Fig. 6.1
A brief history of microRNA research milestone
The function of miRNAs requires a sequence specific match to their target mRNA. The majority of match pairs are composed of 7–8 nt nucleotides in the “seed” regions (5′-end) of the miRNAs that are perfectly complementary to 3′-UTR segments of the target mRNAs (Bartel 2009). The mechanistic model of “seed” pairing leads to the possibility that miRNAs are influencing the expression or evolution of nearly all mammalian mRNAs (Farh et al. 2005). It is well established that miRNAs are broadly involved in cancer cell proliferation, tumorigenesis, metastasis, angiogenesis and drug resistance. Based on the influence on cancer cell growth, they can be categorized as oncogenic or tumor-suppressive miRNAs. Oncogenic miRNAs (oncomirs) induce cancer cell proliferation by down-regulating expression of tumor suppressor genes, whereas tumor suppressor miRNAs (mirsupps) inhibit cancer progression by targeting oncogenes post-transcriptionally (Li and Yang 2013a). However, this dichotomous approach is challenged by growing evidence. A particular miRNA could be increased in some cancers as an oncomir, but downregulated in other cancers. For example, miR-17 was found as a mirsupps in breast cancer (Hossain et al. 2006). while it promotes development of hepatocellular carcinoma (Shan et al. 2013). Moreover, as a single miRNA is able to target a host of mRNAs, studying miRNA’s function is complicated by enormous genetic diversity observed in cancers. Hence, miRNAs and their related network more likely have a buffering effect in cellular hemostasis.
6.2 MicroRNA and Metabolic Stress in Cancer
The growth of cancer requires increased supply of nutrition and oxygen, which permits rapid expansion of a tumor. To adapt to the accelerated metabolism rate, cancer cells develop unique genetic alternations that dysregulate the control of cell proliferation. Perhaps one of the significant adaptations is Warburg effect, which is named after Dr. Otto Warburg. His discovery that cancer cells harbor a highly glycolytic rate which increases glucose consumption and lactate production regardless of the concentrations of oxygen, giving rise to a new era where detection and treatment of cancer could be focused on its unique metabolic signature (Warburg 1956). Thus some researchers suggest that cancer as a whole is a metabolic disease.
6.2.1 MicroRNA and Oxidative Stress
As a result of Warburg effect and anaerobic respiration, several potential toxic compounds are generated. They include reactive oxygen species (ROS), reactive nitrogen species (RNS), reactive sulfur species (RSS) and reactive chloride species (RCS) (Sosa et al. 2013). Of them, ROS are produced most abundantly. These reactive species can cause damage to DNA structure and its repair mechanism. They can also liberate lipid peroxidation and increase permeability of cell membrane. Elevated concentration of ROS has been frequently found in cancer cells. Oxidative stress affects several biochemical pathways, such as PTEN/PI3K/Akt, and MAPK/ERK. Notably, miRNAs also actively respond to intracellular change of ROS. It was firstly identified that ROS accumulation in small cell lung cancer (SCLC) cells was linked with miR-17-92 (Ebi et al. 2009). Overexpression of miR-17-92 cluster counterbalances ROS generation in SCLC cells. It was suggested that miR-17-92 plays a role in fine-tuning the effects of ROS-induced DNA damage, maintaining genomic stability (Ebi et al. 2009). Several ROS-related miRNAs have been described thereafter (Sosa et al. 2013). MiR-200 family—comprising miR-141, miR-200a, miR-200b, miR-200c and miR-429—has been shown as key regulators of oxidative stress (Batista et al. 2013) (Fig. 6.2). These miRNAs control cellular motility by mediating epithelio-mesenchymal transition (EMT), and they also influence cellular stemness and apoptosis by targeting p38α MAPK. High expression of miR-200 s is often found in epithelial ovarian cancer (EOC) and correlated with a better outcome and/or early-stage disease (Marchini et al. 2011). Based on the level of miR-200, EOC can be stratified as “oxidative stress” and “fibrosis” signature (Batista et al. 2013) “Stress” patients have a better response to chemotherapy and longer survival, compared to “fibrosis” patients. There was an enhanced expression of miR-141 and miR-200a in ovarian cancer cells exposed to oxidative stress, leading to down-regulation of p38α and increased ROS production. The up-regulation of ROS levels, in turn, augments expression of miR-200 family, which together sensitizes tumor cells to cisplatin or carboplatin treatment (Mateescu et al. 2011). This study implicates that the signature of miR-200s can be used as predictive biomarker for chemotherapy response. Restoration of miR-200s levels may be a new therapeutic approach in drug resistant EOC patients.
