The presence of FLT3 mutations in acute myeloid leukemia (AML) carries a particularly poor prognosis, making the development of FLT3 inhibitors an imperative goal. The last decade has seen an abundance of clinical trials using these drugs alone or in combination with chemotherapy. This culminated with the recent approval by the US Food and Drug Administration of Midostaurin for the treatment of FLT3-mutated AML. Initial success has been followed by the emergence of clinical resistance. Although novel FLT3 inhibitors are being developed, studies into mechanisms of resistance raise hope of new strategies to prevent emergence of resistance and eliminate minimal residual disease.
Key points
- •
Single-agent FLT3 inhibitors can induce remission without cure in FLT3-internal tandem duplication (ITD) acute myeloid leukemia (AML), but to eliminate the last bastion of minimal residual disease (MRD), additional interventions are required.
- •
Resistance to FLT3 inhibitors may be facilitated by signals from the microenvironment that allow survival of AML cells in the presence of these agents.
- •
Pharmacokinetics and pharmacodynamics do not always correlate and pharmacodynamics is a better predictor of efficacy when using FLT3 inhibitors.
- •
FLT3 inhibitors are potent in clearing circulating blasts, yet this does not always translate into similar effects on bone marrow blasts.
- •
Accumulation of additional mutations in the FLT3-ITD AML clone allows for emergence of clones with cell intrinsic resistance to FLT3 inhibitors.
Introduction
FLT3-mutated acute myeloid leukemia (AML) represents about one-third of all cases of newly diagnosed AML. Two classes of mutations are frequently found: activation loop or tyrosine kinase domain mutations (TKD; about 5%–10% of patients) and in-frame, internal tandem duplication (ITD; about 23% of patients). Although the prognostic impact of de novo FLT3-TKD mutations is usually minimal, the presence of FLT3-ITD mutations confers a poor prognosis in AML and the frequency of the mutated allele (allelic ratio) as well as the length of the tandem repeat (ITD length) correlate with worse outcomes.
FLT3 is a class III receptor tyrosine kinase that dimerizes upon ligand binding and undergoes autophosphorylation to initiate multiple intracellular signaling programs. These pathways, including PI3K/AKT, Jak/STAT, and Ras/MAPK, transduce signals resulting in survival and proliferation of target cells ( Fig. 1 ).

During normal hematopoiesis, FLT3 is expressed in early progenitor cells and the receptor is downregulated while cells differentiate down the myeloid lineage. Patients with FLT3-mutated AML not only have constitutively active signaling but, given the lack of differentiation, these AML blasts continue to express high levels of this mutated protein in addition to the wild-type FLT3 receptor.
This sustained survival and proliferation signal is the hallmark of FLT3-mutated AML. The clinical presentation of these patients is dominated by hyperleukocytosis, myeloblastic/monoblastic differentiation and usually de novo (as opposed to secondary) AML. Compared with other poor prognostic factors in AML, the presence of an FLT3-ITD mutation does not have a major impact on achieving remission after induction chemotherapy, but the remission is characteristically short lived and relapse often occurs during cycles of consolidation (sometimes while an allogeneic donor search is underway). There is a consensus that prompt blood or marrow transplantation in first remission can improve the outcome in this disease even in the absence of FLT3 inhibitors. Nevertheless, the high rate of relapse even posttransplantation makes this approach alone suboptimal.
In the setting of relapsed disease, the FLT3-ITD mutation is often present at a high allelic ratio, and the mutated receptor in the malignant clone renders this disease resistant to chemotherapy. At this stage, the blasts are addicted to signaling downstream of this RTK and, thus, sensitive to FLT3 inhibitors. Thus, the clinical development of small molecule inhibitors that target mutant FLT3 is an active area of research. More than 60 clinical trials are either open or completed testing different FLT3 inhibitors as single agents or in combination with other therapeutic approaches in AML (available at: clinicaltrials.gov ). Full updates on the clinical development of these strategies can be found in this issue (See Jacqueline S. Garcia and Richard M. Stone’s article, “ The Development of FLT3 Inhibitors in Acute Myeloid Leukemia ,” in this issue). Herein, we focus our attention on mechanisms of resistance to FLT3 inhibitors and strategies to overcome such resistance and achieve cure in FLT3-mutated AML.
Introduction
FLT3-mutated acute myeloid leukemia (AML) represents about one-third of all cases of newly diagnosed AML. Two classes of mutations are frequently found: activation loop or tyrosine kinase domain mutations (TKD; about 5%–10% of patients) and in-frame, internal tandem duplication (ITD; about 23% of patients). Although the prognostic impact of de novo FLT3-TKD mutations is usually minimal, the presence of FLT3-ITD mutations confers a poor prognosis in AML and the frequency of the mutated allele (allelic ratio) as well as the length of the tandem repeat (ITD length) correlate with worse outcomes.
