Management of Advanced Lung Disease Including Pleural and Pericardial Effusions
John C. Ruckdeschel
Darroch W.O. Moores
One of the most common, troubling problems for the patient with cancer is the development of a malignant effusion in any body cavity. When it occurs in the pleural cavity or the pericardium, this abnormal accumulation of fluid may cause severe symptoms or may even be life threatening. Although the development of a malignant pleural or pericardial effusion almost always indicates that the patient has incurable cancer, prompt recognition and diagnosis followed up by appropriate treatment can result in excellent palliation and a marked improvement in the patient’s quality of life. In addition, it is critical that an accurate diagnosis is made to establish the malignant cause of the effusion, because patients with cancer can often develop an effusion from a benign cause such as radiation therapy, infection, chemotherapy, or heart failure.
Pleural Effusions
Incidence
In a general hospital patient population, 28–61% of all pleural effusions are malignant; the highest incidence is in the over-50 age-group (1, 2, 3, 4). A malignant pleural effusion is the initial manifestation of cancer in 10–50% of patients (4, 5). Eventually, about half of all patients with disseminated cancer develop a malignant pleural effusion (4). Overall, this is a highly significant clinical problem, resulting in approximately 100,000 malignant pleural effusions annually in the United States (6). Table 28.1 lists the frequency of the various tumor types that are associated with malignant pleural effusions. The tumor origin is not found in 15% of effusions. In such cases, the cell type is usually metastatic adenocarcinoma with the primary site unknown.
Table 28.1 Tumor Causes of Malignant Pleural Effusions From Collected Series | ||||||||||||||||||||||
---|---|---|---|---|---|---|---|---|---|---|---|---|---|---|---|---|---|---|---|---|---|---|
|
The appearance of a symptomatic, malignant, pleural effusion in a patient with known cancer significantly alters his or her quality of life. Although it is reported that up to 25% of patients with an effusion are asymptomatic, this may prove to be an overestimate if a careful history is elicited (7). In general, a prompt diagnosis accompanied by timely treatment of the effusion can markedly enhance the patient’s functional status and should be considered early after the development of this common complication of advanced cancer.
Etiology and Pathophysiology
The pleura is a serous membrane that invests each lung to form a closed sac called the pleural cavity. There is a continuous passage of an almost protein-free fluid (protein content, 1.5 g per dL) through the pleural membrane that is attributable primarily to hydrostatic and colloid osmotic pressures. The net pressure in the parietal pleura is 9 cm H2O, favoring movement of fluid into the pleural cavity, which is balanced with a net 10 cm H2O pressure in the visceral pleura, favoring absorption of pleural fluid by the visceral capillaries. Therefore, the overall direction of movement of fluid is from the systemic circulation in the parietal pleura across the pleural cavity back into the pulmonary circulation in the visceral pleura. At any point of time, either pleural cavity has only 2–5 mL of fluid present, although as much as 5–10 L flow through this space in any 24-hour period (8, 9). Any imbalance in these pressures that disturbs the normal equilibrium may lead to a net accumulation of fluid in the pleural cavity.
Traditionally, it has been held that the normal equilibrium may be interrupted, leading to the accumulation of an effusion. Some of the changes that may occur include the following:
Increased capillary permeability due to inflammation from infection or tumor cell implantation
Increased oncotic pressure of fluid in the pleural space due to inflammatory reaction from tumor cells or infection
Decreased systemic oncotic pressure due to hypoalbuminemia from malnutrition
Increased negative intrapleural pressure due to atelectasis, possibly because of tumor obstructing a bronchus
Increased hydrostatic pressure in the pulmonary circulation, such as in congestive heart failure from cardiac or pericardial metastases
In addition, a malignant effusion may result from obstruction of the visceral or parietal lymphatic channels by tumor or radiation fibrosis (or a combination thereof), resulting in impaired absorption. Most malignant effusions are probably the result of a combination of factors, with an overall increase in fluid production and a decrease in absorption (3, 10, 11).
More recently, the role of vascular hyperpermeability as mediated by vascular endothelial growth factor (VEGF) has
received increasing attention. Fidler’s group at M. D. Anderson has demonstrated that pleural invasion by malignant cells expressing VEGF messenger ribonucleic acid (mRNA) is required for production of malignant effusions and that the effusions (but not tumor growth) could be abrogated by transfection of the cells with an antisense VEGF (12). PTK 787, a compound that blocks VEGF receptor tyrosine kinase phosphorylation (among other receptor tyrosine kinases), leads to reduced pleural fluid formation but not to reduced proliferation of the tumor cells (13). Several investigators have described increased levels of VEGF in the fluid of patients with malignant effusions (14, 15, 16, 17, 18, 19) and the presence of increased FLT-1 VEGF receptors (fms-like tyrosine kinase receptor of VEGF) on mesothelial cells (15). A Dutch group has demonstrated that antibodies against VEGF or SU5416, a small molecule tyrosine kinase inhibitor, can also block production of both pleural and peritoneal fluid accumulation in vitro (20). Some studies have shown that VEGF levels can distinguish benign and malignant effusions (14, 15, 16) but there is some overlap seen (18). Although still in an early phase of understanding, the role of vascular hyperpermeability may explain why some agents that do not induce brisk fibrosis in the pleural space may still be useful in the treatment of malignant effusions (21).