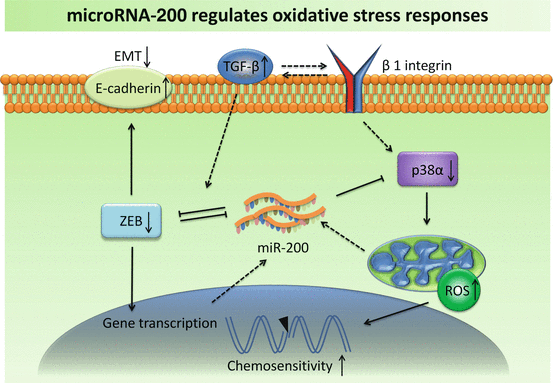
Fig. 6.2
Increased expression of miR-200 suppresses ROS-inhibitor p38α and EMT-inducer ZEB, which in turn regulates miR-200 in a feedback loop
Increased levels of glycolysis and anaerobic respiration prevent tumor cells from entering senescence and stimulate vascularization. Accumulating studies have demonstrated the role of miRNAs in regulating cellular response to hypoxia. Most of these hypoxia-responsive miRNAs are found to be associated with hypoxia-inducible factor 1 (HIF-1) signaling pathway. MiR-210 is a robust target of HIF-1, and its overexpression has been linked to adverse prognosis in breast cancer and hepatocellular carcinomas (Devlin et al. 2011). It is indicated that miR-210 activates the generation of ROS, by targeting ISCU (iron-sulfur cluster scaffold homolog) and COX10 (cytochrome c oxidase assembly protein), two key factors of the mitochondrial electron transport chain. It thus inhibits mitochondrial function and up-regulates the levels of glycolysis (Chen et al. 2010). ISCU, which is also a target of HIF-1, is a cofactor for enzymes involved in the TCA cycle and iron metabolism. Through interfering with HIF-1 at multiple levels, miR-210 enhances cancer cell survival in hypoxic condition, but also makes cells more sensitive to glycolysis inhibitor (Ying et al. 2011).
6.2.2 MicroRNA and Starvation
It is well known that tumor cells are characterized by high nutritional requirements underlying a constitutively ‘hungry’ phenotype. In response to nutrient starvation, varied changes will occur on the genetic and epigenetic levels favoring cell survival. It was firstly found that miRNA was involved in starvation-induced alternations in human hepatocarcinoma cells (Bhattacharyya et al. 2006). When cells are growing under unstressed conditions, miR-122 binds to the 3′-UTR of cationic amino acid transporter 1 (CAT-1) mRNA. Nevertheless, such pairing was reversed in cells subject to starvation, by relocalization of CAT-1 mRNA from cytoplasmic processing bodies (PBs). As scaffolding center of miRNA function, the activity of PBs showed an on- and off- switch in a context-dependent manner. Under stressed conditions such as amino acid deprivation, the 3′-UTR of CAT-1 binds to HuR, an ARE binding protein, relieving CAT-1 from miR-122 suppression in PBs and recruiting it to polysomes for translation (Bhattacharyya et al. 2006). This model suggests a way that RNA-binding protein modulates the activity of miRNAs in tumor cells under stressed conditions. Through promoter region-directed modulation, miRNA activation is closely related to the intracellular environment. It is found that glucose depletion-induced oxidative stress inhibits histone deacetylation in miR-466h-5p promoter region, which actives miR-466h-5p, miR-669c and Sfmbt2 in a time-dependent manner (Druz et al. 2012). The authors suggested that miR-297-669 cluster, including miR-466h-5p, might play a role in cellular detoxification and drug-induced injuries. During oncogenic transformation, induction of miR-297-669 cluster is inhibited with the loss of oxidative stress defense mechanism (Druz et al. 2012).