FLT3 is a class III receptor tyrosine kinase that dimerizes upon ligand binding and undergoes autophosphorylation to initiate multiple intracellular signaling programs. These pathways, including PI3K/AKT, Jak/STAT, and Ras/MAPK, transduce signals resulting in survival and proliferation of target cells ( Fig. 1 ).
During normal hematopoiesis, FLT3 is expressed in early progenitor cells and the receptor is downregulated while cells differentiate down the myeloid lineage. Patients with FLT3-mutated AML not only have constitutively active signaling but, given the lack of differentiation, these AML blasts continue to express high levels of this mutated protein in addition to the wild-type FLT3 receptor.
This sustained survival and proliferation signal is the hallmark of FLT3-mutated AML. The clinical presentation of these patients is dominated by hyperleukocytosis, myeloblastic/monoblastic differentiation and usually de novo (as opposed to secondary) AML. Compared with other poor prognostic factors in AML, the presence of an FLT3-ITD mutation does not have a major impact on achieving remission after induction chemotherapy, but the remission is characteristically short lived and relapse often occurs during cycles of consolidation (sometimes while an allogeneic donor search is underway). There is a consensus that prompt blood or marrow transplantation in first remission can improve the outcome in this disease even in the absence of FLT3 inhibitors. Nevertheless, the high rate of relapse even posttransplantation makes this approach alone suboptimal.
In the setting of relapsed disease, the FLT3-ITD mutation is often present at a high allelic ratio, and the mutated receptor in the malignant clone renders this disease resistant to chemotherapy. At this stage, the blasts are addicted to signaling downstream of this RTK and, thus, sensitive to FLT3 inhibitors. Thus, the clinical development of small molecule inhibitors that target mutant FLT3 is an active area of research. More than 60 clinical trials are either open or completed testing different FLT3 inhibitors as single agents or in combination with other therapeutic approaches in AML (available at: clinicaltrials.gov ). Full updates on the clinical development of these strategies can be found in this issue (See Jacqueline S. Garcia and Richard M. Stone’s article, “ The Development of FLT3 Inhibitors in Acute Myeloid Leukemia ,” in this issue). Herein, we focus our attention on mechanisms of resistance to FLT3 inhibitors and strategies to overcome such resistance and achieve cure in FLT3-mutated AML.
Mechanisms that allow survival of FLT3-mutated acute myeloid leukemia cells during treatment with FLT3 inhibitors
Wisdom gathered from more than 7 decades of antimicrobial use to treat infections tells us that resistance to treatment is either acquired via genetic adaptation or relies on clones already present that are selected under survival pressure. In AML, resistance to chemotherapy can take either one of these two forms. Elegant genetic studies have shown that AML at diagnosis is a polyclonal disease, whereas relapsed AML is usually more oligoclonal. Most of the time, the relapsed clone can be retrospectively found at presentation but at much lower frequency. This pattern seems to be the case with FLT3-mutated AML relapsing after induction chemotherapy. Most of the initial malignant subclones are sensitive to treatment and the patient achieves a clean complete remission. Nevertheless, some clones survive chemotherapy and are responsible for disease relapse. At relapse, the mutant allelic ratio is often higher than what was seen at diagnosis.
In contrast, it is probable that the development of resistance to FLT3 inhibitors is at least in part dependent on some genetic or epigenetic events. For these genetic and epigenetic events to take place, some FLT3 mutated cells will need to have survived the initial treatment.
Initial studies using FLT3 inhibitors demonstrated clearance of circulating FLT3-ITD blasts, but there was little to no effect on bone marrow blasts. More potent and selective FLT3 inhibitors are effective at differentiating most bone marrow blasts, but since these drugs cannot eliminate minimal residual disease as single agents, some leukemia cells, perhaps residing in the stem cell niche, must survive the treatment with the inhibitor.
Potential mechanism that contribute to survival of leukemia blasts in the stem cell niche during treatment with FLT3 inhibitors fall into two categories: a) signaling mechanisms that bypass the effective inhibition of FLT3 receptor and b) suboptimal pharmacokinetics and phamacodynamics in the stem cell niche.
