Keywords
MGMT, P140K, chemoprotection, drug-resistance gene therapy, hematopoietic stem cell gene therapy, glioblastoma
Introduction to MGMT Gene Transfer in Cancer Therapeutics
The O 6 -methylguanine methyltransferase (MGMT) catalytic protein confers resistance to alkylating agents, including chemotherapeutics such as temozolomide (TMZ), dacarbazine (DTIC), N,N′ -bis(2-chloroethyl)- N -nitroso-urea (BCNU), and BCNU derivatives N -(2-chloroethyl)- N′ -cyclohexyl- N -nitrosourea (CCNU) and N′ -[(4-amino-2-methylpyrimidin-5-yl)methyl]- N -(2-chloroethyl)- N -nitrosourea (ACNU). For cancer patients treated with these chemotherapeutics, off-target hematopoietic toxicity resulting in myelosuppression often causes delays in treatment with or without dose reduction to allow for continued administration, or possibly even treatment discontinuation, reducing the efficacy of cancer treatment. Thus, gene transfer of the MGMT transgene to hematopoietic stem cells (HSCs) and subsequent transplantation of the gene-modified HSCs would result in MGMT expression, and subsequent resistance from chemotherapy, in the HSCs as well as in all blood cell progeny arising from such cells mitigating dose-limiting hematopoietic cell toxicity to alkylating agent chemotherapy.
Numerous mutants of MGMT maintain DNA repair activity, removing alkylation adducts from the O 6 position of guanine, but unlike wild-type MGMT, they are resistant to inhibition by the nucleoside O 6 -benzylgunaine ( O 6 -BG). These mutant MGMT transgenes can be delivered to HSCs and provide myeloprotection in the context of a combined alkylating agent and O 6 -BG treatment regimen used to increase tumor cell sensitivity. The two mutants that have been most extensively studied are MGMT with a single amino acid glycine 156-to-alanine conversion (G156A) and a single amino acid proline 140-to-lysine conversion (P140K) . Of these, P140K has been extensively evaluated in preclinical large animal models and recently in phase I clinical studies .
In cancer therapy, the primary goal of MGMT gene transfer to HSCs is to provide hematopoietic protection from alkylating agent chemotherapy, but an additional feature of this system is the ability to increase the level of gene-modified cells in vivo . In the discussion of preclinical and clinical studies of MGMT gene therapy, protection of the hematopoietic compartment from an otherwise toxic dose of chemotherapy will be referred to as “chemoprotection” and is indicated by maintenance of normal or near normal complete blood cell counts, including absolute neutrophil count (ANC) and platelets. Increasing the percentage of P140K gene-modified cells in vivo will be referred to as “chemoselection” and will be indicated by the overall stable increase in gene-modified cells detectable in the peripheral blood and bone marrow. As the presented preclinical studies will demonstrate, these features of the MGMT gene therapy strategy are not mutually exclusive. For most applications, chemoprotection or chemoselection is the primary indicator of successful treatment, but the combined benefit is advantageous regardless of the application.
In either case, because P140K-modified cells have a transient selective advantage during alkylating chemotherapy treatment over nonmodified cells, any increase in the observed level of P140K-containing cells must be evaluated for safety to ensure that multiple gene-modified HSCs are contributing to peripheral blood cell production (polyclonal selection). In clinical studies of gene transfer, patients developed leukemic transformation as a result of insertional mutagenesis, and survival advantage of the gene-modified cells in vivo was a contributing factor to the oligoclonal expansion of leukemic cells. In retrovirus-mediated P140K gene transfer, clonal analysis has been accomplished by retrovirus integration site analysis (RIS) using either linear amplification-mediated (LAM) polymerase chain reaction (PCR) or modified genomic sequencing PCR coupled with high-throughput pyrosequencing or superconductor sequencing. The patterns of clonal distribution before and after chemotherapy in both large animals and patients are discussed as the primary analysis of safety of this gene therapy approach.