received increasing attention. Fidler’s group at M. D. Anderson has demonstrated that pleural invasion by malignant cells expressing VEGF messenger ribonucleic acid (mRNA) is required for production of malignant effusions and that the effusions (but not tumor growth) could be abrogated by transfection of the cells with an antisense VEGF (12). PTK 787, a compound that blocks VEGF receptor tyrosine kinase phosphorylation (among other receptor tyrosine kinases), leads to reduced pleural fluid formation but not to reduced proliferation of the tumor cells (13). Several investigators have described increased levels of VEGF in the fluid of patients with malignant effusions (14, 15, 16, 17, 18, 19) and the presence of increased FLT-1 VEGF receptors (fms-like tyrosine kinase receptor of VEGF) on mesothelial cells (15). A Dutch group has demonstrated that antibodies against VEGF or SU5416, a small molecule tyrosine kinase inhibitor, can also block production of both pleural and peritoneal fluid accumulation in vitro (20). Some studies have shown that VEGF levels can distinguish benign and malignant effusions (14, 15, 16) but there is some overlap seen (18). Although still in an early phase of understanding, the role of vascular hyperpermeability may explain why some agents that do not induce brisk fibrosis in the pleural space may still be useful in the treatment of malignant effusions (21).
Pleural effusions caused by malignancy usually result in an exudate with a high-protein content, although rare exceptions have been reported (1, 5). However, transudative effusions (low protein) may occur as the indirect result of an advanced cancer, as in the patient with malnutrition and hypoalbuminemia, congestive heart failure due to cardiac failure, or liver disease secondary to metastases (3).
Clinical Presentation
The most critical initial step in evaluating the patient with a suspected malignant pleural effusion is to take a complete history and perform a careful physical examination. This simple step will usually exclude other causes of an effusion, such as heart failure or infection.
The clinical presentation of a malignant pleural effusion is almost always related to collapse of lung from the increased pleural fluid and the resulting initial symptom of exertional dyspnea. Later, resting dyspnea and orthopnea develop as the effusion increases in volume. A dry, nonproductive cough, a sense of heaviness in the chest, and occasionally pleuritic chest pain are also experienced. Nevertheless, an occasional patient (< 25%) will appear completely asymptomatic in the face of a significant effusion (11).
The physical findings of a malignant pleural effusion often include dullness to percussion of the affected hemithorax, decreased vocal fremitus, decreased breath sounds, egophony, and no demonstrable diaphragmatic excursion. Rarely, a very large effusion will result in a mediastinal shift with contralateral tracheal deviation and possibly even plethora or cyanosis from partial caval obstruction (3, 7). Other signs and symptoms may be present initially but are usually related to the underlying primary tumor and not the effusion.
Diagnosis
The approach to the diagnosis and subsequent treatment of a patient with a suspected malignant pleural effusion is shown in Figure 28.1.
The initial screening consists of the posterior–anterior chest radiograph, including decubitus views, which will confirm the presence of free pleural fluid and will also suggest the presence of any loculated fluid. An upright posterior–anterior chest radiograph that demonstrates blunting of the costophrenic angle will detect 175–500 mL of fluid, whereas a decubitus view will show as little as 100 mL (22).
Computed tomography (CT) scan may play a role in the evaluation of patients with malignant pleural effusion. This is especially necessary if the hemithorax is opaque on chest radiograph, when a mesothelioma is suspected, or when the underlying primary tumor is unknown. However, a large effusion often obscures an underlying tumor in the lung, and CT scan may prove more useful after drainage of the effusion and lung re-expansion. Occasionally, chest ultrasonography may prove useful in differentiating between pleural fluid and pleural thickening (23), but its more common application is to localize small effusions to guide in thoracentesis (24).
After confirmation of a pleural effusion radiographically and after exclusion of any obviously nonmalignant causes, the next step is a diagnostic thoracentesis. In general, when a malignant effusion is suspected, only a small amount of fluid is withdrawn for diagnosis (at least 250 mL is needed for cytology). If the patient is symptomatic, enough fluid should be removed, slowly, to relieve symptoms but the chest should not be drained dry. Leaving a moderate amount behind makes insertion of a chest drainage tube easier.