Bypassing the FLT3 Receptor
This early evidence pointed toward stromal mediated mechanisms of survival. To this end, coculture of FLT3-ITD AML cells with bone marrow mesenchymal stroma also protects the blasts from quizartinib as well as Fl-700. In these settings, soluble as well as membrane-bound cytokines that seem to play a major role include CXCL12, angiopoietins, as well as vascular endothelial growth factor/epidermal growth factor/insulin-like growth factor and granulocyte colony stimulating factor/granulocyte macrophage colony stimulating factor/tumor necrosis factor. Similarly, patients with FLT3-ITD AML treated with quizartinib develop high levels of stromal-derived fibroblast growth factor 2 in bone marrow mesenchymal cells. Pathways downstream of fibroblast growth factor receptor 1 maintain active RAS/MAPK signaling in FLT3-ITD blasts treated with quizartinib. Most patients with FLT3-ITD continue to have a wild-type allele of FLT3. This receptor is rather resistant to FLT3 inhibitors but sensitive to FLT3 ligand. Because high levels of FLT3 ligand are found in the bone marrow microenvironment during induction therapy, ligand-induced activation of the wild-type FLT3–MAPK pathway may provide survival signals to leukemic blasts, even in the presence of effective TKI treatment.
Potent FLT3-ITD inhibition results in apoptosis of AML blasts in the absence of stroma. The presence of bone marrow mesenchymal stroma rescues the blasts from apoptosis through extracellular receptor kinase (ERK)-mediated signaling but not STAT5. In this context, inhibition of FLT3-ITD induces G1 arrest, possibly via downregulation of cyclin D2/cyclin D3 and subsequent dephosphorylation of Rb. Individual FLT3 inhibitors may have differential effects, for instance, contact with niche cells expand FLT3-ITD blasts treated with SU5615 but not sorafenib.
Suboptimal Pharmacokinetics and Pharmacodynamics
Complete and sustained inhibition of FLT3-ITD is paramount for the successful elimination of the malignant clone. Initial studies with midostaurin clearly demonstrated the impact of hepatic drug metabolism and CYP3A4 activity in particular of this drug plasma pharmacokinetics. More so, pharmacodynamic studies have shown wide variations between systemic concentrations and plasma inhibitory activity of various TKIs, likely owing to unique protein binding affinities of specific drugs. Pharmacodynamic-directed dose escalation clinical studies have mitigated these limitations for the most part and resulted in improved efficacy in clearing not only circulating blasts, but also most bone marrow disease. Recent work proposes the existence of unique niches in the bone marrow, some of which are true biochemical sanctuaries where local drug levels may be significantly different from systemic plasma drug levels. To this end, bone marrow mesenchymal stroma expresses similar levels of drug metabolizing enzymes compared with hepatocytes. They are able to metabolize CYP3A4 substrates, creating potential biochemical spaces where FLT3 inhibitors achieve levels inadequate for potent inhibition of FLT3-ITD.
Emergence of resistance
Initially, survival in the setting of TKI therapy probably happens in remote and unique niches within the bone marrow, and thus relies on the presence of a minute population of leukemia stem cells. The emergence of clinical resistance is most likely preceded by cell intrinsic events that allow the new clone to leave the “nest” and dominate the organism. Accumulating knowledge from studying the emergence of resistance to imatinib in chronic myelogenous leukemia points toward two types of resistance: (a) mutation in the target receptor or (b) activation of alternative pathways that bypass the mutant receptor.
Mutations in the Target Receptor
A variety of FLT3 mutations that could confer resistance to FLT3 inhibitors have been predicted based on in vitro models of resistance. Some of these predicted mutations have been confirmed in patients relapsing with FLT3 mutated disease during treatment with FLT3 inhibitors.
Most FLT3 inhibitors are active against FLT3-ITD, but have limited activity against TKD mutants. Even though a TKD mutation may have only minimal prognostic value when present at diagnosis, the appearance of point mutations in the FLT3 receptor is a major mechanism of resistance to FLT3 inhibitors (see Fig. 1 ). These point mutant can develop either in cis or in trans and newer FLT3 inhibitors have various degrees of activity against individual mutants.
It is important to recognize that, depending on the domain used to bind the receptor, FLT3 inhibitors can be segregated in two classes: type I inhibitors, like CEP-701, PKC-412, and crenolanib, bind to the “gatekeeper” domain adjacent to the activation loop or the ATP-binding domain; type II inhibitors, like sorafenib, quizartinib, and MLN518, directly bind the ATP-binding domain. As expected, point mutants conferring resistance to one TKI show cross-resistance within the class. To this end, patients with FLT3-ITD that relapse while treated with quizartinib, if they are found to have point mutations in the activation loop (most frequent D835) or “gatekeeping” domain (ie, F691), usually show resistance to sorafenib, another type II TKI. Interestingly, these cells remain sensitive to type I TKIs such as PKC412 and crenolanib. Similarly, some TKIs, like the type I inhibitor TTT-3002, demonstrate preclinical potential to target both type of mutations.
