Established risk factors
Possible risk factors
Suspected risk factors
Age
Early age at menarche
Hormonal replacement therapy
Family history – genetics
Late age at menopause
Dietary fat
Nulliparity
Infertility
Obesity
Oral contraceptive use (protective)
Lactation (protective)
Sedentary lifestyle
Oophorectomy (protective)
Tubal ligation (protective)
Cigarette smoking
Hysterectomy (protective)
Alcohol consumption
Low vegetable intake
Furthermore, approximately 10 % of ovarian cancers may be due to an inherited genetic predisposition, since familial predisposition to cancer has been recognized for many years [2].
Other, rarer hereditary gene mutations are associated with increased risk of breast cancer, such as Li–Fraumeni syndrome, Cowden syndrome, Peutz–Jeghers syndrome and hereditary diffuse gastric cancer syndrome [3].
The History of Hereditary Ovarian Cancer (HOC) Discovery
The discovery 15 years ago of some of the genes that are involved in the heritance of very common malignant diseases, like breast and ovarian cancers, has represented a substantial improvement in the understanding of hereditary ovarian cancer and a challenge for cancer prevention [3].
Over the last four decades, Henry Lynch has collected pedigrees and samples from high-risk breast and/or ovarian cancer families, showing autosomal-dominant inheritance patterns for breast cancer in the late 1960s; Henry Lynch was the first to hypothesize forward a probable genetic etiologic association between carcinoma of the breast and ovary [5].
These families allowed the discovery of a genetic association between breast and ovarian cancer predisposition, contributing to the mapping of the BRCA1 and BRCA2 genes. They advanced the idea of the existence of other breast cancer susceptibility genes, allowing the evaluation of BRCA-associated cancer risks and psychosocial aspects of BRCA testing and so on [1–4].
In 1990, Mary-Claire King followed in demonstrating the first convincing linkage data in early onset breast cancer families which mapped the susceptibility gene, subsequently named BRCA1 for breast cancer 1 [5].
A particular aspect of this collection was the predominance of families with both breast and ovarian cancers and broad availability of biological samples [6].
The two genes involved in breast and ovarian cancer, called BRCA1 and BRCA2, belong to the group of tumour-suppressor genes, are transmitted in an autosomal-dominant way and encode proteins that are involved in DNA repair pathways [7].
So far, hundreds of different mutations have been reported in BRCA1 and BRCA2, with deleterious frameshift and missense mutations from variants of unknown functional significance and polymorphisms [8].
Epidemiology of Ovarian Cancer and of BRCA Mutations in Hereditary Ovarian Cancer
A large amount of epidemiologic research focusing on hereditary ovarian cancers (HOC) target on epithelial ovarian cancers because they are the predominant subtype; the highest age-adjusted incidence rates are detected in developed parts of the world, including North America and Western and Northern Europe, with rates in these areas exceeding 10 per 100,000 [9].
Practically, all benign and malignant ovarian tumours derive from one of three cell types: epithelial cells, stromal cells and germ cells; in developed countries, more than 90 % of malignant ovarian tumours are epithelial in origin, 5–6 % of tumours constitute sex cord-stromal tumours and 2–3 % are germ cell tumours [10, 11].
The incidence of ovarian cancer increases with age, with a median age at diagnosis of 63 years; more than 80 % of ovarian cancers occur after age 45 years [12].
One of the most considerable risk factors for ovarian cancer is a family history of the disease: it is estimated that approximately 7 % of women with ovarian cancer have a positive family history of this disease. The first-degree relatives of ovarian cancer probands have a threefold to sevenfold increased risk, particularly if multiple relatives are affected and at an early age at onset [13].
Three clinical manifestations of hereditary ovarian cancer have been identified: site-specific ovarian cancer, hereditary breast and/or ovarian cancer (HBOC) mostly due to BRCA1 and BRCA2 gene mutations and Lynch syndrome, formerly known as hereditary nonpolyposis colorectal cancer (HNPCC) syndrome.
On these data, a subset of ovarian cancers occurs as part of a hereditary cancer syndrome that is inherited in an autosomal-dominant pattern. The majority of hereditary ovarian cancers can be attributed to mutations in the BRCA1 and BRCA2 genes, located on chromosomes 17 and 13, respectively [14].
The prevalence of BRCA mutations in the general population is assessed to be approximately 0.1 %. Generally, mutations in highly penetrant genes account for 10–15 % of epithelial ovarian cancers; in various populations over the world, founder mutations have been found [13, 14].