Glioblastoma cells are characterized by an aggressive growth pattern and frequent cellular apoptosis, making it an optimal model to test the nutrition-dependent functions of microRNAs. In cultured U87 cells deprived of serum, the levels of miR-17 increased remarkably (Fig. 6.3). By targeting PTEN and stabilizing HIF-1 alpha, miR-17 reduced cellular metabolic rates under unfavorable conditions in order to protect them from starvation. Notably, miR-17 also inhibited tumor cell proliferation under unstressed conditions through targeting MDM2, an oncogene often over-expressed in cancer cells. Thus, miR-17-overexpressing cells became more resistant to chemotherapy, since most cytotoxic reagents act by diminishing highly proliferative cells (Li and Yang 2012). This finding, in which miR-17 plays a dual role in glioblastoma cells, provides a new perspective to our understanding of stress responses in cancer. MiR-93, a paralog of miR-17-92, was found increased in human breast carcinoma. By modulating large tumor suppressor homology 2 (LATS2), miR-93 enhances tumor angiogenesis and metastasis in a mouse lung metastasis model (Fang et al. 2012). It also promotes tumorigenesis and angiogenesis in human brain tumor by targeting integrin beta-8 (Fang et al. 2011). These findings suggest a promising role of miRNA as a predictor in “tumor-starving” therapy. Since tumors harboring highly expression of these miRNAs often show an excessively angiogenesis pattern, elucidation of the underlying cross-reaction between miRNAs and anti-angiogenic treatment is likely uncover new opportunities for therapeutic intervention.
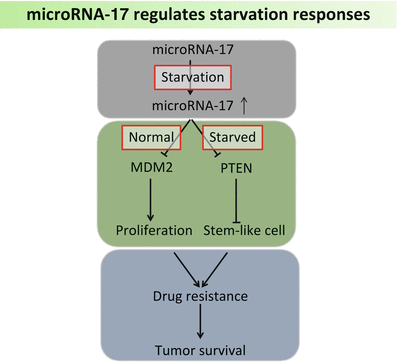
Fig. 6.3
MicroRNA-17 expression increases under starved conditions, which further facilitates tumor survival by targeting MDM2 and PTEN
In order to survive under conditions of high nutrient demands (‘hungry state’), cancer cells heavily rely on aggressive angiogenesis to permit ample blood supply and oxygen uptake. Therefore, “tumor-starving” (anti-angiogenic) therapy has been employed to prevent tumor vascularization and deprive it from nutrition. Initially, it was found that miR-378 contributes to tumor angiogenesis in transplanted glioblastoma, by targeting SuFu and Fus-1. As a result, miR-378 promotes tumor cell survival and growth (Lee et al. 2007). Furthermore, miRNA-induced angiogenesis is a common phenomenon observed in different types of tumor (Li and Yang 2013b).
6.2.3 MicroRNA and Autophagy
Autophagy is a catabolic process which transports cellular components to lysosomes for self-degradation. It is a cytoprotective mechanism in maintaining hemostasis and highly conserved during evolution. Deregulation of autophagy has been implicated in a variety of cancers (Xu et al. 2012). Due to elevated metabolic demands, aggressive tumor cells often display robustly activated autophagy in order to fuel mitochondrial metabolism. Autophagy may also limit ROS toxicity by triggering mitophagy, wherein damaged mitochondria are eliminated (Mathew and White 2011). Beclin 1 (also known as autophagy-related gene 6 or Atg 6) is a key autophagy-promoting player in development and progression of cancer, including breast cancer, ovarian cancer, glioblastoma and lymphoma (Huang et al. 2010). It was firstly demonstrated that Beclin 1 is a potential target of miR-30a. Inhibition of beclin 1 expression by miR-30a blunted the activation of autophagy induced by rapamycin in tumor cells (Zhu et al. 2009). The miR-30 cluster contains five paralogs: miR-30a, b, c, d, e. The potential targets of miR-30 subfamily also include B-Myb, a transcription factor that positively regulates cell proliferation and cell cycle. By binding to the 3′-UTR of B-Myb, miR-30 is able to repress endogenous expression of B-Myb and inhibit cellular senescence in Hela cells (Martinez et al. 2011). Further investigation suggests that miR-30 is a prominent tumor suppressor in prostate cancer, breast cancer and glioblastoma (Kao et al. 2014). Through regulation of EMT-associated oncogenes, miR-30 in prostate cancer cells suppresses EMT features and inhibits tumor cell migration and invasion (Kao et al. 2014). Remarkably, miR-30 is also broadly involved in tumor cell apoptosis and stem-like cells generation (Yu et al. 2010). Taken together, these data suggest that miR-30 represents a bridge between apoptosis and autophagy (Xu et al. 2012).