The use of a disposable thoracentesis kit with a specially designed multihole catheter is preferred, because it tends to lessen the chance of complications such as a pneumothorax. Rapid removal of a large amount of fluid, especially over 1500 mL, is not advised, because this may result in life-threatening re-expansion pulmonary edema, a real and not infrequent complication of large-volume thoracenteses (25).
The fluid removed should be heparinized and sent to the lab for protein and lactic acid dehydrogenase (LDH) determinations and cytology. Other tests, including pH determination (for which the fluid must be collected in an anaerobic container), glucose level, cell count, and cultures with smears, are often obtained, although they are not necessary. With a malignant pleural effusion, the pH and glucose level are often low, but these are nonspecific findings (3). A malignant effusion is frequently hemorrhagic (erythrocyte count > 100,000 per mm3), but this is also nonspecific and occurs in only one third of cases (5). The Health and Public Policy Committee of the American College of Physicians has demonstrated that elevated levels of LDH or protein in the fluid and ratios of fluid to concurrent serum levels of LDH and protein are all that are required for the distinction of a transudate from an exudate (Table 28.2) (26). If the fluid is found to be a transudate, then malignancy is essentially excluded. An exudate
with a negative cytology result demands a second diagnostic thoracentesis with cytology, which will add approximately 6–11% more malignant diagnoses (3).
with a negative cytology result demands a second diagnostic thoracentesis with cytology, which will add approximately 6–11% more malignant diagnoses (3).
Table 28.2 Diagnostic Tests Confirming the Presence of an Exudate | ||||||||||||||||
---|---|---|---|---|---|---|---|---|---|---|---|---|---|---|---|---|
|
Cytology is the best method of diagnosing a malignant pleural effusion, although it is somewhat dependent upon tumor type, the experience of the cytopathologist, and the amount of fluid that is sent for cytologic analysis; at least 250 mL is preferred for the best yield (27). In patients with an effusion eventually proved to be malignant, the first cytology result will be positive in 53–59% of cases, rising to 65% when a second thoracentesis is performed (3). In some tumors, such as Hodgkin’s disease, the rate of positive cytology results is relatively low at 23%, while with others, such as breast or lung cancer, the diagnosis rate is as high as 73% (3). A variety of immunocytochemical staining techniques and, recently, cytogenetic markers have been added to complement the standard cytologic techniques. These methods have resulted in some increased diagnostic yield, although they often lack sensitivity and specificity, and also the laboratory expense is increased (3, 10). We do not recommend using these tests outside of a clinical trial.
If the result of cytologic examination was negative, traditionally a blind pleural biopsy has been attempted, with some expectation of increased diagnostic yield. Although a blind biopsy may be a sensitive test in pleural tuberculosis because of its diffuse involvement, malignancies involving the pleura are commonly patchy in distribution, and a blind pleural biopsy usually adds little to the diagnosis. Although earlier series suggest that the pleural biopsy may be valuable in diagnosing malignancy in a patient with negative cytology results (3), a more recent direct comparison of pleural fluid cytology versus blind pleural biopsy casts doubt on its benefit. In this important series (28), cytology results were diagnostic of cancer in 71% of cases and suggestive in another 8%. Blind biopsy of the pleura gave positive results for malignancy in 45% of cases but provided a diagnosis in only 3% of cases where the cytology result was nondiagnostic.
Therefore, if two exudative pleural fluid cytology results are negative for malignancy, most clinicians now bypass the blind pleural biopsy and move promptly to video-assisted thoracoscopy (VATS), wherein visually directed pleural biopsies are readily obtained and the diagnostic yield is quite high. In large series, the diagnostic sensitivity of VATS with malignant pleural effusion is 97% (29). Although VATS is a surgical procedure usually requiring general anesthesia, the mortality and morbidity is quite low at 0.5% and 4.7%, respectively (30).
In addition to providing a high diagnostic yield of malignancy by directed biopsies during VATS, this procedure, when the ipsilateral lung is collapsed and the patient is under general anesthesia, permits direct definitive therapeutic intervention with lysis of adhesions, mechanical pleurodesis, pleurectomy,
or talc poudrage, with a very low recurrence rate (31). Finally, when the fluid cytology result is positive but the primary site is unknown, VATS may be employed occasionally to obtain a larger amount of tissue for more definitive studies or to rule out the presence of a mesothelioma, especially when “adenocarcinoma, unknown primary” is the working diagnosis according to cytology. The advent of VATS has virtually eliminated the need for diagnostic open thoracotomy when a malignant effusion is suspected.
or talc poudrage, with a very low recurrence rate (31). Finally, when the fluid cytology result is positive but the primary site is unknown, VATS may be employed occasionally to obtain a larger amount of tissue for more definitive studies or to rule out the presence of a mesothelioma, especially when “adenocarcinoma, unknown primary” is the working diagnosis according to cytology. The advent of VATS has virtually eliminated the need for diagnostic open thoracotomy when a malignant effusion is suspected.
