et al. 2014). Altered miRNA abundance was also seen in the follicular fluid of women with polycystic ovarian syndrome compared to normal controls (Roth et al. 2014; Sang et al. 2013). In another study, differences in miRNA abundance in extracellular vesicles compared to miRNA in vesicle-free fractions of follicular fluid was observed (Sohel et al. 2013). MicroRNA were found in both vesicle and vesicle-free fractions of bovine follicular fluid, and the miRNA showed differential abundance in these two fractions. While this finding is intriguing, the biological function of miRNA within the extracellular vesicles and extracellular vesicle-free miRNA remains to be determined. Furthermore, most studies assessing changes of miRNA abundance in follicular fluid lack experimental controls necessary to determine the parental cell of origin that generated the miRNA. Unfortunately, those studies of the corpus luteum showing differential miRNA expression still suffer from being derived from a very heterogeneous population of cell types (i.e., immune, endothelial, luteal). These studies highlight our current lack of understanding of miRNA expression within ovarian tissues and the need to perform more robust studies aimed at understanding the functional role these small molecules have on ovarian function.
In conclusion, the genetic depletion of critical regulators of miRNA biogenesis and function, and the evidence of differential expression of miRNA within tissues and cells collected at discrete functionally relevant time points, does support a role for these molecules on normal ovarian function.
5 MicroRNA Regulation Within the Antral Follicle
As a major functional unit within the ovary , follicles undergo a progressive transformational process as they grow from primordial to ovulatory follicles. During this developmental process the antral follicle becomes the first tissue to produce significant levels of the major endocrine hormone, estradiol (E2). This estrogen dominates during the follicular phase and is critical for regulation of the hypothalamic/pituitary/gonadal axis, secondary sex characteristics, reproductive tract function, and reproductive behavior. Copious numbers of studies have examined the pathway of E2 production and the role gonadotropins play in support of the endocrine functions of the antral follicle (Knobil and Neill 2014). Indeed, the well-known ‘two cell two-gonadotropin theory’ describes how compartmentalization of the steroidogenic enzymes and gonadotropin receptors within the two different follicular cells, facilitate thecal cell production of androgens and subsequent granulosa cell aromatization of those androgens to estrogen (Knobil and Neill 2014) (Fig. 6.2). Furthermore, estrogen has negative and positive endocrine effects at the pituitary that are essential for regulation of the LH and FSH secretion that drives folliculogenesis and ultimately the surge of LH that signals the end of follicular development and the ovulation of the oocyte. While a substantial number ~26 papers have examined the putative functions of miRNA in mural granulosa cells isolated from antral follicles, most of these have focused on other critical cellular elements involved in folliculogenesis such as proliferation and apoptosis (see Reviews McGinnis et al. 2015; Donadeu et al. 2012). A very limited number of studies have examined the role miRNA play specifically in the regulation of steroidogenesis within the antral follicle, those papers and supporting papers from other areas of biology are described below.
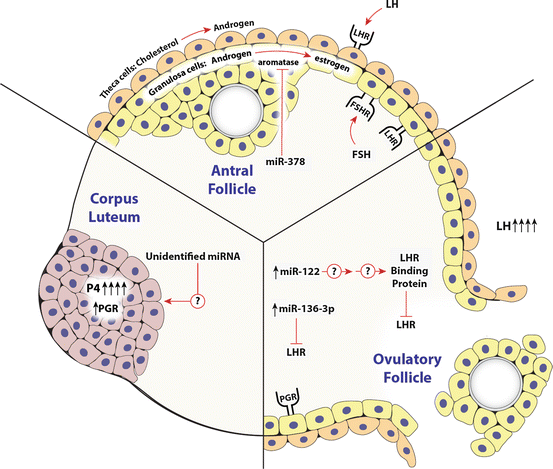
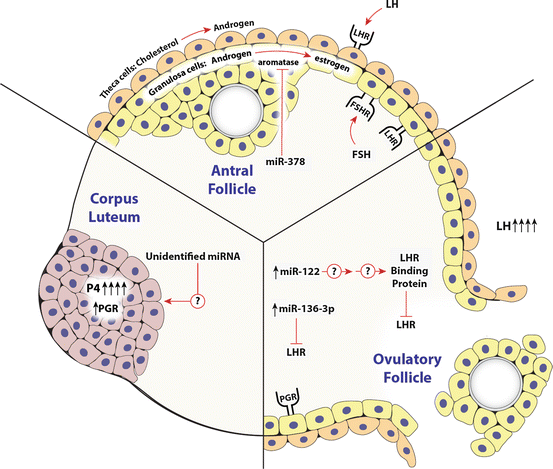
Fig. 6.2