Another well-studied miRNA is the miR-17-92 cluster. The human miR-17-92 cluster locates at 13q31.3, a fragile region often amplified in hematopoietic malignancies. The function of this cluster was firstly reported in B-cell lymphoma, where enforced expression of miR-17-92 accelerated tumor development by acting with c-myc (He et al. 2005). Cumulative evidence has demonstrated a pivotal role of miR-17-92 in cancer. As a potential oncomir, miR-17-92 was found abundantly expressed in immature hematopoietic cells. Sequestosome 1 (SQSTM1), an ubiquitin-binding protein associated with autophagy, was found inhibited by miR-17-92 cluster in myeloid progenitors (Meenhuis et al. 2011). SQSTM1 plays an important role in inclusion body formation by binding to the autophagic regulator Atg8/LC3 (Komatsu et al. 2007). In tumor cells under stress, accumulation of SQSTM1 caused persistent damage to mitochondria and cellular genome. It was indicated this failure to eliminate SQSTM1 was sufficient to alter NF-kB pathway and contribute to tumorigenesis (Mathew et al. 2009). By interfering with SQSTM1-regulated pathways, miR-17-92 actively modulates stress responses in tumor cells.
In addition, miR-155 also was found to play a role in autophagy by regulating multiple molecules including RHEB, RICTOR, and RPS6KB2 in the mTOR signaling pathway (Wan et al. 2014). Increased expression of mIR-155 increases autophagic activity in human nasopharyngeal cancer and cervical cancer cells. Silencing endogenous mIR-155 inhibits hypoxia-induced autophagy. These results release a new role for mIR-155 as a key regulator of autophagy via dysregulation of MTOR pathway.
On the other hand, components involved in the miRNA biogenesis pathway are closely linked with autophagy process (Fullgrabe et al. 2014). As a functional center of miRNA-RISC complex, DICER1 and AGO2 can be integrated in the autophagosome after binding to the selective autophagy receptor NDP52 and GEMIN4 (Fullgrabe et al. 2014). It is eventually leading to protein degradation in the autophagosome-dependent lysosome. Therefore, autophagy is involved in maintaining miRNA biogenesis by removing inactive DICER1-AGO2 complex, preventing them competing for additional factors which are required for miRNA maturation (Gibbings et al. 2012). In turn, miRNAs control the activity of core autophagy proteins. In chronic lymphocytic leukemia cells, miR-130a inhibits autophagosome generation by targeting DICER1 and ATG2B (Kovaleva et al. 2012). It was indicated that miR-130a and DICER1 form a regulatory feedback loop that mediates tumor cell survival (Kovaleva et al. 2012).
6.3 MicroRNA and Tumor Microenvironment
Tumor microenvironment comprises blood vessels, immune cells, fibroblasts and extracellular matrix. Numerous signaling molecules and pathways are influencing the interactions between the tumor and its surrounding microenvironment. It is believed that such interplay is remolding tumor microenvironment, which allows for tumor angiogenesis and metastasis. Meanwhile, immune responses are often suppressed in the host, leading to tumor-tolerogenic macrophages, NK/T cells and neutrophils. Any fluctuation in microenvironment could impact global signaling of tumor cells, and thus influence the stress response through miRNA-regulated pathways.