This chapter outlines how P140K gene therapy in HSCs has been tested in both autologous and allogeneic HSC transplant settings in preclinical large animal models, including dogs and nonhuman primates, as well as in a clinical trial for cancer patients. In addition, this chapter highlights the many different viral vectors used for P140K gene delivery as well as the kinetics of myelosuppression and selective advantage of P140K + cells. Lastly, this chapter discusses other disease models to which P140K gene therapy is applicable based on the results of these studies.
Preclinical Studies in Large Animal Models
MGMT gene therapy in HSCs has benefited greatly from the use of outbred large animal models, which better represent the genetic diversity and scale associated with clinical applications compared to small animal models such as the non-obese diabetic/severe combined immunodeficiency (NOD/SCID) xenotransplantation model. Large animal models display more clinically relevant drug metabolism kinetics and allow for long-term safety assessments, which are crucial for virus-mediated gene delivery given the latency of frank leukemia in gene therapy clinical trials for X-linked SCID, chronic granulomatous disease, and Wiskott–Aldrich syndrome . Although both canine and nonhuman primate models allow for long-term follow-up, each model has several advantages and disadvantages.
The canine model has an extensive history of expanding our knowledge of bone marrow transplantation , and canines require relatively simple care and handling. However, poor cross-reactivity with human growth factors and low similarity to the human genome are limitations of the canine model. The closer evolutionary relationship between nonhuman primates and humans makes safety evaluations concerning retrovirus integration more informative due to much more advanced genomic information in this animal model, and further benefit is derived from cross-reactivity of nonhuman primate HSCs with human recombinant growth factors used in transplantation. However, the care and handling of nonhuman primates is more difficult than that of canines.
To date, delivery of the P140K transgene into large animal HSCs has been accomplished with use of murine stem cell virus (MSCV)-based gammaretrovirus vectors, murine leukemia virus (MND or MND-MFG)-based gammaretrovirus vectors, human immunodeficiency virus 1 (HIV-1)-derived lentivirus vectors, simian immunodeficiency virus (SIV), or a foamy virus vector, all of which are discussed later. Both canine and nonhuman primate studies of P140K gene transfer into HSCs have provided promising efficacy and safety results, indicating both chemoprotection and chemoselection, but have also revealed differences in the kinetics of these two parameters, with the nonhuman primate model proving to be better correlated with kinetics observed in early human studies. In all cases, the initial level of gene transfer, the cell dose infused, conditioning regimen prior to cell infusion, and chemotherapeutic regimen administered after transplant appear to be the most important parameters in determining chemoprotective and chemoselective capacity.
Canine Preclinical Studies
The first published preclinical study of P140K gene therapy in a large animal model was conducted in the dog model of bone marrow transplantation in 2003 . To date, the most extensive analyses have been performed in this model . As discussed in the subsequent section detailing nonhuman primate studies, initial investigation was conducted in the dog primarily due to logistical considerations in transduction efficiency with currently available gene transfer vectors, as well as care and handling.
In the seminal study, two dogs (G069 and G154) were initially infused with CD34-enriched bone marrow cells from a dog leukocyte antigen (DLA)-matched littermate following high-dose total body irradiation (TBI) at a dose of 920 cGy . Infused cells consisted of CD34-enriched, recombinant canine granulocyte colony-stimulating factor (cG-CSF) and recombinant canine stem cell factor (cSCF) primed bone marrow cells following transduction with a P140K- and GFP-expressing gammaretrovirus, as well as nontransduced, non-CD34-enriched cells to provide a cell dose that typically would be expected to result in full donor chimerism in an irradiated recipient. In both dogs, hematopoietic recovery was rapid, with neutrophil recovery occurring by days 11 and 14, as evidenced by an ANC≥500/µl, and platelet recovery occurring by days 72 and 62, as evidenced by a platelet count≥20,000/µl without transfusions for platelet support. Initial chimerism analysis in these dogs at 3 months following transplant indicated that donor cell engraftment was between 77 and 80% in dog G069 and between 53 and 57% in dog G154. Flow cytometry analysis of peripheral blood white blood cells in these animals indicated that 12.9% of granulocytes were GFP + in dog G069 and 10.0% of granulocytes were GFP + in dog G154 at this same time point, demonstrating that P140K-transduced HSCs were able to engraft in this animal model following high-dose irradiation.