Prognosis
Once the diagnosis of a malignant pleural effusion has been made, the choice of therapy must be put in perspective with respect to the extent of the tumor, the condition of the host, and the prognosis. Virtually all of these patients have an incurable disease, so the treatment must be aimed at the most effective palliation for maximal, comfortable time outside of the hospital. For all patients, the overall mean survival time is 3–6 months. The mortality is as high as 54% at 1 month and rises to 84% at 6 months (11, 32, 33). Some malignant effusion patients with responsive tumors, such as breast cancer, may have a longer survival averaging 7–15 months (11); 20% have a 3-year survival (32). Ovarian cancer patients with a malignant effusion also have a longer expected mean survival of 9 months (23). Conversely, patients with lung cancer have a worse prognosis, with a mean survival of 2 months, and 66% will die by 3 months (11).
Treatment
Systemic Therapy
When the effusion is small and asymptomatic and the tumor is likely to be sensitive to systemic therapy, as with lymphoma, leukemia, breast cancer, ovarian cancer, small cell lung cancer, or germ cell tumors, the first line of therapy should be systemic chemotherapy or hormonal therapy, preceded by a “therapeutic” thoracentesis if the patient is symptomatic (7, 10). When the tumor is relatively chemoresistant or has been shown to be so in the past, as with non–small cell lung cancer or pancreatic cancer, the choice then is for prompt tube thoracostomy followed up by intrapleural therapy if the patient has a reasonable life expectancy. In instances where the patient’s outlook and performance status are poor, simple prolonged drainage by an indwelling catheter may be more appropriate.
Thoracentesis Alone
Thoracentesis alone may relieve symptoms briefly, but the fluid usually reaccumulates rapidly. In a study of 94 patients, the mean time for reaccumulation of the pleural effusion after thoracentesis was 4.2 days, and 97% had a recurrence of the fluid by 1 month (34). In addition, repeated thoracenteses carry the risk of empyema, pneumothorax, trapped lung from inadequate drainage and loculation of fluid, and the real possibility of progressive malnutrition from repeated removal of a large amount of the high-protein effusion fluid.
Radiation Therapy
Radiation therapy may occasionally be useful as primary therapy, but this is the case only when it is directed at paramalignant effusions caused by mediastinal lymphadenopathy and lymphatic obstruction. Improvement is uncommonly seen before 3 weeks, and therefore radiation has little application in the more acute symptomatic effusion.
Tube Thoracostomy Alone
Tube thoracostomy alone has been proposed as “effective” therapy for malignant effusions (3). But review of the results demonstrates that none of these older studies reliably supports the conclusion that chest tube drainage alone is efficacious in long-term control (3). Tube thoracostomy does appear quite useful in draining the pleural cavity and maintaining opposition of the pleural surfaces when a therapeutic agent is subsequently instilled into the chest for sclerotherapy.
Chronic Indwelling Chest Catheters
Soft silastic indwelling catheters can easily be placed under local anesthesia. Once placed, the patient or caregiver drains the pleural space periodically by connecting the catheter to a suction canister to provide relief from dyspnea or symptoms of pressure. When used long term (>6 weeks), these catheters can achieve spontaneous pleurodesis between 40 and 46% of the time (35, 36, 37). This rate of pleurodesis is substantially lower than that seen with a number of sclerosing agents (see subsequent text) but the catheters can be used in the setting of loculated effusions where sclerotherapy is not attainable. Results of treatment with the silastic catheter were compared with those obtained from chest tube drainage and sclerosis with doxycycline in 148 patients with malignant pleural effusion. Overall relief of symptoms and success rate were comparable in the two groups (37). The advantage for the silastic catheter group was the shortened hospitalization: 1 day versus 6.5 days, but the major disadvantage is the requirement that the catheter must stay in place for an extended period of time and may, therefore, not be well tolerated by the patient.
Recently, some enthusiasm has been expressed for the use of small-bore (8 French or 12 French) catheters for gravity drainage or even suction drainage of the pleural cavity followed by intracavitary sclerotherapy. The long-term results of this technique are still undetermined, although the selection of patients with free-flowing effusions can enhance the outcome significantly (38). These small-bore catheters can rapidly become occluded from debris and fibrin clots, and effective suction can usually be maintained in the pleural cavity for only a few hours. If, as per the general opinion, the visceral and parietal pleurae should be kept in direct opposition for a day or two after instillation of the sclerosing agent to obtain a pleurodesis, then it is unlikely that these small catheters will be as successful as larger chest tubes.