MicroRNA regulation of ovarian endocrine system. Ovarian endocrine regulation is divided into three main temporal periods: the antral follicle, ovulation, and corpus luteum. The cell types involved (theca, granulosa and luteal), the primary endocrine hormones (FSH and LH) and their respective receptors (FSHr and LHr), and the primary steroids (progesterone, P4; androgens, A4; and estrogen, E2) produced by these cells are illustrated. Those miRNA that have been implicated in the direct regulation of these hormone secretion pathways or the regulation of the receptors are shown. Details including the references related to these findings are described in the appropriate sections within this chapter
Ovarian endocrine function is dependent on androgen signaling through interactions from the theca cells and granulosa cells (Hu et al. 2004). Androgens can affect ovarian steroidogenesis directly through androgen receptors (AR), or indirectly by the process of aromatization to estrogen. Androgens play a critical role in early follicle development, as demonstrated by mice that lack a functional AR. These mice are less fertile and have a shorter reproductive window than controls (Hu et al. 2004; Lyon and Glenister 1980). Androgen receptors are highly expressed in granulosa cells and theca cells of early stage follicles (Gervasio et al. 2014). AR can enhance the expression of miR-125b, which has anti-apoptotic effects and may regulate follicular atresia by promoting survival of granulosa cells (Sen et al. 2014). In primary mouse granulosa cells, AR binds to the promoter of the miR-125b primary transcript at three androgen response elements, in an androgen dependent fashion, indicating a transcriptional regulation of miR-125b. Interestingly, the transcriptional regulation of miR-125b was dependent upon nuclear and extranuclear actions of androgen, because the transcriptional upregulation of miR-125b was dependent on interactions of AR and paxillin, a scaffolding mediator of kinase cascades. The in vivo effect of miR-125b on ovarian function was demonstrated using bursal injections of miR-125b inhibitors, which significantly increased the expression of pro-apoptotic proteins BAK, BAX, BMF, and p53 in granulosa cells and induced apoptosis. This study demonstrated the in vivo effect of a miRNA on follicular development, in an androgen-dependent manner. MicroRNAs targeting the AR have also been identified systematically in prostate cancer cell lines (Ostling et al. 2011). A gain-of-function screen of 1129 miRNA in a panel of human prostate cancer cell lines was used to quantify changes in AR protein. Identification of 13 miRNA were validated using a luciferase reporter assay containing the AR 3′UTR : (miR-135b, miR-185, miR-297, miR-299-3p, miR-34a, miR-34c, miR-371-3p, miR-421, miR-449a, miR-449b, miR-634, miR-654-5p, and miR-9). Briefly, these assays work by linking the 3′-UTR of the gene of interest (i.e., AR, PGR) to the luciferase gene, followed by transfection of this reporter into cells where the investigator either blocks or enhances the expression of a specific miRNA. The contribution of these AR-modulating miRNA within the ovary remains to be determined.
During folliculogenesis and steroidogenesis, E2 acts together with FSH and is critical in enabling pituitary neuron cells to exhibit fluctuating endocrine patterns allowing for the preovulatory LH surge (Xu et al. 2011). Because of the importance of E2, the regulation of aromatase (CYP19A1) has been extensively studied (reviewed Stocco 2008). In porcine granulosa cells, miR-378 down regulates the expression of CYP19A1 (and therefore estrogen) (Xu et al. 2011). MicroRNA-378 targeted both CYP19A1 and progesterone receptor (PGR), and the down regulation of this miRNA appeared to be necessary for granulosa cell differentiation to the preovulatory stage. These studies were both performed in vitro, and it remains unclear how the miRNA is regulated in vivo.