Next, engraftment of P140K-transduced cells was tested following reduced-intensity conditioning (400 cGy TBI). Due to concerns that the extended culture period needed for efficient transduction of cells by gammaretroviruses, which require cell cycling, would result in loss of engraftment potential, the P140K transgene was delivered instead by a lentivirus vector capable of efficient transduction in a shorter, overnight transduction protocol . Two additional dogs (G250 and G258) were infused with DLA-matched littermate cells as described for animals G069 and G154 and displayed rapid engraftment indicated by recovery of ANC and platelet counts, with initial donor chimerism in both dogs exceeding 75%. Given this high initial donor chimerism, a subsequent study (discussed later) was undertaken to further evaluate reduced-intensity TBI regimens in the allogeneic canine preclinical model.
Following the demonstration that both P140K-lentivirus- and P140K-gammaretrovirus-transduced CD34 + cells could engraft in canines, subsequent administration of O 6 -BG and BCNU chemotherapy was attempted to evaluate chemoprotection and chemoselection in three of the four animals (G069, G154, and G250) ( Figure 28.1 ). In all three dogs, increases in the level of circulating GFP + peripheral blood cells were observed after each O 6 -BG and BCNU treatment. Extrahematopoietic toxicity was limited, affecting only dog G069, and was confined to elevation in liver enzymes and cholestasis parameters prior to chemotherapy and without apparent correlation to drug treatments. Furthermore, LAM-PCR analysis of gene-modified cells indicated that multiple clones persisted after chemoselection, and bone marrow analysis showed normal, trilineage maturation and blast counts.
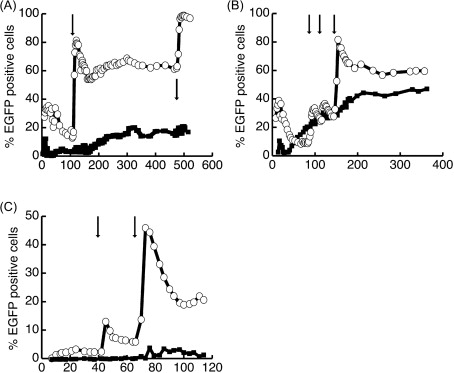
As noted previously, given the high initial donor chimerism observed in the animals receiving 400 cGy TBI in this study, a subsequent study was performed to further evaluate reduced-intensity TBI for allogeneic transplantation of P140K-modified HSCs . In this study, two dogs received reduced-intensity TBI 200 cGy (G340 and G403) prior to infusion with P140K-expressing, lentivirus-transduced CD34 + cells from a DLA-matched littermate donor. Both dogs received chemotherapy including O 6 -BG and TMZ (G340 and G403) early after transplant in an attempt to stabilize engraftment of gene-modified cells; however, robust chemoselection was not observed in either of these animals. Following these initial chemotherapy cycles, chimerism was allowed to stabilize, and both dogs transplanted following 200 cGy TBI (G340 and G403) and a dog previously transplanted following 400 cGy TBI (G258) were enrolled in a separate study to evaluate the ability to chemoselect long-term repopulating HSCs with O 6 -BG and BCNU between 210 and 589 days after transplantation.