6.3.1 MicroRNA and Immune Response
The puzzling question how tumor cells escape from natural immune surveillance has stimulated extensive research into tumor mediated immune suppression. It is becoming increasingly clear that immune response dysregulation plays a critical role in cancer progression and therapeutic resistance. Hence normalizing of the microenvironment can improve anticancer outcome. Analysis of tumor infiltrating lymphocytes has demonstrated that many types of tumors show evidence of T-cell infiltration (Gajewski et al. 2013). Of particular, activated CD8+ T cell responses have been associated with a positive prognosis in tumors such as colorectal cancer (Mlecnik et al. 2011). More studies are underway to explore the prognostic value of cancer associated immune biomarkers. Recent findings have suggested that miRNAs are greatly involved in modulating the proliferation, differentiation and response of CD8+ T cells. Initial characterization of miRNA profile in CD8+ T cells provided insight into the understanding of miRNA’s role in a cell-specific setting (Fig. 6.4). Comparing naïve, effector, and memory CD8+ T cells, it was shown that 7 miRNAs (miR-16, miR-21, miR-142-3p, miR-142-5p, miR-150, miR-15b and let-7f) are most frequently expressed in all the T cell subsets, whereas they tend to be down-regulated in effector T cells and come back up in memory T cells (Wu et al. 2007). During the process of differentiation, some miRNAs such as miR-21 and miR-155 are found up-regulated while the miR-17-92 cluster is concomitantly decreased (Salaun et al. 2011). T cell tolerance to cancer cells characterizes immune suppression in the tumor microenvironment. Rescuing tolerant T cells by lymphopenia-mediated homeostasis-driven proliferation may enable development of new immunotherapeutic strategies. By analyzing genome-wide miRNA profile in tolerant T cells, Greenberg et al. found that miR-21 and miR-184 up-regulated after rescuing, whereas miR-181a was decreased (Schietinger et al. 2012). Further studies revealed that miR-181a expression inversely correlated with mRNA levels of 56 predicted target genes. The authors pointed out that miR-181a could be a possible key negative regulator of functions in CD8+ T cells (Schietinger et al. 2012). By inhibiting innate immune response, miR-181 may enhance tumor vascular invasion and metastasis. Over-expression of miR-181 was found correlated with poor survival in oral squamous cell carcinoma, suggesting it as a potential biomarker for cancer tolerance and prognosis (Yang et al. 2011b). The understanding of miRNA’s potent effects in tumor-mediated immunosuppression was driven by studies in tumor-bearing mice. Increased expression of miR-15b was observed in isolated CD8+ T lymphocytes in mice with Lewis lung carcinoma (Zhong et al. 2013). Ectopic expression of miR-15b in CD8+ T cells inhibits apoptosis by knocking down death effector domain-containing DNA-binding protein (DEDD). DEDD is a ubiquitous death effector domain containing protein which induces apoptosis through its N-terminal DED motif. High expression of miR-15b is also associated with inactivation of CD8+ T lymphocytes by repressing the production of cytokines such as IL-2 and IFN-γ (Zhong et al. 2013). Despite of its anti-apoptotic effect, miR-15b likely plays a negative role in the activation of effector T cells and anti-tumor immune response. Dynamic change of tumor-associated miRNA expression can be also observed in miR-17-92 cluster. In patients with multiple myeloma, the miR-92a level in CD8+ T cells was significantly down-regulated compared with normal subjects (Yoshizawa et al. 2012). With the remission of disease, the plasma miR-92a levels became normalized. Together, these findings suggest gain or loss of miRNA functions may represent the T-cell immunity status in tumor host.
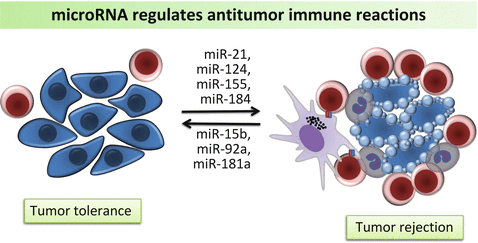
Fig. 6.4
MicroRNAs are involved in regulation of tumor tolerance and antitumor immune reaction by mediating CD8+ T cells, NK cells and macrophages