Several additional miRNA can target genes involved in E2 production by mouse granulosa cells. MicroRNA-224 can affect E2 production by targeting Smad4, a transcription factor involved in the transforming growth factor-beta1 (TGF-β1) pathway that can activate expression of a variety of proliferation genes (Yao et al. 2010a). These studies, however, are difficult to interpret because TGF-β did not induce miR-224 expression consistently. The same group identified miR-383 as being down regulated by TGF-β1. MicroRNA-383 can target RNA binding motif, single stranded interacting protein 1 (RBMS1), thus increasing E2 release (Yin et al. 2012). Another miRNA associated with E2 synthesis is miR-133b, which directly targets the transcription factor Forkhead L2 (Foxl2), which inhibits transcription of steroidogenic acute regulatory protein (StAR) and CYP19A1, thus leading to an increase in E2 production after FSH stimulation (Dai et al. 2013). Both isoforms of the estrogen receptor (ERα and ERβ) are important in fertility, as demonstrated in KO mouse models. Null ERα (αERKO) mice are infertile, while null ERβ (βERKO) mice are subfertile (Couse et al. 2003). The αERKO mice contained hemorrhagic and cystic ovaries, and displayed abnormally high levels of serum LH, which resulted in elevation of steroidogenic genes (Cyp17a1, Cyp19, and HSD17b3). ERβ is expressed in granulosa cells, and the βERKO mice had a predominant phenotype in follicles, with evidence of unruptured follicles after superovulation as well as decreased numbers of recovered oocytes (Krege et al. 1998). While the two estrogen receptors are essential to fertility, the contribution of miRNA to their regulation is poorly understood in the ovary . Several studies have identified miRNA that target ERα and ERβ in cancer cell lines. In hepatocellular carcinoma cell lines ERα was shown to be directly targeted by miR-18a and miR-22 (Liu et al. 2009), while in breast cancer cell lines miR-206 was shown to affect ER directly (Pandey and Picard 2009; Adams et al. 2007). Fewer studies have analyzed ERβ as a target of miRNA, limited to miR-92 in MCF-7 breast cancer cells (Al-Nakhle et al. 2010).
Additionally, endocrine hormones such as FSH that affect antral follicle development and function can also alter the expression of miRNA (Yao et al. 2010b). Specifically, 12 h post-FSH treatment in primary rat granulosa cells, miR-29a and miR-30d were significantly down regulated, but increased in expression after 48 h. However, the specific targets of these miRNA were not identified, and the biologic consequences of the biphasic response at 12 and 48 h were not explored. Importantly, many of the identified miRNA involved in steroid production pathways have been identified through in vitro experiments. Few studies have confirmed miRNA effects on steroidogenesis in vivo.
6 MicroRNA Regulation Within the Ovulatory Follicle
At the completion of follicular growth, increasing follicular estrogen signals to the hypothalamus and pituitary to initiate a surge of LH secretion that facilitates the release of the oocyte and the conversion or luteinization of the granulosa and thecal cells. During this periovulatory period, P4 becomes highly concentrated in follicular fluid (Emori and Drapkin 2014), and it is critical for the final stages of folliculogenesis and for ovulation (Duffy et al. 2010). Simultaneously, the LH surge induces the transient expression of progesterone receptor (PGR) in the luteinizing granulosa cells of rodents, and in mammals with a true luteal phase, the LH surge induces long-term expression of PGR (Gougeon 2010; Hatzirodos et al. 2014; Iwai et al. 1990; Revelli et al. 1996). In mice the function of the two different isoforms of PGR (A and B) have been evaluated and loss of PGR-A was shown to cause a 70 % decline in the number of oocytes ovulated while in contrast, loss of PGR-B had no effect detectable on ovulation (Conneely et al. 2003; Shao et al. 2003). The transcriptional expression of PGR is clearly upregulated in granulosa cells following hormonal gonadotropin signaling through FSH and LH (Shimada and Terada 2002; Clemens et al. 1998), but post-transcriptional regulation by miRNA has also been shown to be involved (Toms et al. 2014). In the pig, PGR was inversely correlated with expression of miR-378-3p in developing follicles and in vitro studies using isolated granulosa cells showed that miR-378-3p could directly target PGR mRNA as evidenced by 3′-UTR luciferase reporter assays (Toms et al. 2014). The expression of miR-378-3p is lower in porcine large follicles, suggesting that by repressing this miRNA during late folliculogenesis, the repression of genes essential for granulosa cell differentiation (CYP19A1 and PGR) is alleviated. Additionally, in three breast cancer cell lines, miR-513a-5p decreased the luciferase activity in a PGR luciferase reporter assay, indicating that this miRNA also directly targets PGR (Cochrane et al. 2012). Supporting miR-513a-5p as a direct regulator of PGR, this miRNA was also induced by P4 (Cochrane et al. 2012), whether this miRNA is expressed in the ovary and if it may function there has not been assessed.