Administration of O 6 -BG and BCNU resulted in significant chemoselection in all three dogs. Following one to four cycles of O 6 -BG and BCNU chemotherapy, levels of gene-marked granulocytes stably increased from 2 to 75% in dog G258, total white blood cells increased from 30 to 60% in dog G340, and total white blood cells increased from 2 to 5% in dog G403. These data demonstrated that gene marking could be increased after nonmyeloablative transplantation, which could be clinically applied to patients—especially elderly patients—with relapsed hematopoietic malignancy after transplantation. In addition, the severity of myelosuppression associated with these chemotherapy treatments progressively declined as gene marking increased in all three animals in this study, confirming an inverse relationship between the level of P140K-expressing cells and chemotherapy-induced myelosuppression.
Extending these studies to address safety concerns pertaining to insertional mutagenesis in gene-modified HSCs with an intrinsic selective advantage, arising from development of frank leukemia in X-SCID patients treated with gene therapy , a subsequent study evaluating the retrovirus integration profile before and after chemotherapy, providing a transient selective advantage, as well as engraftment and repopulation potential of chemoselected P140K + HSCs, was reported . In this report, long-term follow-up (median 4.8 years) of 11 dogs transplanted with P140K-modified HSCs was provided that demonstrated that increased gene marking was stable and consistently resulted in multilineage hematopoiesis. In-depth analysis of retrovirus integration sites by LAM-PCRin some of the animals revealed no global chemoselection for gene-modified clones with provirus integration events near transcription start sites of proto-oncogenes.
Most convincing of the repopulation potential of chemoselected P140K-expressing HSCs, this report described DLA-matched secondary transplants resulting in sustained gene marking in the secondary recipients with multiple gene-modified clones identified in peripheral blood of both primary and secondary recipients ( Figure 28.2 ). Subsequent treatment of one of the secondary recipients with O 6 -BG and BCNU resulted in chemoprotection and chemoselection of gene-modified cells. These data together supported that chemotherapy treatment and chemoselection of P140K-expressing clones did not compromise multilineage repopulating potential or result in selection of malignant clones.
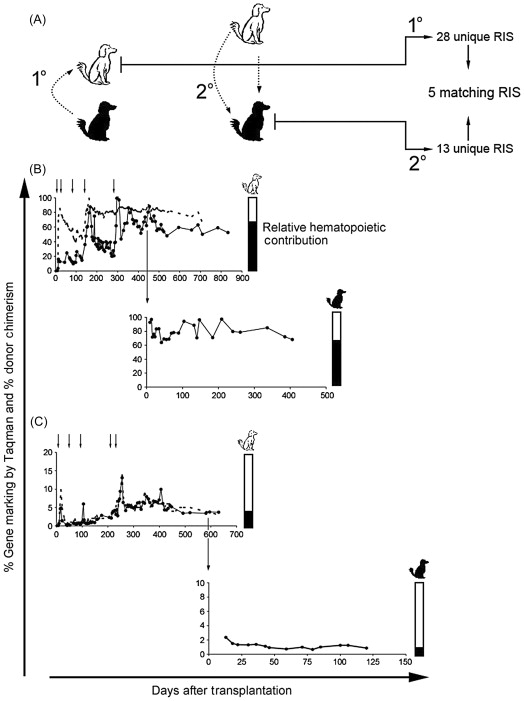
These initial allogeneic studies in the dog confirmed that P140K gene-modified HSCs were capable of engrafting and mediating both chemoprotection and chemoselection in the clinically relevant dog model of bone marrow transplantation safely and efficiently. This application would be suitable for hematopoietic malignancies where the source of gene-modified cells would be a donor (allogeneic). However, to establish that this model could apply to solid tumor cancer patient populations, wherein autologous HSCs could be used, additional studies were performed in the dog. The most appropriate patient population in which to develop this platform was the poor prognosis glioblastoma multiforme (GBM) population in which tumor cells displayed an unmethylated MGMT promoter indicative of high endogenous MGMT expression and, thus, poor response to standard chemotherapy treatment including TMZ (see Chapter 30 ). To specifically target the MGMT + GBM population as a potential clinical population for early clinical trials, these studies also examined the ability to chemoprotect and chemoselect P140K-modified cells in the context of O 6 -BG and TMZ chemotherapy because TMZ is the standard-of-care for GBM patients but also used extensively for patients diagnosed with malignant melanoma and acute leukemia.
