Fig. 13.1
Redox dysregulation in melanomagenesis driven by genetic alterations. Cumulative experimental evidence indicates that melanoma progression (from benign nevi to the metastatic stage of the disease) is modulated by redox dysregulation. Alteration of specific genes with an established causative role in melanomagenesis may also impact cellular redox homeostasis and signaling, contributing to suppression or promotion of tumorigenesis and opening novel avenues for redox-directed therapeutic interventions
13.4.1 MC1R and MITF in Melanomagenesis and Redox Modulation
MC1R encodes a cyclic AMP-stimulating αs-type G protein-coupled receptor that controls pigment production through CREB (cAMP response element-binding protein)-dependent upregulation of MITF (encoding microphthalmia-associated transcription factor), a basic helix-loop-helix leucine zipper transcription factor that serves as the master regulator of melanocyte differentiation controlling the transcription of melanogenic genes including TYR, TYRP1, and DCT (Tsao et al. 2012; Denat et al. 2014). During solar UV-induced tanning, MC1R is activated by melanocortins including α-MSH (α-melanocyte stimulating hormone) producing eumelanin, whereas impaired receptor function results in pheomelanin production.
Importantly, MITF has been proposed to act as an oncogene in melanomagenesis stimulating the transcription of hypoxia inducible factor (HIF1A) with upregulation of VEGF expression, suggesting the existence of an α-MSH/MC1R-cAMP-CREB-MITF-HIF1α axis that contributes to melanoma progression (Busca et al. 2005). Moreover, MITF might play a causative role in conferring a genetic susceptibility to co-occurring melanoma and renal cell carcinoma based on the finding that a SUMOylation-defective MITF germline mutation predisposes to melanoma and renal carcinoma (Bertolotto et al. 2011). MITF repression by posttranslational SUMOylation is of fundamental importance since germline activational MITF gene mutations (Mi-E318K) inhibiting SUMOylation occur at a significantly higher frequency in genetically enriched patients affected with melanoma, renal cell carcinoma or both cancers when compared to controls. In addition, Mi-E318K enhanced MITF protein binding to the HIF1A promoter and increased its transcriptional activity compared to wild-type MITF.
Intriguingly, cumulative evidence suggests an important role of MITF in the control of redox homeostasis in melanocytes and melanoma cells. First, it has recently been shown that oncogenic BRAF regulates mitochondrial respiration and oxidative metabolism via PGC1α and MITF (Vazquez et al. 2013). Moreover, MITF regulates melanoma cell response to oxidative stress through transcriptional regulation of APE-1/Ref-1 [apurinic/apyrimidinic endonuclease/redox effector-1)], a key redox regulator and DNA endonuclease involved in repair of oxidative DNA damage (Liu et al. 2009). In addition, MITF has been shown to increase gene expression of NADPH oxidase type 4, a negative redox-regulator of melanogenesis (Liu et al. 2012b).
MC1R is highly polymorphic displaying over 60 variants in Caucasian skin, and germline variants of MC1R that disrupt the cAMP-MITF signaling cascade are present in 80 % of red haired individuals. It is now widely accepted that gene polymorphisms affecting MC1R that underlie pheomelanogenesis and impaired solar tanning represent predictive markers of melanoma susceptibility (Tsao et al. 2012). In addition, the MC1R genotype is an established determinant of the damage response of melanocytes to ultraviolet radiation, and MC1R signaling has now been recognized as a major regulator of redox homeostasis in melanocytes, acting in part through upregulation of antioxidant pathways that limit UV-induced photo-oxidative DNA damage with enhancement of OGG1-dependent repair of oxidative DNA base lesions (Kadekaro et al. 2012). Importantly, α-MSH-MC1R signaling controls intracellular redox status through control of antioxidant gene expression (e.g. HMOX1, GCLC, PRDX1) downstream of multiple redox-directed transcriptional modulators (MITF, APE-1, NRF2), and cells expressing dysfunctional MC1R variants display higher levels of constitutive oxidative stress, potentially contributing to ROS-induced BRAF mutations (Tsao et al. 2012; Marrot et al. 2008; Kadekaro et al. 2012; Denat et al. 2014). As an additional mechanism of action, pheomelanin precursors have been identified as potent redox-active sensitizers of UVA-induced photooxidative stress, and UVA-induced but not UVB-induced murine melanomagenesis has recently been shown to occur as a function of melanogenesis associated with oxidative DNA damage in melanocytes (Noonan et al. 2012). Strikingly, it has also been demonstrated that pheomelanogenesis contributes to solar UV-independent melanomagenesis thought to originate from the causation of melanogenesis-associated oxidative damage. Selective absence of pheomelanin synthesis reduced oxidative DNA and lipid peroxidation damage and was protective against melanoma development, whereas BRAFV600E expression in a pheomelanin-based ‘redhead’ mouse model with inactive MC1R led to an increased risk of invasive melanoma (Mitra et al. 2012).