Activation of Alternative Signaling Pathways
Although intensively studied, the accumulation of additional mutations in the FLT3 receptor represents a minority of cases developing resistance to FLT3 inhibitors. In a small study following 60 patients with FLT3-ITD alone treated with single-agent TKI, two-thirds of patients progressed on FLT3 inhibitor treatment even though they showed no additional mutations in FLT3 wild-type allele or FLT3-ITD. Only 22% of patients acquired additional mutations, all of them D835 or I836. Thus, alternative mechanisms of resistance, independent of FLT3 receptor, must be playing a major role and recent studies have uncovered some of these pathways.
Generally, these pathways either provide survival signals independent of FLT3-ITD or they change the transcriptional factor network of the leukemic cell to a state where FLT3 signaling can be replaced by activation of other RTKs.
As mentioned, FLT3-ITD can activate signaling cascades downstream of JAK/STAT, PI3K/AKT, and MAPK pathways. Because blasts become addicted to this constitutively active signaling, FLT3 inhibitors induce rapid apoptosis. Although microenvironmental factors may rescue these cells in the stem cell niche (see above), the development of cell intrinsic mechanisms that can protect these cells from apoptosis coincide with development of resistance to TKIs. FLT3-ITD changes the balance between antiapoptotic proteins, such as Bcl2/Bcl XL , and proapoptotic Bcl-2-associated death promoter (BAD) ( Fig. 1 ). Sustained activation of phospho-STAT5 by FLT3-ITD signaling, for instance, activates Pim kinases that, in turn, by phosphorylating BAD, sequesters these proteins in the cytoplasm and allows antiapoptotic activities of Bcl2 and Bcl XL . Inhibition of FLT3-ITD results in rapid loss of phospho-STAT5 and downregulation of Pim-1. Cells resistant to FLT3 inhibitors show sustained activity of Pim-1 or Pim-2 and high levels of phospho-BAD and, thus, protection from apoptosis. Thus, combined inhibitions of FLT3-ITD and Pim1 or Pim-2 are synergistic in inducing apoptosis in mutant blasts. Similarly, high levels of Bcl2 can also confer resistance to FLT3 inhibitors. In these settings, the use of Bcl2 inhibitors such as ABT-737 rescues FLT3 inhibitor–induced apoptosis of mutated cells. Interestingly, FLT3-ITD/TKD mutants that show sustained activation of phospho-STAT5 also exhibit elevated levels of antiapoptotic signals mediated by Bcl XL . In these models, inhibition of the mammalian target of rapamycin (mTOR) pathway can rescue the sensitivity of these cells to both FLT3 inhibitors and anthracyclines. Similarly, cells resistant to sorafenib continue to have an active mTOR/PI3K/Akt pathway even in the presence of effective FLT3 inhibition, and mTOR inhibitors can resensitize the blasts to TKI. Some FLT3-ITD point mutations (D627 E) can induce expression of Mcl-1 (a Bcl-2 family member) independent of kinase activity via a conformational change that favors Grb-2 docking. Because Mcl-1, in addition to its antiapoptotic roles, also impacts mitochondrial morphology and function, it is not surprising that sorafenib resistant cells adopt an abnormal mitochondrial respiratory chain and rely mostly on glycolysis for their energy demands. Thus, glycolytic inhibitors like 2-deoxyglucose can resensitize cells to sorafenib. Of note, a major limitation to a predominantly glycolytic metabolism is a sustained decrease in intracellular pH. Consistent with this concept, FLT3-ITD cells developing resistance to sorafenib also upregulated tescalcin, a type I Na/H exchange channel. Downregulation of this protein or inhibition via amiloride reduced leukemia initiation in xenograft models of sorafenib-resistant FLT3-ITD AML.
Maintaining an active MAPK/ERK pathway either by expression of constitutively Axl-1 or acquiring activating mutations in NRAS has also been shown to be potential mechanisms of resistance to FLT3 inhibitors.
Epigenetic events, particularly methylation of target genes, have been proposed as potential mechanisms of resistance to FLT3 inhibitors. To this end, methylation of SHP-1 and silencing of SOCS proteins both negative regulators of JAK/STAT pathway have been implicated in resistance to FLT3-inhibitors. Treatment with DNMT inhibitors not only rescues expression of these proteins but also resensitizes the cells to the TKI.