Accumulating evidence has identified signal transducer and activator of transcription 3 (STAT3) as a critical molecule in regulating tumor-associated immunosuppression by interfering with multiple factors. Constitutive expression of STAT3 alters gene-expression programs, inhibits expression of immune mediators and suppresses leukocyte infiltration into the tumor (Yu et al. 2007). Blocking STAT3 in immune cells can generate diverse anti-tumor immunity by suppressing negative regulators such as immature dendritic cells and regulatory T cells and activating CD8+ T cells, natural killer cells and neutrophils (Yu et al. 2007). Thus, STAT3 has emerged as a potential target for tumor immunotherapy. Recent studies have demonstrated that the interplay between miRNAs and STAT3 broadly exists in cancer development and progression. MiR-124 has been reported as a potential tumor suppressor in diverse tumor types, such as colorectal cancer and prostate cancer (Cao et al. 2013). In patients with glioblastoma, miR-124 expression is significantly reduced, compared to normal brain tissues (Wei et al. 2013). Ectopic upregulation of miR-124 in glioma stem-like cells promoted T cell proliferation and regulatory T cell induction. Moreover, treatment of T cells from glioblastoma patients with miR-124 induced pro-inflammatory cytokines and chemokines (Wei et al. 2013). As a result, systemic administration of miR-124 prolonged overall survival and decreased tumor incidence in a murine glioma model. Such anti-tumor effects were proved to depend on the presence of T cells. In tumor bearing mice depleted of CD4+ or CD8+ cells, the immunotherapeutic effects of miR-124 was ablated (Wei et al. 2013). Activation of STAT3, in turn, can modulate expression of several miRNAs. For example, there is a highly conserved STAT3-binding site in the promoter of the miR-17-92 gene (C13orf25) (Brock et al. 2009). By modulating the expression of IL-6, activation of STAT3 upregulates the entire miR-17-92 cluster. Interestingly, there are two seed regions of miR-17-92 in STAT3 3′-UTR, and thereby miR-17-92 reversely targets STAT3 expression, leading to reduced ROS generation (Zhang et al. 2011). By modulating STAT3 associated immune tolerance in myeloid-derived suppressor cells (MDSCs), the negative regulatory loop between miR-17-92 and STAT3 may be an important factor in tumor associated immune response and a potential immunotherapeutic target against cancer.
6.3.2 MicroRNA and Epithelial Mesenchymal Transition
Epithelial mesenchymal transition (EMT) is regarded as a key process of tumor invasion and distant metastasis. It is essential for cancer cells facilitating survival in a hostile milieu and escape from adverse sites (Taddei et al. 2013). As a major stress-adaptive strategy, EMT leads epithelial cells to lose their cell polarity and cell-cell adhesion, and gain morphological and functional characteristics of mesenchymal cells (Taddei et al. 2013). A prominent marker of EMT is the loss of epithelial cadherin (E-cadherin) expression. E-cadherin is a transmembrane glycoprotein that mediates intercellular adhesion via hemophilic binding (Sheng et al. 2006). Inactivation of E-cadherin has been found in most carcinomas (Paredes et al. 2012). Recently, miRNAs are emerging as potential regulators of E-cadherin. MiR-200 family is the first miRNAs identified to be associated with E-cadherin expression (Hurteau et al. 2007). In breast cancer cells with less invasive phenotype, there is endogenous expression of both miR-200c and E-cadherin. However, in estrogen receptor negative cells, miR-200c as well as E-cadherin levels are merely detectable. By targeting E-cadherin repressors ZEB1 and ZEB2, miR-200c was able to restore E-cadherin function and therefore inhibit EMT (Hurteau et al. 2007). Most recent study revealed that miR-200/ZEB interaction is crucial to breast cancer growth and metastasis (Truong et al. 2014). This network is subject to the regulation of β1 integrin and transforming growth factor-β (TGF-β). In triple negative breast cancer cells, knockdown of β1 integrin changed cell migration pattern and stimulated distant metastasis by activating TGF-β. Reducing the abundance of TGF-β or restoring the ZEB/miR-200 balance reestablished cell cohesion and reduced tumor dissemination (Truong et al. 2014). Augment evidence suggests miR-200 as a potential marker of metastasis capacity. MiR-200/ZEB network is not only involved EMT in breast cancer, but also a key regulator of prostate cancer and gastric cancer (Williams et al. 2013). Imbalance of miR-200/ZEB is associated to invasive subtype of gastric cancer and poor prognosis of patients (Song et al. 2014). Notably, miR-200 family also exerts their effects on cellular plasticity and metastasis by modulating additional signaling in parallel to ZEB. Actin-associated gene moesin was inversely associated with miR-200 expression (Li et al. 2014). In a similar pattern as miR-200/E-cadherin interaction, miR-200/moesin axis regulates breast cancer cell metastasis in a context-dependent manner (Li et al. 2014).