13.4.2 CDKN2A in Melanomagenesis and Redox Modulation
The CDKN2A (cyclin-dependent kinase N2A) gene encoding the tumor suppressors p16INK4A and p14ARF has been identified as a crucial melanoma susceptibility factor, representing a critical target of inactivation (i.e. loss of function) at both the germline and somatic levels (Tsao et al. 2012). In familial cutaneous malignant melanoma, accounting for ~10 % of all cutaneous malignant melanoma cases, the most common known high-penetrance susceptibility gene is CDKN2A (followed by CDK4). The p16INK4A cyclin-dependent kinase inhibitor exhibits a cell cycle regulatory function by binding to CDK4/6 leading to a reduction in Rb phosphorylation and inhibition of the G1/S transition. The alternate reading frame product p14ARF interacts and antagonizes HDM2 (human double minute 2 homolog), an E3 ubiquitin-protein ligase that targets the tumor suppressor p53 towards proteasomal degradation. Homozygous deletion of CDKN2A deletes both p16INK4A and p14ARF, compromising the p16INK4A-CDK4-Rb and p14ARF-HDM2-p53 tumor suppressor pathways, and CDKN2A loss renders p53 mutation redundant, a finding consistent with the observation that the p53 mutation rate is low in melanoma. Inactivation of the p16 INK4A gene through germ-line mutations is associated with hereditary melanoma predisposition, and most CDKN2A germline mutations that confer melanoma risk occur in exons 1α and 2 (encoding portions of p16INK4a) suggesting that p16IN4a is the preferentially targeted and functionally dominant component of CDKN2A.
Recently, a novel and unexpected role of p16INK4A in redox regulation of melanomagenesis has emerged (Jenkins et al. 2011, 2013). In human melanocytes, p16INK4A has been identified as a redox responsive factor, undergoing rapid upregulation following solar UV- or hydrogen peroxide-dependent p38 MAPK signaling. Strikingly, genetic antagonism of p16INK4A increases oxidative stress and oxidative DNA damage in melanocytes suggesting that p16INK4A plays a heretofore unrecognized role as a cytoprotective redox modulator in melanocytes. The increased susceptibility of melanocytes to oxidative stress as a result of p16INK4A depletion suggests a dual tumor suppressor function of p16INK4A, preventing oncogenic oxidative DNA lesions by controlling oxidative stress on one hand and allowing DNA repair after induction of cell cycle arrest through the p16INK4A-CDK4-Rb pathway on the other. More recently, it has been demonstrated that specific familial melanoma-associated p16INK4A mutations can selectively compromise either one of these two tumor suppressor functions mediated by distinct regions of the protein. Indeed, familial melanoma-associated point mutants spanning the p16INK4A coding region displayed differential effects on cell cycle regulation and modulation of oxidative stress, with several mutations impairing cell cycle (R24Q, R99P, V126D) or redox functions (A36P, A57V, P114S) selectively, a finding indicative of cell cycle- and redox-directed activities of p16INK4A that can be mutually uncoupled in human melanoma cells.
Redox modulation exerted by various tumor suppressor genes represents a molecular function that reaches beyond their canonical activities and has attracted considerable interest. Similar to an emerging antioxidant function of p16 suppressing melanomagenesis, the CDKN1A-encoded tumor suppressor p21 (CIP1/WAF1) has been shown to downregulate oxidative stress by increasing stability of NRF2 with upregulation of antioxidant NRF2-target gene expression (Chen et al. 2009). Indeed, other tumor suppressor genes including TP53, the upstream regulator of CDKN1A, and BRCA1 are known to control oxidative DNA base lesions and redox homeostasis through upregulation of various redox-directed target genes as reviewed recently (Vurusaner et al. 2012).