In another approach to examine whether miRNA can elicit changes in steroidogenesis in vitro, primary granulosa cells were harvested from normally cycling women after ovariectomy due to non-metastatic cervical cancer and were transfected with 80 different pre-miRNA constructs (Sirotkin et al. 2009). Markers of proliferation, apoptosis, and secretory activity of P4 were assessed to identify miRNA involved in ovarian function (Sirotkin et al. 2010). Pre-miR-15a treatment enhanced the expression of proliferative and apoptotic signals, and promoted the release of P4 and testosterone, but not E2 (Sirotkin et al. 2014).
In vivo experiments performed in mice identified several miRNA in granulosa cells including miR-21, miR-132 and miR-212 that changed significantly following the LH surge (Fiedler et al. 2008). Inhibition of miR-21 by injection of inhibitors beneath the bursa of mice blocked ovulation (Carletti et al. 2010). Conversely, none of these three miRNA have shown effects on ovarian steroidogenesis or luteinization in vitro or in vivo (Carletti et al. 2010; Fiedler et al. 2008; McGinnis et al. 2015). Lastly, the LH receptor (LHr) itself has been shown to be under regulation by miRNA. Studies performed in whole ovary digestion experiments in rats identified miR-122 as positively correlated with an increase of LHr mRNA binding protein (LRBP ) after treatment with hCG, through an indirect and unknown mechanism (Menon et al. 2013). The authors postulate that this mechanism may be through the regulation of sterol regulatory element binding proteins-1 and -2 and the temporal patterns of expression does support this claim, yet direct evidence for this remains to be shown. Another study performed using whole rat ovary homogenates identified miRNA-136-3p as being overexpressed 6 h after hCG treatment and bioinformatic analyses indicated that 3′-UTR of the rat LHR has a miR-136-3p site within it. The biologic activity of miR-136 was then tested in primary rat granulosa cell culture and was shown to directly target the rat LHr (Kitahara et al. 2013). It should be noted that bioinformatic (TargetScan) analysis of the human LHr 3′-UTR does not detect a miR-136-3p site within it. This does not preclude the possibility that a miR-136-3p site is present, but considering that the 3′-UTR of genes have the greatest sequence variability, this would need to be tested empirically. It is thus critical that we do not generalize results across species and that until confirmatory results are produced in vivo the functional roles of miRNA can at best be speculative.
7 MicroRNA Regulation Within the Corpus Luteum
The granulosa and thecal cells following exposure to the LH surge, differentiate (i.e., luteinize) to form the corpus luteum (CL). This luteinization process involves the rapid hypertrophy of the granulosa and thecal steroidogenic cells and rapid proliferation of endothelial cells that allows invasion of capillaries around the previous avascular granulosa cells. These profound cellular and tissue remodeling events enable this newly formed tissue to produce exceptionally high P4 levels that are critical for normal cycles and for establishment and maintenance of pregnancy. To date, the female infertility observed in a mouse Dicer hypomorph provides the best evidence that miRNA play a role in luteal function (Otsuka et al. 2008).
The Dicer hypomorphic female mice were infertile due to CL insufficiency resulting, in part, from impaired growth of new capillaries in the ovary (Otsuka et al. 2008). This phenotype was partially due to the lack of miR-17-5p and let7b, two miRNA that function in angiogenesis by down regulating anti-angiogenic tissue inhibitor of metalloproteinase (TIMP1). The angiogenesis defect was rescued by re-administering the miRNA-17-5p and let7b, however full-term pregnancy was not maintained indicating that the miRNA are not the only factors controlling these complex processes. Recently, global expression analysis of miRNA from CL of sheep, pigs and cows have provided additional evidence for miRNA involvement in luteal function.
