Fig. 2.1
Bone turnover is usually very high in prostate cancer (PC) patients for many reasons (aging, vitamin D deficiency, androgen deprivation therapy, or abiraterone or enzalutamide, cytokines released from primary cancer and for metastatic cancer cells activating the bone microenvironment). High bone turnover increases the rate of bone loss and impairs bone quality, increasing the risk of fragility fractures. Consensually, high bone turnover promotes the homing of circulating cancer cells and the promotion of the so-called osteoblastic premetastatic niche. A clinically evident bone metastasis (BMT) develops and grows due to the effect of growth factors released from bone matrix breakdown. The increase in size of the BMT into a frail bone finally increases the risk of an SRE. In a patient with PC bone disease, all these steps are present at the same time as a continuum in the skeleton. ADT androgen deprivation therapy, CTIBL cancer treatment-induced bone loss, SRE skeletal related event (fracture, pain, cord compression, orthopaedic surgery, radiotherapy), yellow cell osteoclast, red cell osteoblast, blue cell PC cell
Breakdown products of type I collagen by osteolysis as cross-linked collagen peptides (the amino (N)- and carboxy (C)-terminal cross-linked telopeptide of type I collagen, NTX and CTX, respectively), and the terminal peptides that are cleaved from procollagen before its integration into new bone matrix (e.g. procollagen type I N-terminal and C-terminal peptides, or P1NP and P1CP), can provide meaningful insights into the ongoing effects of tumour growth on bone turnover (Fig. 2.2). Bone-specific alkaline phosphatase (bone ALP) concentrations in serum reflect the ongoing rate of osteogenesis [2]. The International Osteoporosis Foundation and the International Federation of Clinical Chemistry and Laboratory Medicine recommend that a marker of bone formation (serum procollagen type I N propeptide) and a marker of bone resorption (serum C-terminal telopeptide of type I collagen) be used as reference analytes for bone turnover markers in clinical studies [2] (Fig. 2.3). Nowadays, a number of bone markers can be determined using enzyme immunological procedures (enzyme-linked immunosorbant assay) by means of a commercial kit that can be easily adapted to laboratory automated machines to achieve greater analytical reliability during determination compared with manual methods [3]. Although a great deal of the data in the literature are obtained on markers analysed from urine samples (i.e. NTX), analysis of makers of bone turnover on serum and plasma are recommended because of lower inter- and intraindividual variability [2]. Standardised assays are available for many bone turnover markers and normal or reference ranges for several markers have been established. As the normal range changes with age and sex, selection of appropriate reference values is critical for data interpretation [2, 4]. In systemic metabolic bone diseases, such as osteoporosis, primary hyperparathyroidism and osteomalacia, biochemical markers reflect ongoing rates of bone resorption and formation in the body as a whole. Therefore, bone marker assessments in “focal” diseases, such as Paget disease or BMT, do not provide information specific to individual lesion sites. Moreover, changes in bone marker levels are tissue specific (bone) and not disease specific and are associated with an imbalance in skeletal metabolism independently of the underlying cause [1, 5]. In cancer patients, bone turnover markers may be very high for many concomitant causes, such as age, vitamin D deficiency, adjuvant hormone therapy and BMT, but it is impossible to distinguish the contributions of the different components that elevate the marker levels in the serum and urine (Figs. 2.1 and 2.3). For example, NTX levels were similar in PC patients on androgen deprivation therapy with and without BMT. Furthermore differences between bone resorption markers and bone formation markers were not found in patients with BMT from different cancers [6, 7]. In an attempt to differentiate the source of the marker (BMT vs non-metastatic skeletal), the non-isomerised form of CTX and type I collagen breakdown products generated by matrix metalloprotease (ICTP), apparently more specific for BMT breakdown, have been evaluated (Fig. 2.2) [8].