13.4.3 NRAS and BRAF in Melanomagenesis and Redox Modulation
Activation of the ERK-mitogen activated protein kinase (MAPK) pathway due to oncogenic mutation of NRAS and BRAF is a primary mechanistic driver in melanomagenesis, and activating mutations in the NRAS and BRAF oncogenes, which are both mutually exclusive, have been identified in a majority of melanomas (Davies et al. 2002; Tsao et al. 2012; Kunz 2014). Activating mutations in the NRAS small GTPase are present in 15–20 % of all melanomas with the majority of mutations represented by NRASQ61R or NRASQ61K. In addition to the MAPK pathway, NRAS simultaneously activates the phosphatidyl-inositol 3-kinase (PI3K) pathway. RAS-driven tumorigenesis has been the target of various stress response pathway-directed therapeutic strategies but no specific interventions targeting NRAS in melanoma have been reported (Yang and Stockwell 2008; De Raedt et al. 2011).
BRAF is a serine/threonine kinase involved in the Ras-Raf-MAPkinase pathway. Remarkably, activating mutations in BRAF are the most common genetic alterations in melanoma, and gain-of-function mutations of the BRAF gene are found in the vast majority of benign nevi and primary melanomas representing a key event in early melanomagenesis (Davies et al. 2002). Numerous BRAF mutations have been documented, the most frequent of which results in a valine-to-glutamic acid change at position 600 (BRAFV600E) constitutively activating this protein kinase upstream of MEK and ERK1/2, which in turn phosphorylates transcription factors that control proliferative and anti-apoptotic targets. Although UV exposure is a major risk factor for melanoma, BRAFV600E originates from a T > A transversion, rather a common UV-associated C > T transition, an unexplained phenomenon that has been attributed to oxidative stress-induced mutagenesis downstream of melanogenesis-derived ROS formation (Tsao et al. 2012).
Importantly, downstream of BRAFV600E or NRASQ61R expression, oncogene-induced senescence is a common occurrence that determines the senescent phenotype displayed by melanocytes in benign nevi. Indeed, benign melanocytic lesions harboring a mutant allele of BRAF or NRAS display crucial hallmarks of senescence including proliferative arrest, p16INK4A upregulation, and senescence-associated β-galactosidase expression. It has been demonstrated earlier that receptor tyrosine kinase- or NRASQ61K-signaling leads to redox dysregulation characterized by accumulation of high ROS levels in melanocytes, an oxidative stress-induced senescent phenotype suppressed by antioxidant supplementation (Leikam et al. 2008). Interestingly, the ROS-generating oxidases Nox1 and Nox4 are an important source of endogenous oxidative stress contributing to oncogenic Ras-induced premature senescence (Kodama et al. 2013). In addition, mitochondrial respiratory electron leakage might contribute to ROS-mediated oncogene-driven senescence, and oncogene-dependent regulation of mitochondrial function, oxidative metabolism, and redox regulation in melanoma have recently attracted considerable interest (Theodosakis et al. 2014). Importantly, the mitochondrial gatekeeper pyruvate dehydrogenase (PDH) has been identified as a crucial mediator of BRAFV600E-induced oxidative stress-mediated senescence, accompanied by simultaneous suppression of the PDH-inhibitory enzyme pyruvate dehydrogenase kinase 1 (PDK1) and induction of the PDH-activating enzyme pyruvate dehydrogenase phosphatase 2 (PDP2) (Kaplon et al. 2013). Activation of PDH-enhanced pyruvate metabolism through the tricarboxylic acid cycle causes increased mitochondrial respiration and ROS formation, and engineered normalization of either PDK1 or PDP2 expression levels resulting in PDH inhibition enables BRAFV600E-driven melanoma development by abrogation of BRAFV600E-induced senescence. The observation that depletion of PDK1 eradicated melanoma subpopulations resistant to targeted BRAF inhibition causing regression of established melanoma tumors suggests that therapeutic interventions upregulating pyruvate mitochondrial metabolism can be harnessed to antagonize tumor growth in BRAF-driven melanoma by reestablishing oncogene-induced senescence.
Recently, it has been reported that oncogenic BRAF regulates mitochondrial oxidative metabolism via the mitochondrial master regulator PGC1α and MITF (Vazquez et al. 2013). Melanomas displaying activation of the BRAF/MAPK pathway have suppressed levels of MITF (an established BRAF target) and PGC1α resulting in decreased oxidative mitochondrial metabolism. In contrast, BRAF inhibition induces an oxidative phosphorylation gene program and mitochondrial biogenesis together with increased expression of PGC1α, indicating that treatment of BRAF-mutated melanomas with BRAF inhibitors causes a functional dependence on oxidative phosphorylation. These data suggest an adaptive metabolic program that limits the efficacy of BRAF inhibitors, and mitochondrial uncouplers undermining oxidative phosphorylation may therefore have therapeutic use in combination with BRAF inhibitors (Haq et al. 2013).