Women with a BRCA1 mutation have an 85 % risk of developing breast cancer, a 60 % risk of ovarian cancer by age 70 and an increased risk of colon cancer. Those with mutations of the BRCA2 gene have a lower cancer risk, but, in addition to being a causal gene of familial breast and ovarian cancer, the BRCA2 gene is also involved in Fanconi anaemia, subtype B and familial pancreatic cancer [15].
In every case, it is important to identify population founder mutations and other recurrent mutations in each geographical area because they simplify genetic testing and facilitates counselling due to a better knowledge of the risk associated to mutation. The reported frequency of BRCA mutations in high-risk HOC families is approximately 50 % [15].
On the other hand, when unselected families are tested in the context of clinical practice, the frequency of BRCA mutations is lower, ranging between 15 and 30 % depending on selection criteria and diagnostic methods [8].
Genomic of BRCA1 Genes
The BRCA1 (BReast CAncer1) gene is located on 17q21 and has a total length of about 100 kb. It was isolated in 1994 by Wiseman, Skolnick and their co-workers.
This gene consists of 24 exons, and the coding region starts at the middle of exon 2; the size of the molecular RNA (mRNA) is 7.8 kb, and BRCA1 expression depends on the cell cycle and increases during the G1 to S phase.
The gene product of BRCA1 is a nuclear phosphorylated protein that consists of 1,863 amino acids with a molecular weight of 220 kDa; the protein is phosphorylated in a cell cycle-dependent manner, it undergoes hyperphosphorylation during the S phase and is dephosphorylated after the M phase [16, 17]. These observations suggest a role of BRCA1 in cell cycle regulation as experimentally demonstrated in vivo and in vitro [18].
A RING-finger domain is present in the N-terminal region; two BRCA1 C-terminal (BRCT) domains, also found in cell cycle checkpoint DNA repair proteins, are present in the C-terminal region. BRCT domain was found to interact with phosphorylated peptides with a role in cell cycle control [19, 20].
BRCA1 is believed to contribute to the maintenance of chromosomal stability, since BRCA1-deficient cultured cells show decreased cell growth and hypersensitivity to ionizing radiation, resulting in DNA damage associated with chromosomal abnormalities such as breaks and aneuploidy [21].
Genomic of BRCA2 Genes
The BRCA2 gene is located on 13q12.3; it has a total length of 70 kb and was isolated in 1995. It consists of 27 exons with a translational start sites in exon 2. The size of the transcription product is approximately 11.5 kb.
The expression of BRCA2 is high in thymus and testis and relatively high in mammary gland and ovary. The expression of BRCA2 is cell cycle dependent.
BRCA2 is a nuclear protein that has a molecular weight of 384 kDa; it consists of 3,418 amino acids with eight repetitive units (BRC motifs).
The sequence of BRCA2 protein shares no homology with that of BRCA1 protein; however, the BRCA2 gene is similar to the BRCA1 gene in terms of structure and expression, suggesting for both genes a common role in the control of cell proliferation and differentiation [25].
BRCA2-deficient cell lines are hypersensitive to drugs causing DNA double-strand breaks and to DNA cross-linking agents; these cell lines are considered to have abnormalities in the DNA repair of double-strand breaks.
BRCA2 binds to the DNA repair protein Rad51 at the BRC repeat regions and contributes to the maintenance of chromosomal stability and to homologous recombination [26].
Another function of BRCA2 is transcriptional regulation; Marmorstein et al. demonstrated that BRCA2 interacts with p53 in vivo and inhibits its transcriptional activity [27].
The Role of Genetics in Hereditary Ovarian Cancer
For ovarian cancer it is estimated that approximately 10 % of cases seem to be related to germline BRCA1 and BRCA2 genes; the risk of cancer related to BRCA germline mutations is variable and it derives on the type of mutation and also on the population where the risk has been studied.
The risk of ovarian cancer is slightly higher for BRCA1 than for BRCA2, of 40 and of 25 %, respectively [28].
Families with clear autosomal-dominant inheritance of ovarian cancer, but without apparent excess of breast cancer are defined at risk for HOC [12].
Three distinct clinical patterns of cancer have been noted in the families of women with ovarian cancer [14, 15]:
Ovarian cancer in association with breast cancer
Ovarian cancer alone
Ovarian cancer in association with cancers of the colon, rectum, endometrium, stomach, urothelium and pancreas, the HNPCC syndrome or “Lynch II” syndrome.