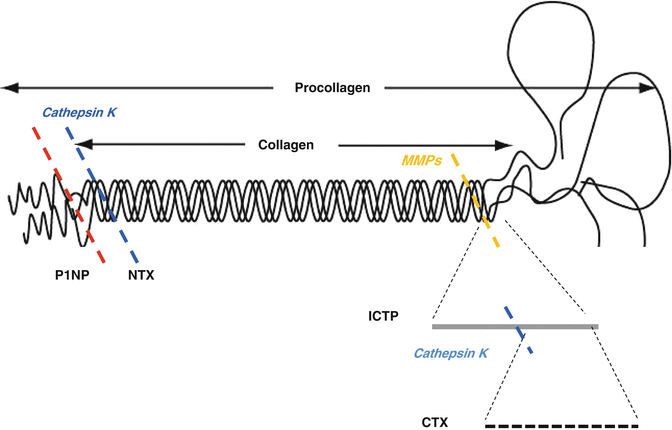
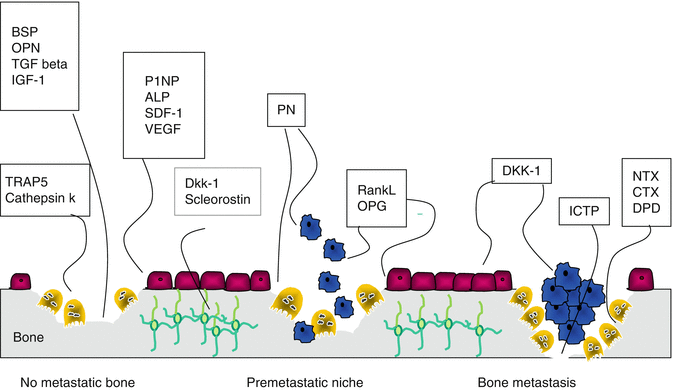
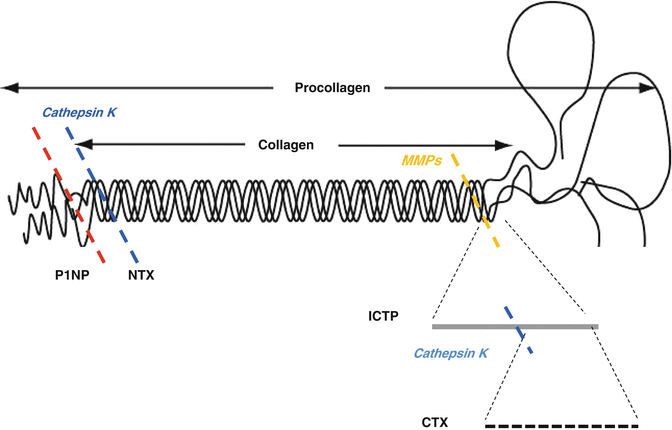
Fig. 2.2
Schematic representation of the amino-terminal propeptide procollagen type 1 (P1NP), N-terminal cross-linking telopeptide collagen type 1 (NTX), and C-terminal cross-linking telopeptide collagen type 1 (CTX). P1NP epitope is used as a marker of bone formation. NTX, CTX and ICTP epitopes are used as markers of bone resorption on type I collagen. CTX epitope is constituted by an eight-amino acid sequence on the C-telopeptide of a1. The cross-linked carboxy-terminal telopeptide collagen type 1 (ICTP) epitope is a larger conformational epitope including at least two telopeptides and the first phenylalanine of the phenylalanine-rich region. It is a product of metalloprotease breakdown of collagen type 1. As shown in the figure, cathepsin K degrades the ICTP epitope whereas it generates CTX
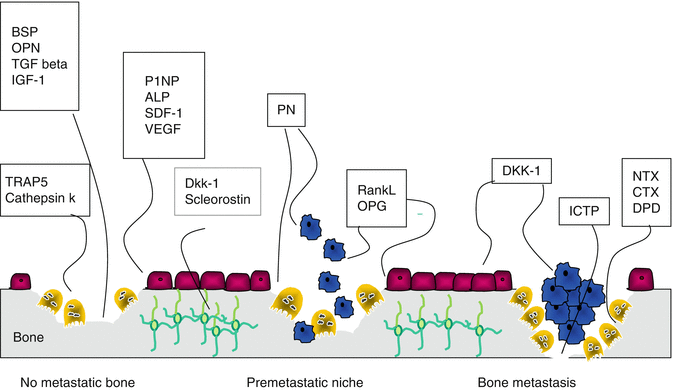
Fig. 2.3
Schematic representation of the origin of peptides used as markers of bone formation and reabsorption of bone by osteoblasts, osteoclasts, osteocytes and cancer cells. NTX and CTX derived from bone breakdown by osteoclasts through cathepsin K, ICTP mainly by cancer cell bone breakdown through metalloproteases. Cathepsin K and TRAP5 are expressed from osteoclasts during their osteolytic activity. Periostin (PN) is stored by osteoblasts during bone formation, but released during osteoclast activity. It is also expressed directly from cancer cells. P1NP and alkaline phosphatase (ALP) expressed from osteoblasts are used as markers of bone formation. Osteoblasts also express vascular endothelial growth factor (VEGF) and chemokine (SDF-1), RANKL and osteoprotegerin (OPG). DKK-1 and sclerostin are expressed by osteocytes, osteoblasts and PC cells. Bone sialoprotein (BSP), osteopontin (OPN), transforming growth factor-1 (TGF-β) and insulin-like growth factor-1 (IGF-1) are released from bone matrix during osteoclastic (and cancer) bone reabsorption (see text for details)
The clinical utility of bone markers as diagnostic indicators of bone metastatic disease and as prognostic indicators has been extensively examined. Several studies have revealed an association between bone turnover marker and the presence or progression of skeletal metastases from prostate cancer (PC) [9, 11, 12]. In these studies the formation marker and resorption markers are elevated in the case of typical osteoblastic osseous metastasis expressing a disrupted balance between bone formation and resorption. P1NP, bone sialoprotein (BSP), and osteoprotegerin (OPG) showed more significant differences between PC patients with and without BMT. BSP is not a typical bone marker and works as a general tumour marker [13]. In addition to being elevated in PC patients with BMT, OPG correlates with the extent of osseous metastasis. Furthermore, in association with RANKL, it may predict recurrence after radical prostatectomy [14–16]. P1NP as bone ALP correlates with the extent of osseous changes (bone scan index). Bone ALP had the highest diagnosis accuracy (72 % sensitivity, 88 % specificity) and P1NP the greatest diagnostic specificity (92 %) [11]. Recently, the elevated alkaline velocity was found to be an independent predictor of OS and BMT-free survival in patients with CRPC [17]. On the other hand, recent data do not confirm the diagnostic performance of P1NP. Current consensus is that the diagnostic sensitivity and/or