In addition to studies that substantiate the vemurafenib-induced mitochondrial oxidative metabolism in BRAFV600E melanomas expressing PGC1α, mitochondrial oxidative stress has also been identified as a specific vulnerability characteristic of melanoma cells resistant to BrafV600E inhibitors (Corazao-Rozas et al. 2013). The observation that elevated ROS levels rendered vemurafenib-resistant melanoma cells prone to cell death induced by pro-oxidants including the investigational redox drug elesclomol suggests that the mitochondrial oxidative signature of resistant melanoma constitutes a druggable redox vulnerability, an intriguing hypothesis to be pursued by ongoing preclinical and clinical research.
13.4.4 KIT in Melanomagenesis and Redox Modulation
The KIT gene encodes the transmembrane receptor tyrosine kinase c-KIT [stem cell growth factor receptor (SCFR), CD117] expressed by melanocytes, mast cells, interstitial cells of Cajal, as well as hematopoietic stem cells and multipotent/common myeloid progenitors. Activating KIT mutations, KIT L576P being the most common variant, are observed in 14 and 18 % of acral lentiginous and mucosal melanomas, respectively, but occurrence in skin melanomas is marginal (Tsao et al. 2012; Hodi et al. 2013; Kunz 2014). KIT-activation may also occur through gene amplifications observed in a significant percentage of melanoma cases. c-KIT dimerization with activation of its tyrosine kinase activity occurs upon extracellular binding of stem cell factor (SCF), a c-KIT ligand and cytokine involved in hematopoiesis, spermatogenesis, and melanogenesis. Melanoblast migration is a SCF/c-KIT controlled process through which SCF guides melanoblasts expressing c-KIT from the neural crest to their epidermal locations, and SCF also regulates survival and proliferation of fully differentiated melanocytes. The activation of c-KIT leads to the activation of multiple signaling cascades, including the RAS/ERK, PI3-Kinase, Src kinase, and JAK/STAT pathways controlling cellular proliferation and survival in melanoma cells (Smalley et al. 2009). Moreover, c-KIT proliferative signaling depends on MITF activity in melanoma, where c-KIT stimulation leads to the activation of MITF specifically through the c-KIT phosphorylation sites Y721 (PI3 kinase binding site), Y568 and Y570 (Src binding site) (Phung et al. 2011).
Little is known about the role of c-Kit in redox modulation of melanomagenesis. SCF/c-KIT-dependent protection of keratinocytes from ROS-induced apoptosis has recently been demonstrated, and c-KIT anti-apoptotic activity regulating cellular redox state and loss of mitochondrial transmembrane potential have been described (Lee 1998; Lam et al. 2011). Interestingly, c-KIT redox mechanisms have been shown to play critical roles within stem cell niches, and c-KIT expression in cardiac precursor cells exhibits antioxidant activity through downregulation of NOX2 and its subunit p67(PHOX), while overexpression of NOX2 and NOX4 antagonizes KIT expression initiating cardiac lineage commitment and differentiation (Nadworny et al. 2013).
Due to the pronounced c-KIT-antagonism displayed by clinically used BCR-ABL-directed kinase inhibitor drugs, representing standard-of-care medications in chronic myelogenous leukemia, c-KIT-targeted pharmacological intervention for the treatment of acral lentiginous melanoma has quickly entered the stage of clinical practice (Hodi et al. 2013; Karimkhani et al. 2014).
13.4.5 AKT3-PTEN in Melanomagenesis and Redox Modulation
Activation of the PI3K pathway is a common genetic event in melanoma, with hyperactivation of the oncogenic kinase AKT3, observed in up to 60 % of sporadic melanomas, thought to originate from both AKT3 (V-akt murine thymoma viral oncogene homolog 3; protein kinase B gamma) gene amplification and decreased activity of the AKT antagonist and tumor suppressor PTEN (phosphatase and tensin homolog deleted on chromosome 10) (Tsao et al. 2012; Kwong and Davies 2013). Among three AKT isoforms (AKT1/PKBα, AKT2/PKBβ, and AKT3/PKBγ) expressed in melanocytes and melanoma cells, AKT3 is the predominantly active member involved in cancer cell survival signaling, documented to be upregulated in 17 % of benign nevi, 43 % of dysplastic nevi, 49 % of primary melanomas, and 77 % of metastatic melanomas (Dai et al. 2005).