6.4 MicroRNA Regulation of Stress Responses to External Stimuli in Cancer
When a patient is first diagnosed with cancer, the common medical practice is to perform surgical resection of the tumour, in an attempt to liberate the patient from the cancerous mass of cells. Unfortunately, tumour resection is not always an optimal procedure for cancer on its own, and despite the surgeon’s best efforts, cancer cells will still be present in the patient’s body, and will continue to grow if left untreated. In an attempt to combat these remaining cells, chemotherapy or radiotherapy is often used adjunctively. Each of these procedures induces a molecular stress response, as the patient’s remaining tumour cells attempt to survive the poisons and high-energy radiation that they are being bombarded with. The cells are faced with two options—adapt or perish. These responses are usually the manifestation of complex molecular signaling cascades, which are attempting to maintain cellular homeostasis despite the increasingly harsh environment. These signaling cascades are fine-tuned through constant monitoring and regulation of the genes, transcripts and proteins involved. As research elucidates the participants in these complex networks, microRNAs are emerging as key players in the regulation of stress responses to chemotherapy and radiotherapy highlighting a potential for the exploitation of these oligonucleotides for therapeutic use. There are thousands of microRNAs, each regulating the levels of hundreds to thousands of proteins, and this review aims at illuminating the nature of microRNAs through select examples, thus elucidating possible therapeutic opportunities.
6.4.1 MicroRNA and Chemotherapy
Chemotherapy is a cancer treatment generally involving the use of one or more cytotoxic drugs with the aim of slowing and ideally stopping the growth of tumours. Chemotherapeutics generally act by targeting rapidly dividing cells (which cancer cells are) and preventing cell division through a variety of mechanisms including impairing the cell division machinery and damaging DNA—often leading to programmed cell death, known as apoptosis.
Chemotherapy can offer an excellent adjuvant treatment for killing cancer cells. However, this treatment often becomes less effective, as cancer cells acquire traits helping them to survive the toxicity. Resistance to chemotherapy is believed to cause treatment failure in over 90 % of patients with metastatic cancer (Longley and Johnston 2005). Resistance to the stressors of chemotherapy can occur through many different mechanisms, which are poorly understood. However, it is becoming increasingly apparent that microRNAs can serve a regulatory role in the molecular mechanisms underlying drug resistance and thus may hold the potential to be used to reverse chemoresistance.
6.4.1.1 Regulation of Multidrug Resistant Proteins
The ATP-binding cassette (ABC) transporter is a superfamily of transmembrane proteins transporting compounds across the cellular membrane against the concentration gradient through the use of ATP-coupling. Nine members of the “C” subfamily of ABC transporters (entitled multidrug resistance proteins, or MRPs) have been found mediate chemotherapeutic drug resistance through efflux of the drug out of the cells (Chen and Tiwari 2011). By pumping the chemotherapeutic out of the cell via MRPs, cancer cells are able to minimize exposure to the toxic drugs.
Several studies have implicated microRNAs in the regulation of MRP levels, and thus more broadly implicate microRNAs in chemoresistance. For example, miR-27a was found to directly target and have the net effect of down-regulating the MRP p-glycoprotein expression and reversing chemoresistance in leukemic cells. Interestingly, a separate study found that miR-27a could activate p-glycoprotein indirectly (through the down-regulation of an upstream target) and contribute to chemoresistance in ovarian cancer cells. This seemingly contradictory data exemplifies the convoluted and often environment-specific effects of microRNA, of which a complete understanding is still in its infancy.
6.4.1.2 Regulation of Drug Metabolization
As chemotherapy drugs course through patients’ vasculature, they are slowly metabolized by the liver, where they are rendered inactive and then eventually expelled from the body. The cytochrome P450 (CYP) superfamily is a group of enzymes that catalyze the oxidation of organic molecules, and account for approximately 75 % of drug metabolism (Guengerich 2008).