Two dogs, G179 and G197, were transplanted with autologous CD34 + cells following transduction with a P140K-expressing gammaretrovirus after conditioning with 920 cGy TBI. Following hematopoietic recovery and determination of stable engraftment after transplant, animal G179 received four cycles of O 6 -BG and 50 mg/m 2 TMZ every day for 3 days, which resulted in a rise in gene marking levels in all peripheral blood cell lineages examined. This dog then received an additional four cycles of O 6 -BG and TMZ chemotherapy with escalated doses of TMZ from 300 to 600 mg/m 2 in single bolus doses, again demonstrating increases in gene-modified cell levels following each cycle. Given the sustained chemoprotection and chemoselection observed in this animal nearly 2 years after transplant, a ninth chemotherapy cycle at a TMZ dose of 700 mg/m 2 was administered with minimal hematopoietic toxicity, requiring no transfusion support and observation of only transient transaminitis, which did not occur after administration of the 600 mg/m 2 TMZ cycle. These data suggested that autologous, P140K gene-modified bone marrow-derived CD34 + cells engraft and afford significant chemoprotection and chemoselection, potentially allowing for dose intensification of TMZ and improved efficacy of chemotherapy in the treatment of cancer.
These findings were then extended to the second animal, G197, which also received a total of nine cycles of combination O 6 -BG and TMZ, again with observed chemoprotection and chemoselection following each cycle, with a maximum TMZ dose of 800 mg/m 2 . Again, minimal hematopoietic toxicity was observed, this time with no extrahematopoietic toxicity. Because no information was available concerning TMZ metabolism in dogs, the authors evaluated toxicity associated with TMZ doses of 500 and 600 mg/m 2 in two control dogs (E977 and G047). Toxicity in these two dogs was pronounced and included severe neutropenia and thrombocytopenia, in stark contrast to platelet levels observed in animals that received P140K-expressing cells.
The previous studies demonstrated promising results for autologous transplantation using P140K-expressing cells, but ablative transplantation with high-dose TBI is not appropriate for most clinical applications for patients diagnosed with various solid tumors. Ideally, a conditioning regimen for these applications would (1) be toxic to the recipient’s HSCs, (2) have limited toxicity outside the hematopoietic system, and (3) have a documented anti-glioma effect. In a recent report, dogs received autologous P140K-expressing cells following reduced-intensity conditioning with the combination of BCNU and TMZ . In this study, four dogs were treated with BCNU (30–50 mg/m 2 ) followed by administration of TMZ (550–850 mg/m 2 ) 2 hr later. All dogs recovered from chemotherapy conditioning and displayed detectable, albeit low, levels of gene marking. Subsequent treatment of these dogs with O 6 -BG and TMZ at TMZ doses ranging from 600 to 700 mg/m 2 resulted in gene-marking-dependent chemoprotection and chemoselection. However, one animal (G593) exhibited a spontaneous loss in gene-modified cells 100 days after transplant, corresponding to discontinuation of cyclosporine administration. To evaluate whether loss of gene-modified cells was related to human P140K expression, a competitive repopulation assay in a fifth dog was carried out, in which half of the transplanted CD34 + cells were transduced with a promoterless P140K-containing lentivirus vector (no transgene expression) and the other half of the cells were transduced with the same lentivirus backbone driving P140K expression from the human elongation factor 1α (EF1α) promoter. In this animal, both gene-modified populations were detectable at equivalent levels in peripheral blood cells up to 123 days after transplant; however, following discontinuation of cyclosporine, a complete loss of cells containing the expressed human P140K transgene was observed, whereas cells containing the promoterless (no transgene expression) human P140K provirus stabilized at approximately 1% of peripheral blood white blood cells. Whether this observation resulted from an immune response to the human P140K transgene being expressed in canine cells, inefficient engraftment or differentiation of P140K-expressing long-term repopulating HSCs, or cytotoxicity induced by high MGMT expression is unclear .