It has recently been shown that abrogation of BRAFV600E-induced senescence by PI3K pathway activation contributes to melanomagenesis (Vredeveld et al. 2012). AKT3 has been shown to phosphorylate BRAFV600E attenuating the activity of the mutant protein to levels that promote rather than inhibit melanoma tumor progression, and acute shRNA-mediated depletion of PTEN prompted tumor progression in established murine BRAFV600E-driven nevi. In the same study, pharmacologic PI3K-inhibition in melanoma cells suppressed proliferation and induced the senescence-associated tumor suppressor p15INK4B eliminating subpopulations resistant to targeted BRAFV600E inhibition, findings that suggest feasibility of therapeutic reactivation of senescence and elimination of melanoma cells refractory to BRAFV600E by PI3K inhibition.
In support of a redox-directed involvement of AKT-signaling in melanomagenesis, it has been shown that the crucial conversion from radial growth phase (RGP) to the invasive vertical growth phase (VGP) of melanoma is orchestrated by AKT overexpression causing NAD(P)H oxidase 4 (NOX4)-dependent ROS production (Govindarajan et al. 2007; Liu-Smith et al. 2014). In malignant melanoma cell lines, NOX4 was instrumental in causing prooxidant deviations from redox homeostasis, and Nox4-directed siRNA intervention decreased ROS production with inhibition of anchorage-independent cell growth and tumorigenicity in nude mice. In melanoma patient samples, NOX4 expression was detectable in approximately one third of tumor specimens suggesting the association of NOX4 expression with melanomagenesis (Yamaura et al. 2009). It was also demonstrated that NOX4 expression supports the transformed phenotype by orchestrating the redox modulation of G2/M cell cycle progression involving CDK1 and CDC25C. NOX4-dependent upregulation of constitutive oxidative stress and redox modulation of FAK (focal adhesion kinase) have been shown to control melanoma survival mediated via increased FAK-phosphorylation downstream of ROS-dependent inactivation of redox-sensitive phosphatases including PTEN and protein tyrosine phosphatases (Ribeiro-Pereira et al. 2014). More recently, a mechanism by which NOX4-dependent ROS production could be harnessed for the induction of TRAIL (TNF-related apoptosis-inducing ligand)-induced melanoma cell apoptosis was elucidated using the PI3-kinase inhibitor wortmannin causing a shift towards enhanced pro-apoptic (Thr167) and reduced anti-apoptotic phosphorylation (Ser184) of BAX (Quast et al. 2013). Targeting signaling through the PI3K-AKT pathway in malignant melanoma is an important goal of ongoing preclinical and clinical drug development efforts, and dual pathway inhibition using PI3K and MEK inhibitors that attenuate signaling through the PI3K/AKT/mTOR and RAS/RAF/MEK/ERK pathways is currently explored in ongoing clinical trials in malignant melanoma with BRAF or NRAS mutations (Britten 2013; Kwong and Davies 2013).
Experimental and clinical evidence suggests that expression and activity of the phosphatase PTEN is compromised in up to 43 % of melanoma patients (Mikhail et al. 2005). In the majority of melanomas PTEN impairment is caused by either chromosomal alteration, mutation, methylation-induced transcriptional silencing, or microRNA-dependent regulation (Tsao et al. 2012). In melanoma, PTEN expression has been shown to be a critical regulator of cell proliferation and survival acting through modulation of AKT3 phosphorylation status, and a murine spontaneous melanoma model (BRAFV600E/+/PTEN−/−/Tyr-CRE+) combining expression of BRAFV600E with PTEN gene silencing elicits development of melanoma with 100 % penetrance, short latency, and metastases observed in lymph nodes and lungs (Dankort et al. 2009). Oxidation of the PTEN active site cysteine residue (C124) by ROS has long been recognized as a common mechanism regulating several key phosphatases including PTEN, inactivating its lipid phosphatase and tumor suppressor functions as observed in multiple cancer cell lines (Kwon et al. 2004; Silva et al. 2008). However, redox modulation of PTEN has not been substantiated in melanoma cells.