The identification of the genes that predispose to ovarian cancer has gone a long way towards explaining the patterns of cancers seen in families. Nevertheless, various tumours are related with BRCA mutations: colorectal cancer in BRCA1 mutation carriers (relative risk of 4) and prostate cancer in both BRCA1 and BRCA2 mutation carriers (relative risk 3). Other types of tumours are mainly associated to BRCA2 mutation: male breast cancer, melanoma, laryngeal and pancreatic tumours [28, 29].
The ovarian cancers in families with BRCA mutations are mainly serous adenocarcinomas, often showing papillary changes. Epithelial ovarian adenocarcinomas that occur in a hereditary setting due to transmitted germline BRCA1 mutations are predominantly of the serious type, with under presentation of mucinous and border line tumours [10, 11].
When prevention measures are to be discussed with patients or otherwise healthy women, authors have to consider the individual risk and take special care with new BRCA mutations discovered in families with a very low number of breast cancer cases [30].
Basing on the expensive costs of genetic testing, who also creates expectations in the families, when BRCA testing is planned, a prior calculation of probability of finding a mutation should be done [20].
Full sequencing is considered the standard technique for diagnosis, in view that in about 15 % of these families, rearrangements have been described. Direct sequencing is currently the main technique used for sequence analysis. A set of primers is designed for the amplification by polymerase chain reaction (PCR) of the DNA extracted from lymphocytes or cancer tissues; the complete nucleotide sequence is then performed on a DNA auto sequencer [32].
Multiple Genes Involved in Ovarian Cancer
Ovarian cancer shows a high mortality rate because it is often only diagnosed when it is in an advanced stage. Great effort is to identify screening and diagnostic strategies that improve the likelihood of early diagnosis. In the last few years, there has been an improvement of the knowledge base relating to the molecular biology of ovarian cancer.
Genes such as BRCA1, BRCA2, TP53, ATM, SKT11, PTEN, PTCH, MLH1, MSH2, KRAS/BRAF, HER2/neu and many others have been associated with increased risk of breast and ovarian cancer as part of other cancer syndromes [33].
Upregulation or downregulation of these genes can be determined on the basis of a statistically significant increase or decrease in level of the biomarker.
The morphologic and molecular genetic data support different pathways of development for each of the major histological types of ovarian carcinoma [34]:
1.
Low- and high-grade serous carcinoma most probably arise via different pathways, the former progressing along an adenoma–borderline tumour–carcinoma sequence involving mutations in KRAS and BRAF and the latter from alterations in surface epithelial inclusion glands involving mutations of TP53 and dysfunction of BRCA1 and/or BRCA2.
2.
Mucinous carcinomas most probably arise via an adenoma–borderline tumour–carcinoma sequence with mutations of KRAS.
3.
Endometrioid carcinomas arise from endometriosis with mutations of CTNNB1 (the gene encoding b-catenin) and PTEN.
4.
The genetic alterations in clear cell carcinoma are the least investigated but also support an origin from endometriosis.
The Role of Epigenetics and of Cancer Genetic Counselling Units
Mutations in BRCA1 and BRCA2 genes explain the greatest part of hereditary breast and ovarian cancers; epigenetic events, such as methylation of the promoter region, but not mutations, are considered to cause inactivation of BRCA1. Abnormalities of BRCA1/2, caused by epigenetic influencing factors, can often be implicated in carcinogenesis not only in hereditary breast and ovarian cancer but also in its sporadic forms [32].
There are several models that calculate the risk of cancer based on personal conditions or familial history; one of these is indicated in Table 7.2.
Table 7.2
The common indications to test potential patients with suspicion of hereditary ovarian cancer (HOC)
A family with two or more ovarian cancer, at least two between first-degree relatives |
Two first-degree relatives affected by breast cancer and ovarian cancer, respectively |
Breast cancer and ovarian cancer in the same patient |
Testing for genes related to hereditary cancer allows the selection of individuals who will really benefit of prevention measures in such high prevalent diseases. The majority of these genes belong to the category of tumour-suppressor genes, so a second genetic hit is always needed for cancer developing [15].
Epigenetics, described as the factors that modulate the penetrance of a genetic disease, play an important role in hereditary cancer predisposition. Due to the complexity of genetics and clinical management of the hereditary cancers, all individuals with risk should be seen at specialized group of researchers, so-called Cancer Genetic Counselling Units: the clinicians that work in these units should have special training in genetic counselling, cancer genetics and hereditary cancer management [35].
Cancer genetic counselling is a communication process in which genetics, medical and psychological questions related to hereditary cancer risk development and prevention options are discussed with the individuals and families [36].