To achieve the best palliation, the pleural cavity should be drained completely and the lung fully expanded. This is best accomplished by closed tube thoracostomy. A no. 24 French or 28 French chest tube is usually inserted in the sixth or seventh interspace in the midaxillary line with the tube directed posterior to the lung to maximize drainage. Generally, the patient is given 3–6-mg morphine sulfate intravenously just before the procedure, to reduce pain and allay anxiety. The patient is positioned supine but turned up with the appropriate side of the chest elevated at 45–60 degrees, with the physician standing behind the patient. A brief thoracentesis is always performed first at the planned pleural entrance interspace to make sure that there is free aspiration of fluid. If no fluid is obtained, the chest tube entrance site must be altered to a place where fluid is readily obtained by thoracentesis. A small 2–3-cm skin incision is made 1.0–1.5 interspaces below the target interspace, and a subcutaneous tunnel is created bluntly with a clamp. Once the tube is inserted to a predetermined depth based on numerical markings on the tube itself, it is firmly sutured into place to the skin with heavy silk suture (0-silk or larger). The tube is then attached to an underwater seal drainage device at approximately 20 cm H2O suction. If there is a very large effusion, all of it should not be drained immediately. Instead, 1000–1500 mL should be drained initially, and then the tube
should be clamped for 30–60 minutes, draining approximately 1000 mL every 60 minutes until the chest is completely empty. More rapid drainage of a large pleural effusion encourages the development of the life-threatening problem of re-expansion pulmonary edema, a real and preventable phenomenon (25). Although some physicians use a trocar chest tube, the risks of laceration of the lung or even the heart or diaphragm are significant.
should be clamped for 30–60 minutes, draining approximately 1000 mL every 60 minutes until the chest is completely empty. More rapid drainage of a large pleural effusion encourages the development of the life-threatening problem of re-expansion pulmonary edema, a real and preventable phenomenon (25). Although some physicians use a trocar chest tube, the risks of laceration of the lung or even the heart or diaphragm are significant.
Chest radiographs are obtained immediately following the procedure and then daily as long as the tube is in place. The chest tube is left on suction for total drainage of the effusion and to encourage re-expansion of all possible lung. When a properly positioned chest tube is completely open and is on suction, essentially all of the fluid that is drainable will have drained within a few hours of insertion and certainly after overnight drainage. Likewise, all portions of the lung that are expandable and are not trapped will have expanded after overnight suction. Although some authors suggest waiting an indefinite period of time until the chest tube drainage has decreased to an arbitrary 100 mL per 24 hours (10), waiting this extra time is probably not necessary and may even lessen the chance of success. The prolonged presence of the tube irritating the pleural cavity may encourage loculations and lessen the eventual distribution and effectiveness of the sclerosing agent. Therefore, the decision when to instill the intracavitary agent should rest on the appearance of the chest radiograph and not the daily drainage. Usually, the best time to instill the sclerosing agent is the day after chest tube insertion.
Fibrinolytic Agents
If the chest radiograph taken the day after chest tube insertion shows apparent residual fluid, and especially if the fluid is thick or gelatinous, it may be worthwhile to try intrapleural instillation of urokinase 100,000 units in 100 mL 0.9% saline, clamping of the tube for 6 hours, and then resumption of suction for another 24 hours (39). This technique has been reported to improve drainage and lung expansion in loculated empyemas as well as with loculated malignant effusions with no side effects or systemic effects on coagulation or fibrinolysis (39, 40).
Pleural Sclerotherapy
By far, the most common palliative treatment of malignant pleural effusions is drainage of the pleural space with re-expansion of the lung, followed by instillation of a chemical agent into the pleural cavity. It has been thought that the treatment works by causing a pleuritis designed to create a symphysis between the visceral and parietal pleurae (also called pleurodesis by sclerotherapy) to prevent reaccumulation of fluid in this space. As noted in the following text, this may not be the mechanism by which many sclerosing agents work, but rather it is their impact on production of various cytokines that impact on vascular permeability.
The most commonly used method to drain the effusion is with a chest tube, as described earlier (see the section Tube Thoracostomy). The complete drainage of the chest and full re-expansion of the lung are the major predictors of success. Another apparent determinant of the success in pleural sclerosis is the glucose level and the pH of the pleural effusion. Several authors have found that a low glucose level (< 60 mg per dL) and pH (< 7.20) in the malignant effusion result in a higher recurrence rate after attempted chemical pleurodesis as well as an overall shortened patient survival (41, 42). Although interesting, the exact significance of these isolated reports is uncertain and probably should not influence the choice of agent or techniques employed.