13.4.6 RAC1 in Melanomagenesis and Redox Modulation
Recently, RAC1 P29S (Ras-related C3 botulinum toxin substrate 1) was identified as a recurrent UV-induced signature mutation in human melanoma tumors, representing the third most frequent gain-of-function mutation described in melanoma (Hodis et al. 2012; Tsao et al. 2012; Kunz 2014). Intriguingly, the mutated RAC1 P29S maintains intrinsic GTP hydrolysis and is spontaneously activated by substantially increased inherent GDP/GTP nucleotide exchange displaying high binding activity to downstream targets inducing melanocyte proliferation and migration (Davis et al. 2013). Rho GTPase family proteins (including RAC1, RhoA, Cdc42) are crucial regulators of cytoskeletal rearrangement and adhesion enabling cancer metastasis, and Rac1 is known to impact multiple cellular processes including actin-based cytoskeletal reorganization, DNA synthesis, cell transformation, motility, and -importantly- superoxide/ROS production thought to impact the aforementioned biological readouts. Overexpression of RAC1 has been observed in various tumour types, and expression of constitutively active RAC1 promotes cellular transformation, consistent with RAC1-dependent survival signaling through NFκB- and PI3K/AKT-dependent pathways (Singh et al. 2004). Pro-metastatic signaling via RAC-1 and ROS downstream of c-Met/hepatocyte growth factor receptor (HGF-R) proto-oncogene action has been demonstrated recently in melanoma, and catalytic SOD-mimetics (e.g. EUK-134) attenuated c-Met signaling-dependent ERK activation with inhibition of anchorage-independent growth, a finding consistent with a critical role of RAC1 and ROS in HGF/c-MET pro-metastatic signaling in melanoma (Ferraro et al. 2006). Importantly, RAC1 is a crucial activator of NOX1, and, intriguingly, NOX1 over-expression has been identified as a causative factor in ROS-dependent melanoma cell invasion characterized by induction of matrix metalloproteinase expression (MMP2, MMP9) and epithelial–mesenchymal transition (Liu et al. 2012; Liu-Smith et al. 2014). RAC1 activation upstream of NOX-dependent ROS formation has also been shown to control FAK (Focal Adhesion Kinase)-dependent motility in cultured melanoma cells (Kim et al. 2008). Based on the emerging mechanistic involvement of a RAC1-NOX1-ROS axis in melanoma invasiveness and the recent identification of activational oncogenic RAC1 mutations in human melanoma tumors, it has been proposed that activation of RAC1 may represent a valuable biomarker of melanoma progression (Liu-Smith et al. 2014). Moreover, due to the availability of NOX-inhibitory therapeutics, RAC1- and NOX-dependent redox dysregulation represents an attractive melanoma drug target to be explored by future preclinical and clinical efforts (Block and Gorin 2012).
13.4.7 KEAP1 in Melanomagenesis and Redox Modulation
Recently, exome sequencing of acral lentiginous melanoma (ALM), a subtype harboring the BRAF V600E mutation in only 10 % of cases, identified a somatic inactivating frameshift mutation in KEAP1 (codon 507, exon 4), the gene encoding Kelch-like ECH-associated protein 1, a molecular change resulting in aberrant NRF2 (nuclear factor erythroid-derived 2-like 2) activation and increased intrinsic drug resistance characteristic of AML (Kunz 2014; Miura et al. 2014). KEAP1 is a redox-sensitive negative regulator of the basic leucine zipper (bZIP) transcription factor NRF2, a master regulator of the cellular antioxidant electrophilic stress response (Zhang 2010). KEAP1 is a substrate adaptor essential to Cul3-dependent ubiquitination of NRF2 followed by proteasomal degradation (Villeneuve et al. 2010). Oxidative or electrophilic stressors target critical cysteine residues in KEAP1, disrupting the KEAP1-Cul3 ubiquitination system leading to NRF2 accumulation followed by nuclear translocation and NRF2-dependent transcription of target genes with an ARE (antioxidant response element)-promotor sequence. Indeed, NRF2 target genes encode important regulators of cellular oxidative stress including components controlling biosynthesis and redox status of glutathione (e.g. SLC7A11, GCLC, GCLM, GSR), important determinants of cancer progression and chemoresistance (Zhang 2010; Mitsuishi et al. 2012a, b).
Strikingly, recent data have shown that constitutive activation of NRF2 can occur during later stages of tumorigenesis, representing a remarkable redox adaptation of cancer cells that enables chemoresistance and enhanced tumor cell survival under adverse conditions (Wang et al. 2008; Zhang 2010; Jaramillo and Zhang 2013). Moreover, the functional expansion of NRF2 may also contribute to metabolic reprogramming of cancer cells triggered by proliferative signals (Mitsuishi et al. 2012a, b). Consistent with these findings, the single nucleotide deletion observed in AML generates a mutant KEAP1 protein with impaired DGR/Kelch domain essential to KEAP1-NRF2 interaction, causing pronounced upregulation of NRF2 and resistance of AML cells to chemotherapeutics that impose electrophilic stress including cisplatin and dacarbazine. Due to the emerging role of NRF2 in redox adaptation of tumors, current drug discovery efforts aim at the development of NRF2 inhibitors for cancer cell chemosensitization (Ren et al. 2011).