A collaborative model between counsellor and patient is preferred, and all the following points should be included in the informed consent process: options for risk estimation if genetic testing is not done, information on the specific test (accuracy, limitations), interpretation of all possible test results, implications of results for the individual and family, confidentiality issues, risk of psychological distress, options for care after testing and limitations [36].
Some ethical and legal issues arise in the global processes of cancer genetic counselling that must be recognized and taken in account. Several questions still do not have answers in this field [35–37].
Indications for testing for BRCA mutations should be based, in every case, on the prevalence in the own population, previous reported frequencies in unselected families from the same geographical and also in cost-effectiveness criteria. Nonetheless, it would be inappropriate to dismiss the potential benefits of genetic counselling as a reaction to sporadic uncritical application of genetic testing [38].
Genetic Tests in High-Risk Subject for Hereditary Ovarian Cancer
The recent progress of research into the molecular basis of cancer in general and hereditary cancer particularly has provided more insight into the aetiology of hereditary cancer; regarding the methods of genetic risk calculation, there are several models that can be used to do that [39].
Mutation analysis of the BRCA genes should always be performed through the Regional Clinical Genetics departments, after appropriate counselling about the implications of a positive result for the individual and their family [38].
Diagnostic genetic testing can be offered in the following circumstances:
The pedigree falls into the high-risk category.
A living, affected member is available to give a blood sample for analysis.
A computer program, called BRCAPRO, is available to test positive women to risk assessment primarily based on the family history of ovarian cancers.
It applies Bayesian analysis to calculate the probability of mutation carrier for a given individual based on family history (number of breast or ovarian cancers, or age at diagnosis of cancer, presence of bilateral or male cancer and Ashkenazi origin) [40].
Since a mutation cannot be always found, the false-negative rate of the test should be considered. A large variety of methods is currently available for the detection of mutations [41].
As stated before, there is no one technique that is ideally suited to a complete analysis of BRCA1 and/or BRCA2. Some techniques are simple to perform but not very sensitive, whereas others may be very sensitive but laborious and, therefore, usually expensive [42].
The most commonly used techniques include:
Direct (semiautomated) sequencing (DS); this is generally considered the gold standard for mutation detection because of its high sensitivity, but the disadvantages are the time consuming and laborious procedures involved, although the availability of semiautomated, fluorescent sequencing systems has increased the feasibility of this method for large-scale (clinical) use.
Allele-specific oligonucleotide analysis (ASO).
Single-strand conformation polymorphism analysis (SSCP/SSCA) and heteroduplex analysis (HA); both techniques are easy to perform and relatively quick. Compared to DS, the sensitivity is much lower at a reputed 60–80 %.
Conformational sensitive gel electrophoresis (CSGE); this method has an increased sensitivity compared to HA and SSCP, but is more labour intensive.
(Constant) denaturing gradient gel electrophoresis (DGGE/CDGE); this technique, which is based on the melting behaviour of the DNA double helix, is more sensitive than SSCP; however, the technique only detects differences between both alleles; therefore, additional techniques are required to identify the precise nature of the mutation. Another disadvantage of all techniques mentioned thus far is that it may be difficult to distinguish between benign polymorphisms and pathogenic mutations.
The protein truncation test (PTT) overcomes the previous problem by detecting nonsense and frameshift mutations that result in a stop codon by visualizing a truncated protein in an in vitro transcription–translation assay.
Southern analysis (for genomic deletions); recently, specific founder mutations have been identified that consist of the loss of large fragments of coding sequence. Such genomic alterations can be detected by Southern analysis in specific populations, which have a high-expected frequency of such alterations [39–42].
Diagnostic genetic testing is still far from straightforward and the reasons for this are the following [43]:
There are more than hundreds of different mutations within the BRCA genes and each family will have its own mutation.
It is still not possible to look through the whole of these genes with current technology; therefore, the mutation cannot be found in every family whose cancer is linked to a BRCA gene.
Testing is time consuming and expensive.
Even in research laboratories undertaking extensive screening, mutations are found in less than 50 % of families with two or more women with ovarian cancer [40–43].
Genetic testing offers the following benefits: identification of high-risk individuals who will benefit from the initiation of recommended cancer risk management; identification of noncarriers in families with a known mutation, who do not need to have rigorous cancer screening and would be considered at “general population risk”; education in early detection and prevention strategies; and perhaps relief of anxiety through increasing the understanding of medical options. However, it also has its risks and limitations, including its inability to detect all mutations, the continued risk of sporadic cancer, the unproven efficacy of some interventions and the possibility of psychosocial or economic harm [44].