In both allogeneic and autologous studies, clonal composition analysis by LAM-PCR found clones with multipotential repopulating capacity both before and after chemotherapy in all dogs evaluated, suggesting that chemoselection occurs at the HSC level and that chemoprotected HSCs that are active early after transplant were able to survive multiple rounds of chemotherapy. These pioneering studies provided supportive data indicating that this strategy would be a clinically translatable approach, specifically in the MGMT + GBM patient population in which O 6 -BG and TMZ chemotherapy had been attempted, but was found to result in considerable myelotoxicity in the absence of P140K-modified cells . The initial clinical trial based on these data is discussed later.
Nonhuman Primate Preclinical Studies
The first published study of P140K gene-modified hematopoietic cell engraftment and repopulation in the nonhuman primate model was conducted in the pigtailed macaque ( Macaca nemestrina ) . Prior to this report, poor transduction efficiency with HIV-1-derived lentivirus vectors, thought to be the result of potent viral capsid uncoating by the restriction factor tripartite motif-containing protein 5α (TRIM5α), had hampered preclinical use of Old World monkeys for lentivirus-mediated gene therapy . This seminal study included an in vitro demonstration that pigtailed macaque CD34 + cells were permissive to HIV-1-derived lentivirus transduction similar to levels seen in human cell and unlike poor transduction observed in rhesus macaque ( Macaca mulatta ) and baboon ( Papio cynocephalus ) CD34 + cells. For in vivo studies in the pigtailed macaque, autologous CD34 + cells isolated from the bone marrow of monkeys treated with recombinant human (rh)G-CSF and SCF were transduced with either a P140K- and GFP-expressing (J02370 and T04228) or P140K- and C46-expressing (J02043) self-inactivating lentivirus vector. C46 is a membrane-bound peptide that can inhibit fusion of HIV viral particles , rendering C46-expressing cells resistant to HIV infection, an application that is discussed later. During transduction, cells were cultured in media containing recombinant human growth factors including G-CSF, SCF, TPO, flt3-L, interleukin-3 (IL-3), and IL-6. For conditioning prior to transplantation, all animals received myeloablative TBI ranging from 800 to 1020 cGy. The total cell dose received by all three monkeys ranged from 4.6 to 11.7×10 6 cells/kg of body weight. Following transplant, all three animals recovered neutrophil counts to levels above 500/µl in less than 11 days, platelet counts to greater than 20,000/µl within 25–35 days, and displayed GFP + or provirus + cells (in the case of J02043, which received cells that did not express GFP) in all peripheral blood cell subsets analyzed. Although these animals did not receive chemotherapy until a later study (described later), all displayed stable, long-term, high-level gene marking of hematopoietic cells gene-modified with an HIV-1-derived lentivirus vector expressing P140K in peripheral blood cells. This study demonstrated the preclinical utility of the pigtailed macaque as a model to study HIV-1-derived lentivirus-mediated gene transfer, and it provided additional evidence that P140K-expressing HSCs could engraft in a myeloablated recipient, differentiate to form all blood cell progeny, and maintain provirus transgene expression in vivo long term.
Two years following these initial reports, a subsequent study of the chemoprotective and chemoselective efficacy of transplanted autologous P140K gene-modified HSCs was published by a separate group utilizing rhesus macaques ( M. mulatta ) . In this study, an SIV-based lentivirus vector was used because rhesus CD34 + cells were previously established to be resistant to HIV-1-dervied lentivirus vector transduction. A total of five rhesus macaques were treated with rhG-CSF and rhSCF, or with AMD310 alone, for HSC mobilization. CD34 + cells were then enriched from either mobilized apheresis products or aspirated bone marrow and transduced with SIV-based lentivirus vectors encoding either P140K and GFP or YFP or tricistronic vectors encoding P140K, GFP, and the homeobox protein 4 (HOXB4) gene, which was thought to promote expansion of short-term repopulating hematopoietic cells. Following myeloablative total body irradiation consisting of two fractionated doses of 450–500 cGy, animals were infused with gene-modified cells at doses ranging from 2.1 to 8.5×10 6 cells/kg body weight. Following transplant, all animals recovered neutrophil counts to a level exceeding 500/µl within 7–17 days (average, 10.8 days), similar to the recovery observed in pigtailed macaques.