Controversy abounds as to which is the most effective chemical sclerosing agent for malignant pleural effusions. Comparison of the many published reports on various agents is often difficult because of the difference in reporting response rates, patient criteria, side effects, methods of evaluating results, and follow-up. The most widely followed set of guidelines to analyze results in the literature were those published by Hausheer and Yarbro (3). Many investigators have challenged whether the concept of complete and partial responses is relevant to the evaluation of pleurodesis (7). However, we believe that a simpler and more accurate means of assessing therapeutic efficacy is to follow the time to recurrence by comparing the chest radiograph taken before and after pleurodesis and removal of the chest tube. In many instances, the pleural effusion recurs or progresses, but re-treatment is not indicated because the patient has progressive disease elsewhere. For assessment of therapeutic efficacy, these cases still need to be counted as “failures.” On the other hand, whether the progression is clinically meaningful—that is, needs re-treatment—is a significant issue in cost-effectiveness studies.
Mechanism of sclerosis
The mechanism of action of sclerosing agents appears to vary somewhat depending on the type of agent and the laboratory model tested. One of the earliest studies (43) employed the rabbit as the test animal and found that the pH of the solution instilled into the pleural cavity appeared to be an important determinant of success. The most acidic solutions tested, including unbuffered tetracycline (the most acidic being pH 2.5), were quite effective in creating a small polymorphonuclear-predominant effusion and resulted in complete pleural symphysis on postmortem examination of the animals. Other agents with higher, more neutral pHs, such as nitrogen mustard and quinacrine, had no significant effect on the rabbit pleurae in this study. However, a later study by the same group (44) using the same model but adding bleomycin and sodium hydroxide to the series test agents found that the pleural sclerosing effect was actually independent of pH and was more related to the increasing dosage of tetracycline, the only agent causing a pleural sclerosis in this model.
Clinical studies in humans have shown a significant discrepancy and lack of correlation with animal studies in the efficacy of sclerosing agents such as bleomycin. It has been demonstrated that bleomycin and even nitrogen mustard are highly effective in humans (3) despite the complete lack of effect in the rabbit model. Traditionally, it has been held that the rabbit model is of little relevance because of these discrepancies. It is possible that we are examining the measure of a wrong outcome. The presence or absence of pleural fibrosis (and all of its molecular findings such as a marked rise in basic fibroblast growth factor after talc pleurodesis (21)) may in fact be an epiphenomenon. If the full role of VEGF and related compounds is better elucidated, it may well be that the balance of permeability enhancing and suppressing factors caused by variations in tumor, inflammatory response, and “sclerosing” agent is, in fact, the key determinant in measuring the outcome of various clinical interventions. The various “sclerosing” agents and laboratory measures (e.g., pH and white blood cell count) will then need to be reassessed.
Technique of pleural sclerotherapy
The most common technique used to instill the sclerosing agent into the pleural cavity has developed primarily from convention and common usage (3, 45, 46, 47) and not on the basis of rigorous studies. After complete drainage of the pleural cavity with a chest tube and re-expansion of the lung on suction, the tube is clamped and the patient is placed into the lateral decubitus position with the affected hemi thorax upward to assure that all of the sclerosing agent initially drains into the chest. Most clinicians will administer a parenteral narcotic, such as morphine 3–6 mg, intravenously 10 minutes before the procedure to minimize the potential and unpredictable pain of the chosen sclerosing agent. Approximately 150-mg lidocaine (15 mL of a 1% solution without epinephrine) is instilled initially into the pleural cavity
through the chest tube using a Luer-lock syringe and 22-gauge needle. It is allowed to remain for several minutes as local analgesia. The sclerosing agent dissolved in 50–100 mL 0.9% saline is then injected into the chest tube, and the tube is left clamped for 2 hours. The use of talc slurry requires a slightly different technique using a bulb syringe and a saline flush (48).
through the chest tube using a Luer-lock syringe and 22-gauge needle. It is allowed to remain for several minutes as local analgesia. The sclerosing agent dissolved in 50–100 mL 0.9% saline is then injected into the chest tube, and the tube is left clamped for 2 hours. The use of talc slurry requires a slightly different technique using a bulb syringe and a saline flush (48).
Usually the patient is then turned and repositioned every 15 minutes during the 2 hours to assure even distribution of the agent throughout the pleural cavity. However, when this patient repositioning maneuver was investigated carefully in a comparative clinical study, rotation of the patient during the time the chest tube was clamped offered no significant benefit to the success of the attempted pleurodesis (47). After 2 hours, the chest tube is unclamped and the tube is placed back on suction. The patient is followed up with daily chest radiographs to verify continued complete drainage of the pleural cavity. The chest tube is removed when the daily drainage drops to 100 mL or less (49) (or < 50 mL per 8 hours), and the patient is discharged the same day, once a confirmatory radiograph has been taken after removal of the chest tube. Most physicians create a moderately long subcutaneous tunnel (1.0–1.5 interspaces) through which the chest tube is initially inserted, so that with tube removal the wound seals well without the need (and discomfort) for placing sutures to close the skin wound.