The goal of genetic counselling is to ensure that women have been sufficiently educated regarding inherited breast/ovarian cancer to make informed decisions concerning genetic testing and available preventive and treatment options. The majority of published studies have found that genetic counselling is effective in increasing knowledge and awareness of cancer risk and of the consequences of genetic testing [45–47].
Pedigree Information in Family with Hereditary Ovarian Cancer
As extensive, a family tree as possible should be taken including all second-degree relatives (grandparents, aunts and uncles) and third-degree relatives (cousins). It is important to take the history from the paternal side, as well as the maternal side as the genes involved are equally likely to be inherited from the paternal side of the family [48].
High-risk families are those which fulfil the following criteria [30]:
The family contains two or more individuals with ovarian cancer at any age who are first-degree relatives.
The family contains one individual with ovarian cancer at any age and one individual with breast cancer diagnosed under 50 years who are first-degree relatives.
The family contains one individual with ovarian cancer at any age and two individuals with breast cancer diagnosed under 60 years who are connected by first-degree relationships.
The family contains an affected individual with one of the known ovarian cancer predisposing genes.
The family contains three individuals with colorectal cancer with at least one case diagnosed before 50 years and one case of ovarian cancer connected by first-degree relationships.
There are problems associated with using pedigree information to assess risk.
Many families nowadays are not large enough to reach the above criteria, and families with a preponderance of males may also not reach the criteria, despite carrying an ovarian cancer susceptibility gene. However, it still remains the most useful tool for assessing risk [49]. Armel et al. evaluated the demographics of the nonresponder population to assess genetic risk estimates for mutations in the BRCA1 and BRCA2 genes and genetic testing eligibility as compared to a responder population of patients who completed a mailed family history questionnaire. A total of 430 pedigrees were obtained, 215 from nonresponders and 215 from responders. Results of this study indicate that 69 % of nonresponders were either unreachable by telephone (42 %), declined an appointment (19 %) or were previously seen in another centre for a genetic counselling visit (8 %). Additionally, results indicate that nonresponders are less likely to be eligible for genetic testing (40 %) as compared to responders (57 %) (p = 0.0004). Together these data shed light on a population of patients for which limited information exists and suggest that we question how and to what extent clinics should pursue nonresponders, particularly in light of global reductions in health care funding [50].
The Risk Management of Hereditary Ovarian Cancer
An accurate estimation of the risk of ovarian cancer is important in counselling these women, since important decisions have to be made during a crucial phase of life, the childbearing years. Risk assessment and counselling are based on penetrance estimates of ovarian cancer at certain ages, which have been investigated by many studies worldwide [51]. Unfortunately, those penetrance studies show varying results, due to the choice of the population studied and the cancer incidence. Estimates of the average penetrance up to 70 years of age are 40 % (95 % CI, 35–46 %) for BRCA1 and 18 % (95 % CI, 13–23 %) for BRCA2 mutations carriers [52].
Mortality for ovarian cancer is generally high because women typically present with late-stage disease, when the overall 5-year relative survival rate is 45 % [53].
Despite success in pinpointing precursor lesions in cervical and uterine malignancies, little progress has been made in identifying premalignant conditions in the ovary: they rest in ultrasonography and in cancer antigen 125 (CA-125) serum level and in the majority of ovarian cancers; no transition area from normal to dysplastic to malignant epithelium can be identified [54].
Difficulties involved in monitoring the ovaries in high-risk subjects are complicated: biopsy is hampered by the ovaries’ location deep in the pelvis and imaging; via ultrasound and computed tomography is helpful for determining ovarian size and morphology, but cannot distinguish more subtle lesions such as ovarian dysplasia or inclusion cysts [55].
Even in high-risk populations, traditional approaches to screening such as pelvic exam, ultrasound and CA-125 are hampered by their poor ability to detect early stage disease and to distinguish benign from malignant neoplasm [56].
Genetic consultation and testing are currently mainstream components of a multidisciplinary, individualized medical evaluation aimed at identification of individuals at risk for hereditary ovarian cancer syndromes [44].
Various options for risk management of hereditary ovarian cancer are available: surveillance, prophylactic surgeries and chemoprevention [57].