specificity of bone markers for the presence of BMT or the prognostic role of bone lesion progression are not sufficient to utilise the results to diagnose BMT [18]. The mean values of the areas under the ROC curve from several studies were 0.81, 0.80 and 0.77 for P1NP, bone ALP and ICTP suggesting that these markers might have diagnostic values in interaction with safely defined reference thresholds or during their course [5, 10, 19]. Data on the use of bone markers as predictors for SRE and survival are quite encouraging [18, 20]. Retrospective analyses of data from phase III trials of zoledronic acid in patients with CRPC and BMT showed that both baseline and on-study elevation in bone marker levels, specifically NTX, were associated with increased risks of SRE, disease progression and death [18, 21–23]. A high baseline level of urinary NTX (above 180 nmol/mmol creatinine) was associated with a more than 2.5-fold increase in the risk of death (RR 2.58, 95 % CI 1.92–3.47) compared with low baseline levels of NTX (<55 nmol/mmol creatinine) [18, 22]. Also, baseline bone ALP was associated with a 4 % increase in the risk of death and SRE per 200 IU/L increase. Elevated bone ALP levels at baseline were associated with a shorter time to the first on-study SRE and a shorter time to the first pathological fracture. The cumulative incidence of SRE over a 1-year period was nearly doubled (50.7 % vs 26.5 %) among patients with elevated versus normal baseline bone ALP [23]. Adequate suppression of NTX and bone ALP levels during treatment (zoledronic acid plus docetaxel vs docetaxel alone) was associated with longer survival time, and similar results have been confirmed by others [23, 24]. Recently, bone ALP velocity (>6.3 IU/L/year) was found to be an independent predictor of overall survival in CRPC. A fivefold increase in death was observed among CRPC patients with rapid bone ALP velocity (OR 5.11, 95 % CI 2.24–11.67) [17]. Other more recent bone markers have been found to be associated with prognosis in PC patients. P1NP and ICTP were associated with survival after 15 months of zoledronic acid therapy [25]. Baseline and 3 months after, zoledronic acid P1NP and CTX predict survival and (only P1NP) risk of SRE [26]. The association of CTX or NTX with P1NP confirmed the prognostic role of these bone markers [27].
The data summarised above suggest that bone turnover markers might be useful to optimise the use of bone-targeted therapy for metastatic bone disease. Promoting lifelong therapy contradictory with the paucity of data regarding the usefulness and the safety (osteonecrosis of jaw and atypical hip fractures) of treatment durations with bone-modifying agents beyond 2—3 years. The serial measurement of BTMs could be a strategy in tailoring the therapy regimen and could help the decision on the optimal duration of antiresorptive therapy, which could allow treatment frequency to be reduced and even theoretically removed for periods in the context of optimal bone metabolism control [28]. In summary, at present, the potential for the clinical use of markers of bone turnover for diagnosis, prognosis and monitoring therapy in cancer patients with BMT remains unfulfilled and the routine use of these markers cannot yet be recommended. As stated in consensus publications, there is a need for harmonisation, standardisation and common reference ranges [18, 29, 30] although a recent position paper solicited their introduction into a clinical setting [28].