Sclerosing agents
Historically, a wide variety of agents have been instilled into the pleural space to create a pleurodesis (3, 7). Table 28.3 lists most of the agents that have been described in the literature. These agents have differed greatly as to their effectiveness, side effects, availability, and even cost. Many are of only historical interest, whereas others, such as talc, bleomycin, and doxycycline, are in common use. Table 28.4 summarizes the efficacy of the most widely used sclerosing agents reported in the literature, comparing them with other methods of therapy designed to prevent the recurrence of malignant pleural effusions.
Table 28.3 Intrapleural Sclerosing Agents | ||
---|---|---|
|
Table 28.4 Malignant Pleural Effusions: Response Rates of Currently Available Therapy | |||||||||||||||||||||||||||||||||||||||||||||||||||||||||
---|---|---|---|---|---|---|---|---|---|---|---|---|---|---|---|---|---|---|---|---|---|---|---|---|---|---|---|---|---|---|---|---|---|---|---|---|---|---|---|---|---|---|---|---|---|---|---|---|---|---|---|---|---|---|---|---|---|
|
Tetracycline
As an agent for intrapleural sclerotherapy, tetracycline gained widespread acceptance and was preferred in the United States (49) and Europe (50). This agent demonstrated consistent efficacy, with a mean objective response (using the 1-g dose) of 69–85%, averaging 72% (3, 45). It proved to be safe, effective, and quite inexpensive, and could be easily administered, with adverse reactions limited to fever (7–33%) and pain (17–62%) (3, 30). Unfortunately, parenteral tetracycline is no longer commercially available.
Over the years, a variety of comparative clinical series, mostly nonrandomized, have been published with disparate methodology, patient selection, and response criteria, as well as retrospective analyses and variable results (3, 45). Despite these limitations, most older studies comparing tetracycline with other agents such as nitrogen mustard, quinacrine, mustine, doxorubicin, bleomycin, and Corynebacterium parvum consistently demonstrated that tetracycline gave superior or at least equivalent results with much less toxicity (51). Of the few recent randomized larger trials, the series of Ruckdeschel et al. (52) and Johnson and Curzon (53) comparing intrapleural bleomycin and tetracycline showed a significantly better response to pleurodesis with bleomycin, with similar toxicity profiles. Nevertheless, tetracycline’s ready availability and lower cost retained it as a preferred agent for pleurodesis of malignant effusions (49, 50).
Doxycycline
Clinical studies with doxycycline have now accumulated, after the demise of parenteral tetracycline. Initially, most were nonrandomized trials (39, 54, 55, 56). Doxycycline has consistently demonstrated effective results: response rates range from 73 to 95%, which is similar to those of bleomycin (Table 28.4). Its toxicity is similar to tetracycline and the pain on administration may be less. The 500-mg
dosage of doxycycline empirically chosen for these preliminary studies was probably too low, because over one third of patients required repeat dosing for it to be effective (39). The major problem in using doxycycline, as noted in prior studies, is that it frequently requires several dosings for equal efficacy (57), and this seriously reduces its cost effectiveness because of the need for longer hospitalizations (58). Minocycline, another substitute for tetracycline, was reported in a small seven-patient study with results similar to tetracycline, and experience with this agent has been minimal.
dosage of doxycycline empirically chosen for these preliminary studies was probably too low, because over one third of patients required repeat dosing for it to be effective (39). The major problem in using doxycycline, as noted in prior studies, is that it frequently requires several dosings for equal efficacy (57), and this seriously reduces its cost effectiveness because of the need for longer hospitalizations (58). Minocycline, another substitute for tetracycline, was reported in a small seven-patient study with results similar to tetracycline, and experience with this agent has been minimal.
Bleomycin
The most extensively studied cytotoxic chemotherapeutic agent used for intrapleural sclerotherapy is bleomycin. Not only does it play a significant role in the treatment of various solid tumors by parenteral administration, but also it has been shown to be highly effective in the palliative treatment of malignant pleural effusions, with response rates averaging 84% (3). Numerous nonrandomized studies have compared this agent with its primary competitor tetracycline, and most have suggested that there is no significant difference in efficacy or toxicity (51).
A few randomized trials of bleomycin versus tetracycline were performed, with varying results. Kessinger and Wigton (59), in their study of 41 patients, found no significant difference in the response rates using 89 units of bleomycin (67%) versus 500-mg tetracycline (61%), and both agents had similar toxicities. However, Ruckdeschel et al. (52), in their multi-institutional randomized study of 74 patients receiving either 60 units bleomycin or 1-g tetracycline intrapleurally, found the complete response rate at 1 month to be 64% (18/28) in the bleomycin arm and 33% (9/27) in the tetracycline arm. At 90 days, the complete response rate was 87% for bleomycin and 56% for tetracycline. Johnson and Curzon, in their randomized trial of 60 patients, found an 87% complete response rate at 90 days with bleomycin, compared with 56% with tetracycline (53). The acute toxicities of both drugs were similar, although there tended to be a higher incidence of pain in the tetracycline arm in both of the latter studies (52, 53).