In sheep, miRNA involved in angiogenesis and cell survival pathways were highlighted in the transition from pre-ovulatory follicle to CL (McBride et al. 2012). In this study, cells were isolated from pre-ovulatory follicles, early CL, late CL and corpus albicans and subjected to RNAseq PCR then confirmed by Northern blot analysis and qRT-PCR (McBride et al. 2012). Overall, the most abundantly expressed miRNA included miR-21, miR-125b, let-7a and let-7b contributing 40 % of all miRNA in the ovarian tissues examined. Some miRNA were expressed at higher levels in the follicles (miR-199a-3p, miR-125b, miR-145 and miR-31) while others were much higher in the CL (miR-503, miR-21 and miR-142-3p) (McBride et al. 2012). Potential gene targets were determined from miRTarBase and it was found that the majority of these miRNA were involved in the regulation of cell cycle, survival, differentiation and angiogenesis. Increases in these pathways makes sense since the CL consists of multiple cell types that must differentiate, multiply and survive long-term in the case of pregnancy.
Global expression analysis of miRNA from CL of pregnant (day 17) versus non pregnant cows identified 15 miRNA that were differentially expressed (Maalouf et al. 2014). Interestingly, similar to studies in sheep (McBride et al. 2012) miR-21, let-7, and miR-199 were among the highest miRNA expressed in the cow ovaries and confirmed an earlier report from the cow (Hossain et al. 2009). Pathway analysis implied miRNA are especially involved in the regulation of cell survival and apoptosis in the bovine CL, a similar theme to that identified in the sheep (McBride et al. 2012). In the pig, miR-378 expression increased during CL development and decreased during luteolysis indicating a possible function in CL formation and maintenance (Ma et al. 2011). Since miR-378 promotes cell survival and angiogenesis in other cell types (Lee et al. 2007) these known functions support the proposal that miR-378 may play similar roles in the CL (Ma et al. 2011). However, in a more recent study miR-378-3p was found to be inhibitory to PGR expression in pig granulosa cells (Toms et al. 2015). Since the PGR activity is critical to maintenance of the CL (Maybin and Duncan 2004), further studies are needed to understand these contradictory, possibly species-specific roles for miR-378.
These few studies indicate essential roles for miRNAs in luteal function. However, more studies are needed to identify and fully define these pathways. There is also a critical need for in vivo based studies to determine the true effects of miRNA on the physiology of cells and tissues.
8 MicroRNA in Reproductive Diseases
With the importance of miRNA in ovarian physiology described above, differences in miRNA expression might be expected in ovarian diseases. Surprisingly, few studies of miRNA function have been conducted on human patients with ovarian diseases and of those few studies that have been reported, only one disease polycystic ovary syndrome (PCOS) is specifically related to endocrine regulation within the ovary. As one of the most common endocrine-metabolic disorders in women of reproductive age (Imbar and Eisenberg 2014; Norman et al. 2007), PCOS results in menstrual disorders and infertility. Since miRNA were first discovered in follicular fluid (da Silveira et al. 2012), additional studies have compared miRNA contents between PCOS and healthy women (Roth et al. 2014; Sang et al. 2013). Combined genome-wide deep sequencing and TaqMan miRNA arrays then followed-up with cell culture experiments were used to identify miRNA involved in follicle steroidogenesis in PCOS patients (Sang et al. 2013). From their microarray results, they selected miRNA that were postulated to function in steroidogenesis in other tissues. MicroRNA-mimics or inhibitors were generated and transfected into a steroidogenic human granulosa-like tumor cell line (KGN) and cultured to determine the intracellular effects of these miRNA. The results identified five miRNA that regulated E2 in KGN cells: miR-24 decreased E2 secretion while miR-132, miR-320, miR-520c-3p and miR-222 all increased E2 secretion. Three miRNA were found to regulate P4 secretion (miR-24, miR-193b and miR-483-5p) (Sang et al. 2013). Follow-up testing of follicular fluid from PCOS and non-PCOS patients confirmed that there were decreased levels of miR-132 and miR-320 in the PCOS patients (Sang et al. 2013). In spite of the decline in miR-132 and miR-320 and that these two miRNA caused an increase in E2 secretion from KGN cells in vitro, the levels of E2 and P4 were not altered in the follicular fluid of PCOS patients. Therefore, the in vivo effects of these miRNA could not be confirmed in vitro and further studies are needed to determine if these miRNA serve a critical function within the follicle.