To test the chemoprotective and chemoselective capacity of P140K-expressing hematopoietic cells, all monkeys were given combination chemotherapy O 6 -BG and TMZ within 3–6 months after transplant. O 6 -BG was administered as a bolus intravenous infusion of 120 mg/m 2 over 20 min. TMZ was administered orally 1 hr after the completion of the O 6 -BG infusion and ranged from 170 to 450 mg/m 2 . All animals received between one and seven cycles of combination O 6 -BG and TMZ chemotherapy spaced 1 or 2 months apart depending on hematopoietic toxicity and recovery between cycles. In this study, only transient chemoprotection and chemoselection were observed following each cycle of O 6 -BG and TMZ chemotherapy in all five animals, and one animal died from radiation-induced toxicity following a single cycle of chemotherapy. Three of the remaining four animals were treated with subsequent chemotherapy including O 6 -BG and BCNU (one, two, or three cycles), with BCNU administered as an intravenous infusion of 20 mg/m 2 over a 5-min period beginning 30 min after the completion of the O 6 -BG infusion. Again, only transient chemoprotection and chemoselection were observed in all animals treated. In addition, pronounced extrahematopoietic toxicity following BCNU treatment, including pulmonary toxicity in the two animals receiving two and three cycles of BCNU chemotherapy and gastrointestinal (GI) toxicity in the remaining animal receiving a single cycle of BCNU chemotherapy, was observed and ultimately resulted in the death of these three animals. Based on these findings, it was postulated that treatment with O 6 -BG in combination with either BCNU or TMZ was selective of hematopoietic progenitor cells, resulting in transient chemoprotection and chemoselection, rather than long-term repopulating hematopoietic cells. These data contrasted with trends in chemoselection and chemoprotection that were observed in the canine studies discussed previously, suggesting a species-specific response difference. However, other important differences between these studies and those conducted in dogs included the chemotherapy regimen administered, vector design, and the level of gene marking prior to initiation of chemotherapy . Specifically, the rhesus study used a 5-day fractionated dose of O 6 -BG and TMZ, as opposed to the single bolus O 6 -BG and TMZ dose that resulted in efficient in vivo chemoselection and chemoprotection in dogs. In addition, the lentivirus vector used in these studies may have resulted in more robust transduction of hematopoietic progenitors or overall less efficient transduction compared to the vector used in canine studies, both of which could compromise effective gene transfer to true hematopoietic stem cells. Lastly, the levels of gene-modified cells in all animals treated in this study were less than 10% of peripheral blood cells and less than 2% in most of the animals at the time chemotherapy was initiated, and few multipotential clones were identified.
In contrast to the observations in canines transplanted with P140K-expressing HSCs, this study suggested that stable chemoselection and adequate chemoprotection may not be achievable in nonhuman primates and, potentially, in human patients. However, in a subsequent study published the following year that included long-term follow-up of the pigtailed macaques ( M. nemestrina ) and baboons ( P. cynocephalus ) transplanted in the initial reports by Beard et al. and Trobridge et al ., as well as transplant of additional pigtailed macaques, stable chemoselection and chemoprotection was observed following transplantation with P140K gene-modified hematopoietic CD34 + cells .