13.4.8 MAP3K5 (ASK1) in Melanomagenesis and Redox Modulation
Recent data support the intriguing hypothesis that melanoma cells counterbalance elevated levels of oxidative stress that increase invasive and metastatic performance through down-regulation of oxidative stress-triggered cell death pathways including the ASK1 (apoptosis signal-regulating kinase 1; mitogen-activated protein kinase kinase kinase 5) pathway. Indeed, exome sequencing has identified frequent somatic mutations in MAP3K5 (and MAP3K9) in metastatic melanoma with 24 % of melanoma cell lines displaying mutations in the protein-coding regions of either MAP3K5 or MAP3K9 (Stark et al. 2012; Kunz 2014). Structural modeling of the kinase domain predicted the inactivating nature of the mutations, confirmed by in vitro biochemical assays that revealed reduced kinase activity of MAP3K5 I780F and MAP3K9 W333* variants. Vice versa, overexpression of MAP3K5 or MAP3K9 variants in HEK293T cells reduced phosphorylation of downstream MAP kinases. Furthermore, siRNA-based target attenuation of ASK1 in melanoma cells led to increased resistance to temozolomide treatment, suggesting that these mutations may cause chemoresistance in melanoma. ASK1 is a redox-sensitive member of the MAPKKK family, which activates both c-Jun N-terminal kinase (JNK) and p38 MAPK pathways and constitutes a pivotal signaling factor in oxidative stress-induced apoptosis (Kawarazaki et al. 2014). Reduced thioredoxin binds to ASK1 inhibiting its activity, and somatic mutations in MAP3K5 have recently been shown to attenuate its proapoptotic function in melanoma through increased binding to thioredoxin (Prickett et al. 2014). Importantly, ASK1 may display dual functions as both tumor promoter and suppressor, opposing activities that depend on cell type and tissue context, and its specific role in melanomagenesis remains to be defined (Soga et al. 2012).
13.4.9 MYC in Melanomagenesis and Redox Dysregulation
The MYC proto-oncogene encoding the helix-loop-helix transcription factor c-MYC is involved in the control of proliferation, differentiation, and apoptosis, and structural alterations of the 8q24 chromosomal locus regulate MYC expression contributing to tumorigenesis. MYC activation is a frequent event in human melanoma representing a prognostic marker that can predict shorter overall survival (Chana et al. 1998, 2001; Grover et al. 1999). Indeed, upregulated chromosomal copy number changes at 8q24 harboring MYC are highly linked to poor prognosis in cutaneous malignant melanoma suggesting that MYC copy number gains play an important role in the aggressive clinical behavior of melanomas (Gerami et al. 2011; Pouryazdanparast et al. 2012). Moreover, c-MYC downregulation can sensitize melanoma cells to chemo- and radiotherapy, and high c-MYC expression enhances melanoma growth in SCID mice and facilitates interferon-α resistance in human melanoma (Biroccio et al. 2001; Tulley et al. 2004; Leikam et al. 2014; Meierjohann 2014).
It is now established that c-MYC overexpression plays an essential role in melanomagenesis through continuous suppression of oncogene (BRAFV600E or NRASQ61R)-induced senescence in melanoma cells enabling the crucial transition from benign to dysplastic nevus, and genetic attenuation of c-MYC expression has indeed been shown to induce senescence in metastatic melanoma cells (Michaloglou et al. 2005; Zhuang et al. 2008). c-MYC-driven evasion of oxidative stress-induced melanocyte senescence downstream of activated receptor tyrosine kinase signaling has been demonstrated, and a role of MIZ1 (Msx-interacting-zinc finger), another zinc finger transcription factor and growth suppressing c-MYC interaction partner, in mediating melanocyte senescence was substantiated based on the observation that either c-MYC overexpression or MIZ1 knockdown antagonized senescence with attenuation of endogenous ROS formation and DNA damage (Leikam et al. 2014; Meierjohann 2014). As a mediator of the oxidative stress-directed effects of c-MYC and MIZ1 modulation, the c-MYC target gene CTH (encoding cystathionase), an enzyme involved in the control of cellular L-cysteine and glutathione synthesis, was identified. Consistent with a causative role of CTH as new c-MYC target gene with an important function in senescence evasion, pharmacological or genetic CTH target inhibition reestablished senescence in human melanoma cells attenuating melanoma cell proliferation, H2O2 resistance, and soft agar growth.