2.1 New Bone Markers
2.1.1 ICTP
ICTP, which reflects non-osteoclastic bone resorption mediated by metalloproteases (MMPs), is liberated to the bloodstream during pathological conditions. Serum ICTP is relatively insensitive to changes in bone remodelling mediated by normal osteoclastic activity.
In a retrospective analysis of four bone markers (NTX-l, ICTP, total ALP, and TRAP5b) in breast cancer patients with and without BMTs, only ICTP and TRAP5b were significantly higher in those patients with BMTs compared with those without (visceral metastases or no metastases). The ICTP and TRAP5b levels were also related to the number of BMTs on the other hand [31]. Furthermore in another study in breast cancer patients comparing a cohort with and without BMTs, ICTP was the marker with higher sensitivity (65 %), and it had similar specificity to bone ALP (91 vs 92 % for bone ALP) [32].
In a prospective cohort study, three bone markers (NTX-l, ICTP, and bone ALP) were tested in 123 patients with various metastatic cancers, 26 of which were extraosseous only (45 bone-only and 52 bone plus visceral). NTX-l and ICTP, but not bone ALP, were associated with bone disease progression. Moreover, NTX-l had the highest sensitivity (70 %), specificity (80 %), positive (72 %), and negative (79 %) predictive values for bone disease progression in the set of markers analysed (for an increase X30% from baseline). Curiously, when assessing ICTP, not only did it increase in the context of bone and extraskeletal progression, but it also did not decrease with bisphosphonate (BP) therapy [9]. This led the authors to speculate that ICTP could represent a bone collagen product derived from an osteoclast-independent mechanism of bone degradation (MMP-1 action on bone collagen) and therefore not influenced by BP therapy (Fig. 2.3).
2.2 Periostin
Periostin is a highly conserved matricellular protein that shares close homology with the insect cell adhesion molecule fasciclin 1. Periostin is expressed in a broad range of tissues, including the skeleton, where it serves both as a structural molecule of the bone matrix and as a signalling molecule through integrin receptors and Wnt-beta-catenin pathways, stimulating osteoblast function and bone formation. The development of periostin-null mice has allowed the crucial role of periostin in dentinogenesis and osteogenesis to be elucidated, in addition to the skeletal response to mechanical loading and parathyroid hormone. Periostin binding to the integrins activates the Akt/PKB- and FAK-mediated signalling pathways, leading to increased cell survival, angiogenesis, invasion, metastasis, and, importantly, epithelial–mesenchymal transition of carcinoma cells [33]. In situ RNA hybridisation in biopsies of breast cancer metastases showed that the periostin gene was highly expressed in the stromal cells immediately surrounding the tumour but not within the breast cancer cells themselves [34]. Although periostin is highly expressed in various types of human cancers, its function is still unclear. In mice the administration of PN1-Ab, a neutralising antibody of periostin, significantly inhibited the growth of primary tumours and metastatic tumours, associated with the prevention of bone destruction, resulting in increased survival of mice. In addition, in vitro, PN1-Ab significantly inhibited the proliferation, migration, and invasion of 4T1 mouse breast cancer cells, which produced periostin [35]. Nude mice were inoculated with human MDA-B02 breast cancer cells. Mouse-derived periostin was markedly overexpressed (eightfold) in metastatic legs compared with non-inoculated mice. Serum periostin levels were also markedly increased in metastatic mice and correlated with in situ expression levels. Immunostaining showed that periostin is derived from the surrounding stromal cells of BMT. It was suggested that periostin might be a biochemical marker of the early stromal response associated with breast cancer BMT formation [36]. The use of circulating periostin as a potential clinical biomarker has been explored in different non-skeletal conditions. These include cancers and, more specifically in the metastasis process, respiratory diseases such as asthma, kidney failure, renal injury, and cardiac infarction. A study including breast cancer and small cell lung cancer patients showed that serum periostin levels were elevated in breast cancer patients presenting with BMTs compared with similar breast cancer patients with no evidence of BMT. No correlation was found between the serum periostin level and any other prognostic factors, such as clinical stage and lymph node metastasis in breast cancer [37]. In postmenopausal osteoporosis, serum levels have been shown to predict the risk of fracture—more specifically non-vertebral—independently of bone mineral density. Because of its preferential localisation in cortical bone and periosteal tissue, it may be speculated that serum periostin might be a marker of cortical bone metabolism, although additional studies are clearly needed (Fig. 2.3) [36].