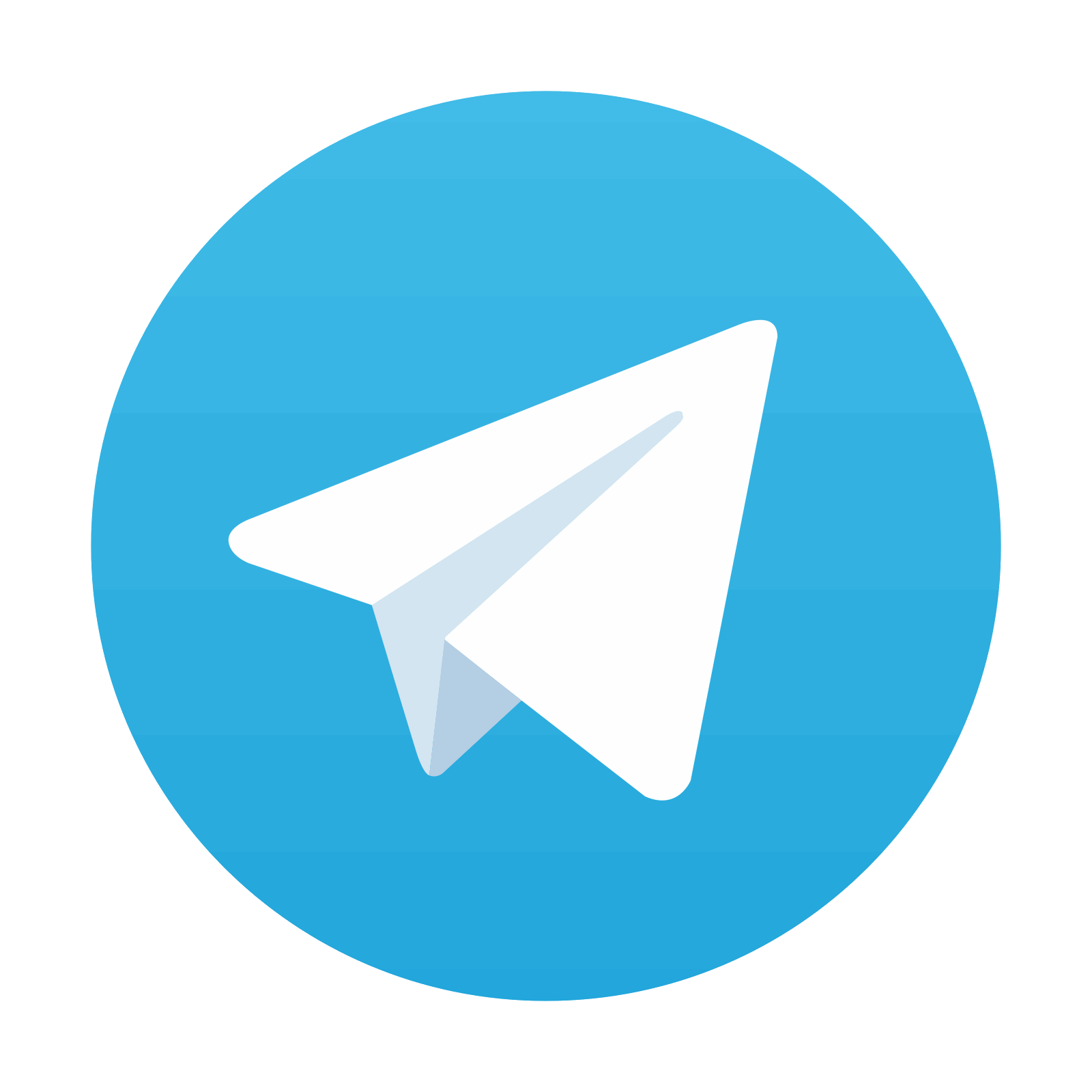
Stay updated, free articles. Join our Telegram channel

Full access? Get Clinical Tree
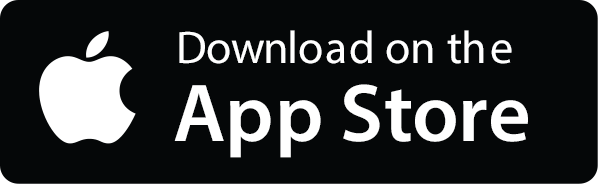
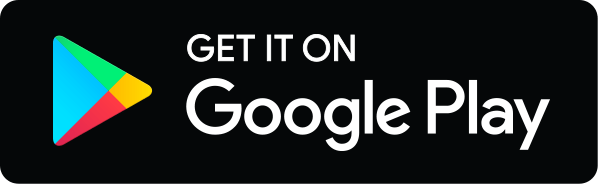