To assess in vivo efficacy of P140K gene-modified HSCs in these animals, administration of O 6 -BG and TMZ was initiated using an identical strategy to that which provided efficient chemoprotection and selection in the canine studies discussed previously . Of the two baboons reported in this study, one initially received three cycles of O 6 -BG and TMZ, whereas the other received a single cycle of this chemotherapy combination, all at a TMZ dose of 400 mg/m 2 . In these initial treatments, TMZ was administered following a single bolus O 6 -BG infusion. An O 6 -BG dose of 120 mg/m 2 was administered as a bolus infusion over 15–20 min. The level of GFP + (and thus P140K + ) cells in the peripheral blood of these animals was greater than 20% at the time chemotherapy was initiated. As observed in the rhesus macaque study , only transient chemoselection of P140K-modified cells was observed in the baboons following cycles of O 6 -BG and TMZ therapy. In the baboons, no pronounced myelosuppression was observed; however, based on canine studies indicating that greater than 20% P140K gene-modified cells in the periphery at the time of chemotherapy was significantly chemoprotective, the lack of observed hematologic toxicity was not unexpected. Transient selection was possibly due to insufficient MGMT suppression in unmodified cells using a single bolus dose of O 6 -BG, thereby not sensitizing the unmodified cells effectively to TMZ, so a fractionated regimen of O 6 -BG was tested with the second O 6 -BG infusion occurring 7 or 8 hr after the first dose based on murine data . Baboons treated with the fractionated regimen of O 6 -BG and ascending doses of TMZ ranging from 400 to 1400 mg/m 2 still only demonstrated transient selection of P140K-modified hematopoietic cells.
Since the fractionated O 6 -BG regimen did not result in stable selection following treatment with TMZ,BCNU, an alkylating agent producing a different DNA adduct and mechanism of cytotoxic action, was then tested in baboons. BCNU was administered intravenously in doses that ranged from 3.5 to 40 mg/m 2 approximately 45–60 min after the completion of the initial O 6 -BG bolus infusion. After treatment of several baboons with O 6 -BG and BCNU, the fraction of P140K-modified cells stably increased in all hematopoietic lineages analyzed. These studies were then extended to the macaque for preclinical assessment of chemoprotection and chemoselection in cells transduced with a P140K-expressing HIV-1-derived lentivirus vector. In the macaques J02370, T04228, and M05189, there was efficient selection of P140K-modified granulocytes, lymphocytes, red blood cells, and platelets following multiple cycles of fractionated O 6 -BG and BCNU. Some of the animals exhibited transient transaminitis, as indicated by moderate increases in alkaline phosphatase, and mild increases in ALT and AST, but no GI or pulmonary toxicity was observed and all animals were followed for a minimum of 4 years after transplant.
To better mimic a transplant protocol appropriate for the patient population that may receive this therapy, one additional macaque was transplanted with CD34 + cells gene-modified with a P140K-expressing lentivirus vector and infused with the cells following a reduced-intensity conditioning regimen consisting of single-agent busulfan (8 mg/kg) administered intravenously over 2 hr on days −2 and −1 relative to cell infusion (transplant, day 0). In this animal, approximately 4% gene-modified cells were observed in the peripheral blood 2 months after transplant, at which time a single cycle of fractionated O 6 -BG and BCNU was administered. Following chemotherapy, a sustained increase in gene-modified cells, indicating chemoselection, with minimal hematotoxicity was observed ( Figure 28.3 ). This data demonstrated that P140K-mediated chemoselection could be achieved in vivo even with low levels of gene-modified cells after transplant. In comparison to rhesus macaques used in previous studies that had attempted reduced-intensity busulfan conditioning prior to transplant of non-P140K-expressing lentivirus transduced CD34 + cells, observed engraftment was higher in the pigtailed macaque. These data indicated that the gene transfer vector, the cell dose received at the time of transplant, as well as the conditioning regimen employed prior to infusion of gene-modified cells could have a significant impact on initial engraftment and subsequent chemoprotection and chemoselection, but they also supported the ability of P140K-modified cells to be chemoselected from low levels of initial gene marking.