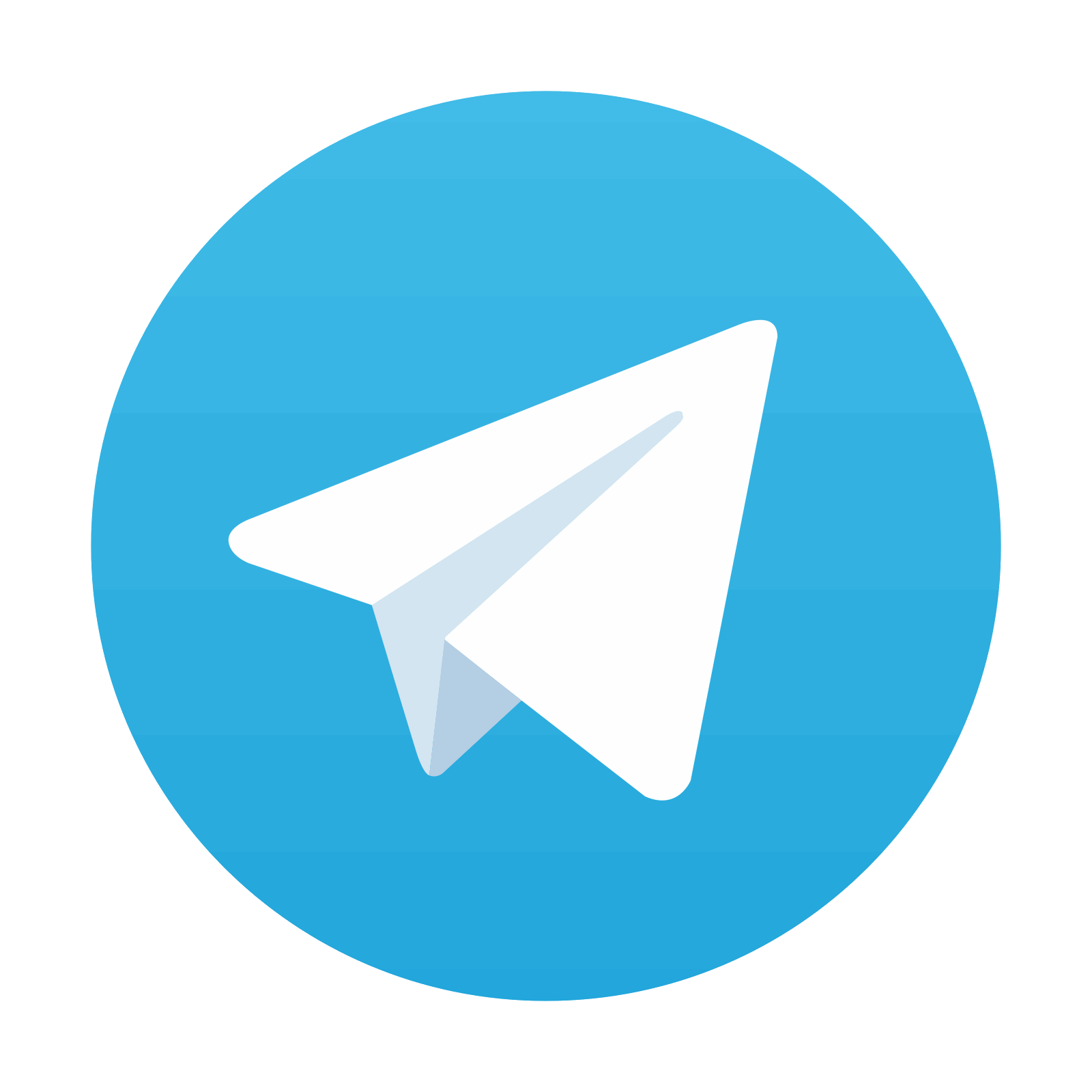
Stay updated, free articles. Join our Telegram channel

Full access? Get Clinical Tree
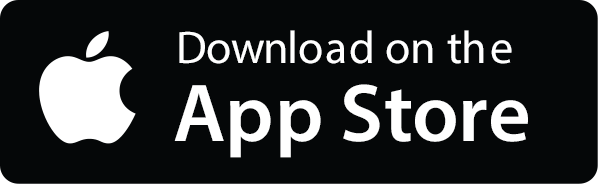
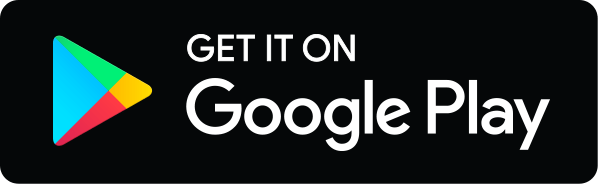