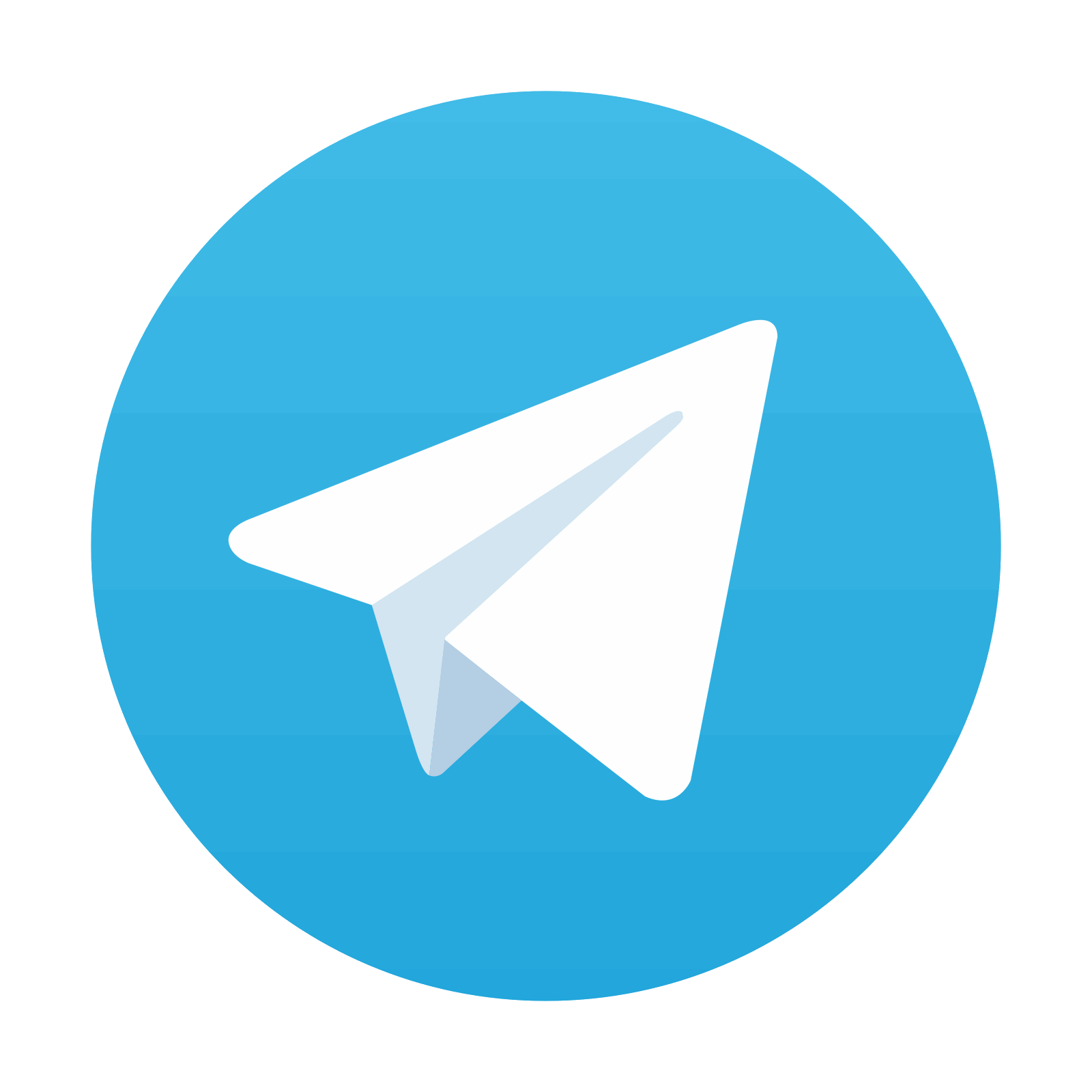
Stay updated, free articles. Join our Telegram channel

Full access? Get Clinical Tree
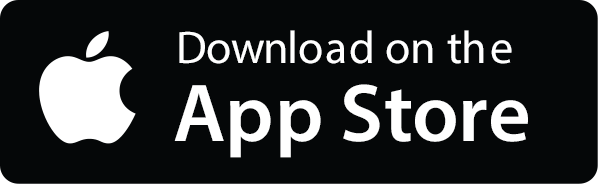
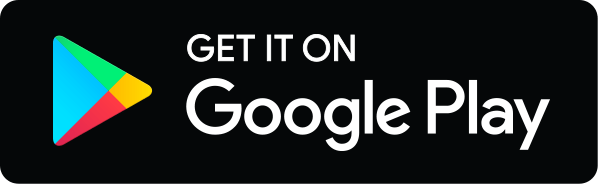