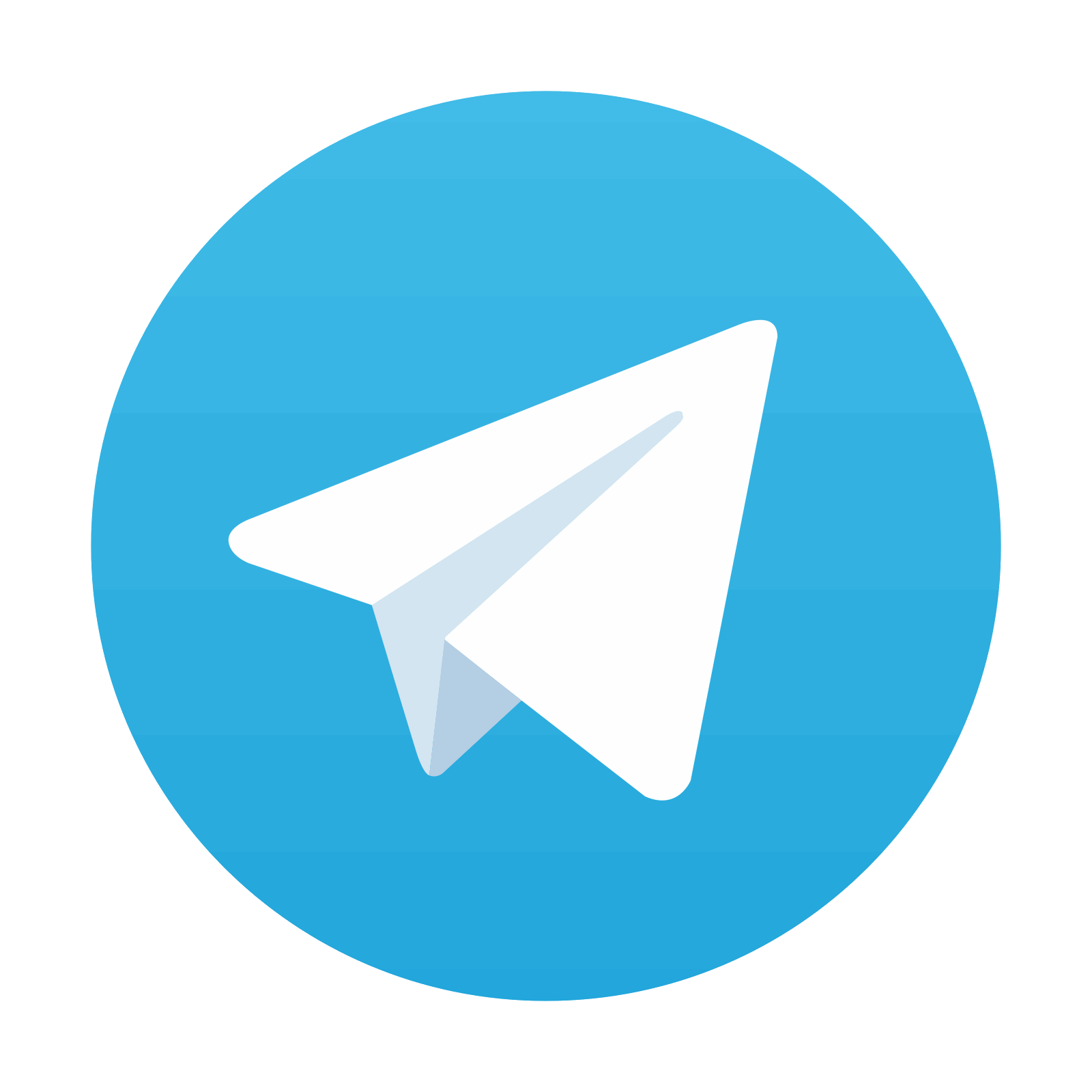
Stay updated, free articles. Join our Telegram channel

Full access? Get Clinical Tree
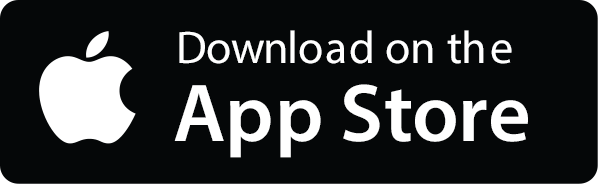
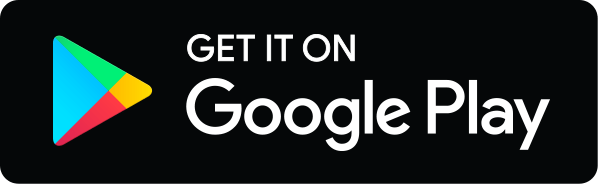