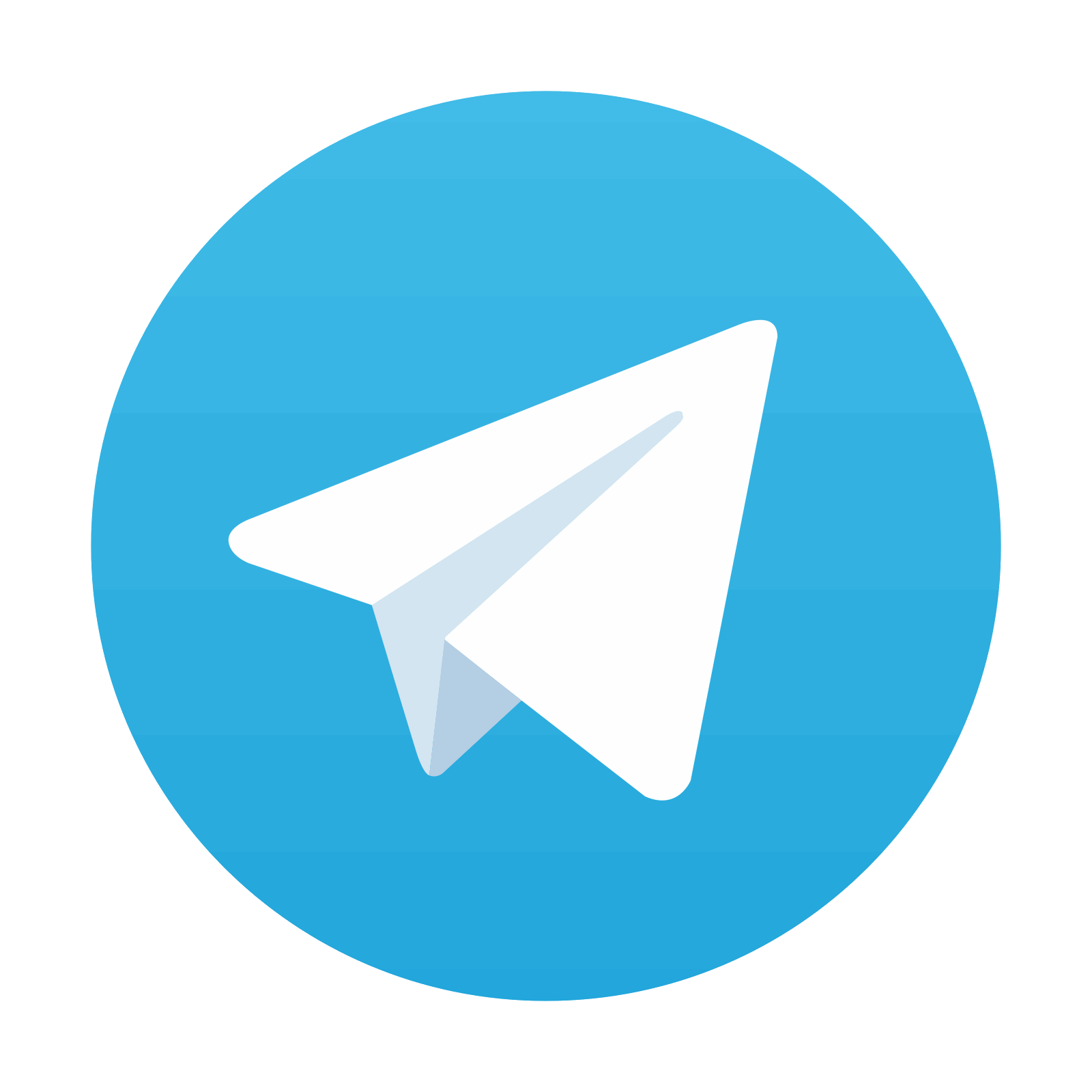
Stay updated, free articles. Join our Telegram channel

Full access? Get Clinical Tree
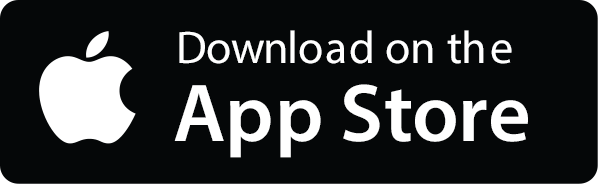
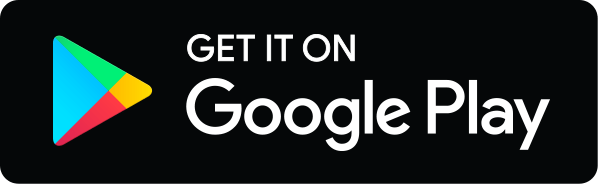