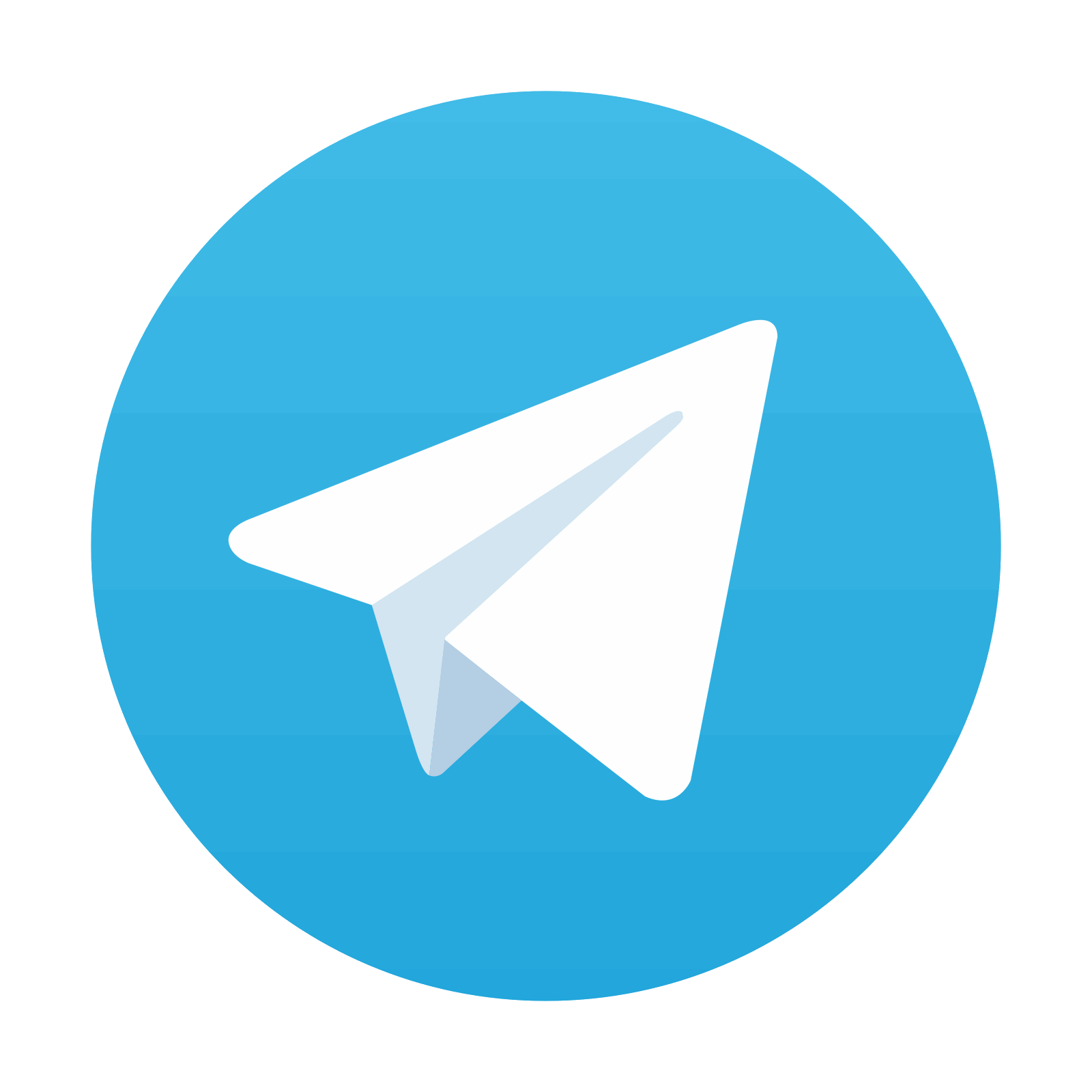
Stay updated, free articles. Join our Telegram channel

Full access? Get Clinical Tree
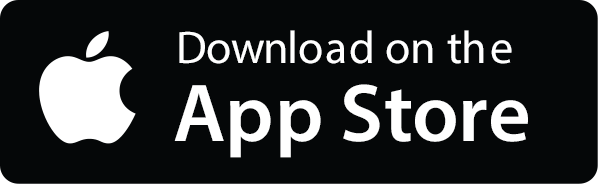
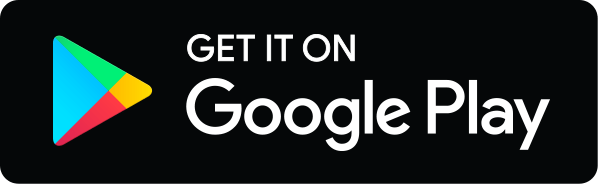