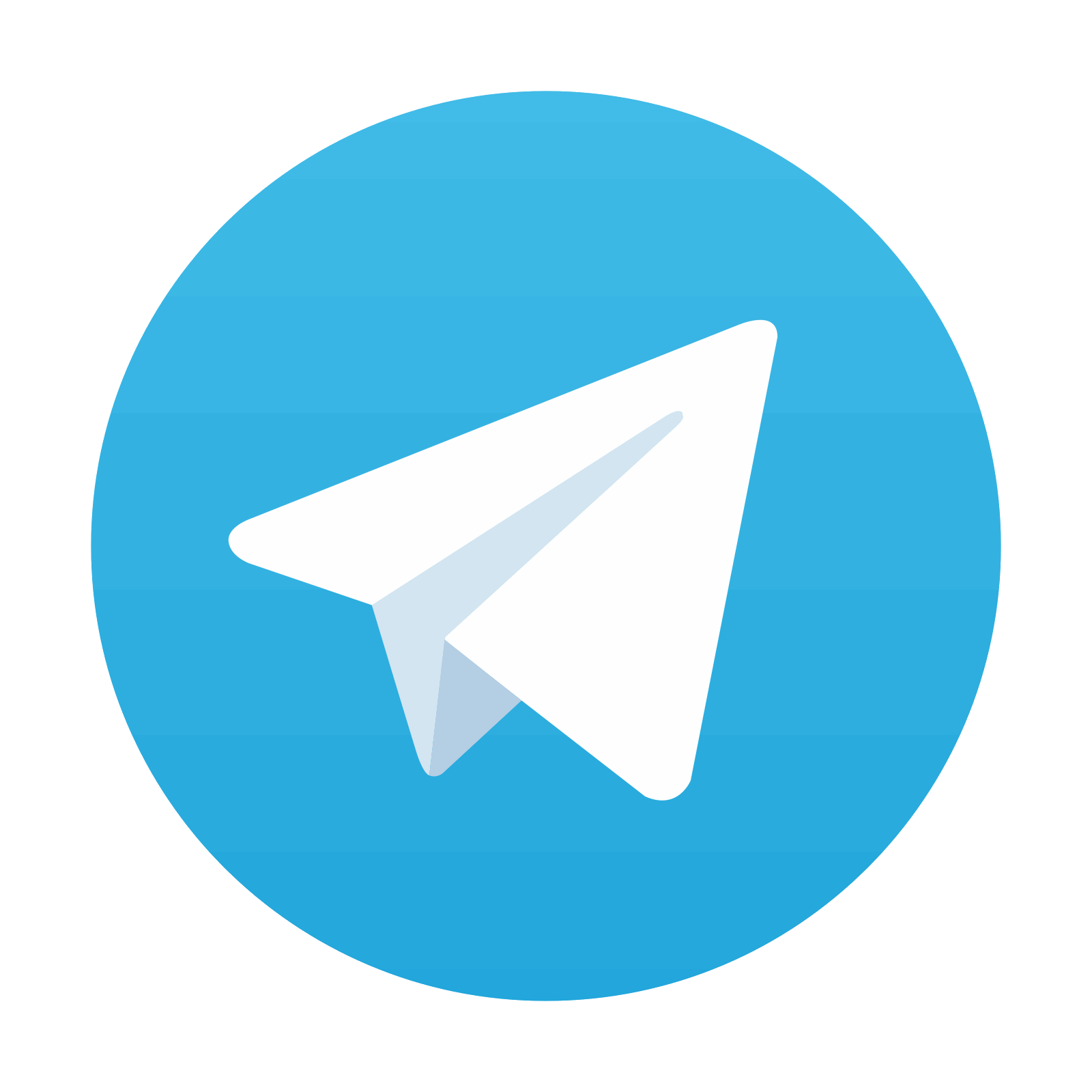
Stay updated, free articles. Join our Telegram channel

Full access? Get Clinical Tree
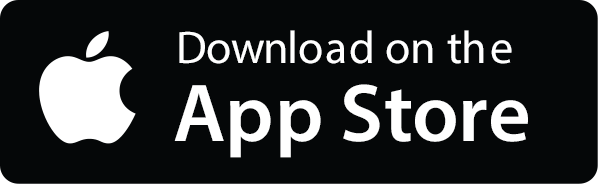
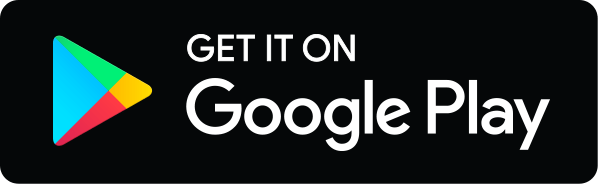